Abstract
Hydrology and competition are major determinants of plant zonation patterns in wetlands. We conducted a pot experiment to investigate effects of water levels on plant growth of two dominant species from the Sanjiang Plain (Deyeuxia angustifolia and Carex lasiocarpa) in monoculture and mixtures (i.e., 2:1, 1:1, and 1:2 mixtures of C. lasiocarpa and D. angustifolia). Water level had significant effects on the biomass of both species (p < 0.05). The aboveground biomass of C. lasiocarpa increased with decreasing water level in both monoculture and mixtures and the same was true for belowground biomass in monoculture. Waterlogging (10 cm water level) and natural drought reduced the aboveground and belowground biomass of D. angustifolia in monoculture and waterlogging (20 and 10 cm water levels) also reduced the aboveground biomass in mixtures. The relative yield of C. lasiocarpa was greater than 1.0 in the 1:1 mixture (t = 3.49) and the 1:2 mixture (t = 6.63) at 20 cm water level (i.e., the performance of individuals was better in mixtures than that in the monoculture), and the same was true for D. angustifolia in the 2:1 mixture (t = 10.23) and the 1:1 mixture (t = 4.12) at −10 cm water level. The relative yield of C. lasiocarpa increased with increasing water level in the 1:1 and 1:2 mixtures but decreased for D. angustifolia in the 2:1 mixture. Moreover, the species with smaller initial proportion usually had higher relative yield in mixtures. This would tend to promote the coexistence of these two species. Our results suggest that hydrologic regime change has the potential to produce marked influences on plant productivity and ecosystem carbon budget in the Sanjiang Plain. Moreover, competition is an additional important determinant of plant distribution and community structure in this area.
Introduction
Hydrologic regime is a major determinant of plant community development and patterns of vegetation zonation in wetlands (Seabloom et al. Citation1998; Casanova and Brock Citation2000; Correa-Araneda et al. Citation2012). In general, hydrology exerts strong effects on both soil–atmosphere gas exchange and soil redox dynamics (Pezeshki Citation2001; Niedermeier and Robinson Citation2007), and consequently, leaf stomatal conductance, photosynthesis (Pezeshki et al. Citation1998; Li et al. Citation2004), and plant biomass production (Kennedy et al. Citation2003; Li et al. Citation2004; Garcia-Serrano et al. Citation2007; Jung et al. Citation2009). Therefore, a better understanding of the relationship between plant growth and hydrology can improve the effectiveness of wetlands management and restoration (Brock and Britton Citation1995). However, there is still much to be learned about responses of plant species to water level variation in wetland ecosystems (Kennedy et al. Citation2003).
Competition is a key factor controlling plant growth and community assembly (Gaudet and Keddy Citation1988; Keddy et al. Citation2002; Engels et al. Citation2011) that interacts with hydrology to affect vegetation zonation in freshwater marshes (Austin Citation1990; Hodgson et al. Citation1999; Engels et al. Citation2011). Lenssen et al. Citation(1999) found that species distribution and richness of freshwater shorelines was mainly controlled by hydrology in the frequently flooded zone but by plant interactions at higher elevation. Moreover, competition among plant species generally varies with water levels in freshwater marshes (Keddy and Reznicek Citation1986; Keddy et al. Citation1994; Kennedy et al. Citation2003), thus influencing the dominant plant species of these communities (Pennings et al. Citation2005). Knowledge about effects of competition on plant growth and community assembly at varying water levels is essential for evaluating factors influencing species distribution and richness (Lenssen et al. Citation1999; Kennedy et al. Citation2003; Engels et al. Citation2011).
The Sanjiang Plain, one of the largest freshwater marshes in China, is located in the Heilongjiang Province in northeast China. Like many wetland ecosystems around the world, these marshes also exhibit distinct plant zonation patterns along a water level gradient (Wang et al. Citation2010). Specifically, the high marshes (i.e., the seasonally inundated marshes and wet meadows) and low marshes (i.e., the permanently inundated marshes) are, respectively, dominated by Deyeuxia angustifolia and Carex lasiocarpa, whereas the middle marshes are co-dominated by the two species. Within the ecotones, individuals of these species would be influenced not only by hydrology, but also by species interaction (Luo et al. Citation2010). In the past decades, the marshes in this area have experienced major changes in hydrology from human activities and natural disturbances (Song et al. Citation2009). There is little information about the impacts of hydrology on the plant community in this region. In this study, we used a pot experiment to investigate the effects of water level and community composition on plant growth of D. angustifolia and C. lasiocarpa. The objectives were to (1) explore the effects of hydrologic regime on plant growth in different communities; and (2) test species’ effects on and responses to competition along water gradients.
Methods
Sites description
The study was conducted in the Sanjiang Experimental Station of Wetland Ecology, Chinese Academy of Sciences, located in the Sanjiang Plain in northeast China (47°35′ N, 133°31′ E). The study site experiences a temperate continental monsoon climate in the seasonal frozen zone. Its mean annual temperature is 2.5°C, precipitation is approximately 558 mm (more than 65% falls in July and August), and the frost-free period is 125 days. Detailed information regarding the study site was reported by Song et al. Citation(2009).
Plant materials and experimental design
C. lasiocarpa and D. angustifolia are both perennial clonal herbs (C. lasiocarpa is a sedge, while D. angustifolia is a gramineae) with shoots germinating in April and senescing in September. Ramets of C. lasiocarpa and D. angustifolia were collected in May 2011 from Sanjiang freshwater marshes. The collected seedlings (height = 27 ± 2 cm) were planted outdoors in plastic cups (13 cm height, 5 cm diameter) that contained 10 cm of marsh soil and 0.5 cm of standing water to germinate new ramets. The soil used in this study was a meadow marsh soil with pH (1:2.5 soil weight:water volume suspension) of 5.66, organic carbon of 37.1 mg g−1, total nitrogen of 3.1 mg g−1, and total phosphorus of 0.51 mg g−1, which corresponds to inceptisols in American soil taxonomy. Ten days later, ramets of similar size were transplanted to plastic pots (50 cm height, 30 cm diameter) that were filled with 20 cm of marsh soil. Then, these containers were buried in the ground outdoors to a depth of 20 cm to keep the temperature inside in equilibrium with the ground temperature.
Each species was grown in pure culture and in combination with the other. The total density of all plants was constant (i.e., 12 individual plants in each pot). C.lasiocarpa ramets were mixed with D. angustifolia ramets in five proportions (1:0, 2:1, 1:1, 2:1, and 0:1; . The monocultures (proportions of 1:0 and 0:1) were designed to simulate C. lasiocarpa and D. angustifolia monodominant communities, while the proportions of 2:1, 1:1, and 1:2 (C. lasiocarpa:D. angustifolia) were designed to simulate communities co-dominated by C. lasiocarpa and D. angustifolia. Water level treatments were chosen for each culture treatment based on the variation of water conditions that each plant community experiences in the field for a total of 20 experimental treatments (see ).
Table1. Water level treatments for each of the monocultures and species mixtures of C.lasiocarpa and D. angustifolia; the first number in the ratio refers to C. lasiocarpa and the second number refers to D. angustifolia.
Figure1. Schematic illustration of the experimental design showing one each of the four replicates of each treatment. White circles indicate C. lasiocarpa and dark circles indicate D. angustifolia. (A)and (E) are sketches of the monocultures of C. lasiocarpa and D. angustifolia, while (B), (C), and (D) are sketches of the 2:1, 1:1, and 1:2 mixtures, respectively.
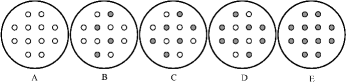
Four replicate pots of each treatment were arranged in a completely randomized design. All plants were allowed to grow for 75 days before harvest. Because the roots of C. lasiocarpa and D. angustifolia in the mixtures were closely intertwined, only the aboveground portions were harvested in the mixture as described previously (Fowler Citation1982; Sammul et al. Citation2000; Garcia-Serrano et al. Citation2007). After harvest, plants in the monoculture were divided into aboveground and belowground portions while aboveground portions in the mixture were divided into C. lasiocarpa and D. angustifolia. Then all plant portions were oven dried at 65°C for 72 h and weighed.
Although it is considered preferable to assess experiments not only in terms of biomass but also with other Darwinian fitness parameters, such as survival and reproduction (Aarssen and Keogh Citation2002), both of the study species are clonal herbs, and in such species biomass often explains most of the variation in competitive ability (Gaudet and Keddy Citation1988). Therefore, biomass is taken as a comprehensive Darwinian fitness parameter in this study.
Statistical analyses
Relative yield (RY, Fowler Citation1982) was calculated for the aboveground portions at each treatment level as follows:
where Yij is the yield for species i growing with species j, Yji is the yield for species j growing with species i, Yi is the yield for species i growing in pure culture, Yj is the yield for species j growing in pure culture, p is the initial proportion of species i in mixed culture and q the initial proportion of species j in the mixed culture, with p + q = 1.
Relative yield measures the average performance of individuals in the mixture compared to that of individuals in the monoculture. If the growth of an individual of species i is unaffected by the identity of the neighboring individuals, then RYij = 1.00; RYij > 1.0 means species i suffers less interference from the individuals of species j (interspecific competition) than it does from the individuals of its own species (intraspecific competition); RYij < 1.0 means intraspecific competition is less than interspecific competition for species i. Detailed information regarding RY was presented by Fowler Citation(1982).
In this study, species i and j were grown in several different proportions, but all at the same total density, so the results could be presented in the form of ‘ratio diagrams’ (de Wit Citation1960), in which the final proportion is plotted against the initial proportion (p or q) using a logarithmic scale on both axes. We obtained the final proportion (F) by
The initial proportion is plotted on the x-axis and the final proportion on the y-axis. Then the points are fitted with a linear equation, ln(y) = a × ln(x) + b. If one species consistently outcompetes the other, then a = 1; a < 1 means a stable equilibrium exists, a situation often described as ‘negative frequency dependent’ (Harper Citation1977; Braakhekke Citation1980) because the performance of each species increases as its initial proportion in the mixture decreases. The equilibrium point is likely to locate where x = y (i.e., where the final proportion equals the initial proportion).
All data were analyzed using the SPSS 13.0 for Windows software package (SPSS Inc. Citation2004), and the significance level was α = 0.05. Data were tested for normality using the Kolmogorov–Smirnov test. When not normal, they were log-transformed (RY = log(x + 0.5)). Relative yield from each mixture was compared to a value of 1.00 using t-tests for control conditions. One-way analysis of variance (ANOVA), followed by Tukey's or Tamhane's multiple comparison test was used to test differences in means of aboveground and belowground biomass and relative yield.
Results
Biomass
Water level treatments had a significant effect on the aboveground and belowground biomass of both species ( and ). In the monoculture of C. lasiocarpa, 0 cm water level resulted in significantly greater aboveground and belowground biomass than all other water levels ((A) and 2(C)). In mixtures, 20 cm water level always resulted in the smallest aboveground biomass of C. lasiocarpa, but the aboveground biomass at 10 cm water level was not significantly different from that at 0 cm water level ((A)). In the monoculture of D. angustifolia, both natural drought and waterlogging significantly reduced the aboveground biomass ((B)), and −10 cm water level resulted in significantly greater belowground biomass than all other water levels ((D)). In the mixtures of C. lasiocarpa and D. angustifolia, waterlogging (both 20 cm and 10 cm water level treatments) significantly reduced the aboveground biomass of D. angustifolia ((B)).
Figure2. Biomass (mean ± SE, n = 4) of the plant species grown in monoculture in the four water level treatments: (A) aboveground biomass of C. lasiocarpa; (B) aboveground biomass of D.angustifolia; (C) belowground biomass of C. lasiocarpa; (D) belowground biomass of D.angustifolia. F and p-values are from a one-way ANOVA, testing the effects of water level on biomass. Different letters represent significant differences among the water level treatments (p < 0.05).
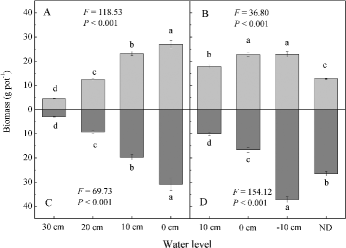
Figure3. Biomass (mean ± SE, n = 4) of the plant species grown in mixtures in four water level treatments: (A) aboveground biomass of C. lasiocarpa; (B) aboveground biomass of D. angustifolia. F and p-values are from a one-way ANOVA, testing the effects of water level on aboveground biomass. Different letters represent significant differences among water level treatments (p < 0.05).
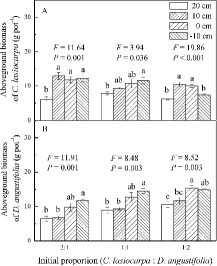
Relative yield
The relative yield of C. lasiocarpa was greater than 1.00 in the 1:1 and 1:2 mixtures at 20 cm water level (t = 3.49 and 6.63, respectively) and in the 1:2 mixture at 10 cm water level (t = 4.09). The same was true for D. angustifolia in the 2:1 and 1:1 mixtures at −10 cm water level (t = 10.23 and 4.12, respectively). Conversely, the relative yield of C. lasiocarpa was significantly smaller than 1.00 in the 2:1 mixture at 0 cm water level (t = −7.34) and in 1:1 mixture at 10 cm water level (t = −10.34). The relative yield of C.lasiocarpa increased with rising water level in 1:1 and 1:2 mixtures, but decreased for D. angustifolia in the 2:1 mixture ( and ). The relative yield of C. lasiocarpa increased with initial proportion declining at all water levels, and the same was true for D. angustifolia at −10 cm water level ( and ). The slopes of the lines fitted by regression to relative yield data were both less than 1.00 at 10 cm and 0 cm water levels (). The calculated stable equilibrium for this pair of species F was 1.72:1.00 at 10 cm water level ((A)), and 0.83:1.00 at 0 cm water level ((B)).
Table2. One-way ANOVA results of the effects of water level and initial proportion on the relative yield (RY) of C. lasiocarpa and D. angustifolia in mixtures.
Table3. Effects of initial proportions (C. lasiocarpa:D. angustifolia) and water levels on RY. Values are means (standard errors in parentheses) of n = 4. Different capital letters represent significant differences between water level treatments under the same initial proportion conditions (p < 0.05), while different lowercase letters represent significant differences between initial proportions under the same water level conditions (p < 0.05).
Figure4. Ratio diagrams of C. lasiocarpa:D. angustifolia mixtures at: (A) 10 cm water level and (B) 0 cm water level. Both slopes of the fitted linear equations differ significantly from 1.00, indicating that the rarer species has the competitive advantage and a stable equilibrium exists in the mixture at these two water levels.
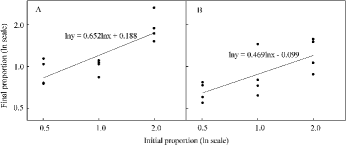
Discussion
In our study, responses of D. angustifolia biomass to hydrology regimes did not vary between the monoculture and the mixture. Similar to Luo et al. Citation(2010), we also found that waterlogging suppressed the growth of D. angustifolia. This is likely attributed to the plant's low porosity (relative volume of internal gas spaces in plant tissues), which renders D. angustifolia unable to overcome anoxic stress due to waterlogging by transporting oxygen to root parts (Armstrong and Drew Citation2002; Xie et al. Citation2008). Consistent with Luo et al. Citation(2010), we also observed that the aboveground and belowground biomass of C. lasiocarpa decreased as the water level in the monoculture increased. Moreover, C. lasiocarpa had the lowest aboveground biomass at 20 cm water level in mixtures. This demonstrates that C. lasiocarpa is a flood-tolerant species with relatively high porosity (Colmer Citation2003) that mainly establishes in permanently inundated habitats (Luo et al. Citation2008), yet long-term waterlogging still inhibits its growth. In the past decades, agricultural activity has caused water level decline and degeneration of the Sanjiang Plain marshes, and annual precipitation has experienced a decreasing trend in this area (Yan et al. Citation2001; Luan et al. Citation2007). Thus, the research results may imply that declining water levels caused by human activity and natural disturbances would increase plant productivity and thus increase carbon sequestration in plants in temperate freshwater marshes.
In this study, the non-waterlogging treatment (0 cm water level) caused an increase in C. lasiocarpa biomass in the monoculture, but generally had no significant difference relative to mild-waterlogging treatment (10 cm water level) in all mixtures. This may be because interspecific competition suppresses the plant's growth at the physiological optimum (Laird and Schamp Citation2008; Bartelheimer et al. Citation2010). Just as the relative yield of C.lasiocarpa showed in this study, the interference from D. angustifolia decreased with rising water level in 1:1 and 1:2 mixtures. Therefore, C. lasiocarpa biomass did not differ between non-waterlogging and mild-waterlogging conditions. In addition, the relative yield of D. angustifolia, more than 1.00 in 2:1 and 1:1 mixtures at −10 cm water level, means that interspecific competition is less than intraspecific competition for D.angustifolia under such conditions and the same was true for C. lasiocarpa in 1:1 and 1:2 mixtures at 20 cm water level. Our results suggest that, similar to hydrology, competition is another strong key factor controlling plant growth and distribution in the freshwater marshes, the Sanjiang Plain. It happens that there are lots of similar cases around the world. For example, Grace and Wetzel Citation(1981) and Snow and Vince Citation(1984) presented evidence that competition produced zonation patterns, by means of a controlled experiment at the W.K. Kellogg Biological Station of Michigan State University, and by means of field experiment in a subarctic marsh, respectively. In addition, Wilson and Keddy Citation(1986) found that the mean positions of species along environment gradients were related to relative competitive performance on the shore of Axe Lake, Ontario.
The slopes of the linear equations fitted by regression (<1.0 at 10 cm and 0 cm water levels) imply that both species show negative frequency dependent behavior. Similar to our research results, Fowler Citation(1982) also found that, in mixtures containing Plantago lanceolata and Poa pratensis, the species that formed a smaller proportion of the mixture had larger individuals in the grassland ecosystem. The negative frequency dependent behavior may reveal a mechanism whereby C. lasiocarpa and D. angustifolia coexist in marshes of the Sanjiang Plain and further imply the maintenance mechanism of diversity in plant community in freshwater marshes. In marshes, however, both species are also found in pure communities. One explanation is that other species have to pay a high cost to adapt to the surroundings of special habitats (Grace and Wetzel Citation1981) and could not find refuge or enough space or resources due to the intraspecific competition of dominant species. Consequently, they eventually escape. In view of the differences in the relative yield and species proportions between 0 cm and 10 cm water level, another inference could be that the plant community structure will be modified when the water level changes substantially during the growing season. Finally, the results also imply that pairwise mixture studies are not ideal for competition studies; that is, competitive hierarchies derived from pairwise (1:1) competition experiments may be questionable.
Limitations of the experiment
Although our experiment allowed us to quantify competitive abilities along a water level gradient, there were some limitations to our study. First, how representative of plants in natural communities are pot-grown plants? Plants grown in controlled conditions are often at ‘optimal’ or high resource levels (Mokany and Ash Citation2008). This may affect the intensity of interspecies and intraspecies competition among individuals. Second, short-term experiments may not extrapolate directly to longer time scales. Both species in this study are perennial herbs; hence, a one-growing-season experiment may lead to biased estimates of competitive performance (Niu et al. Citation2008). Field culture experiments (e.g., Engel and Weltzin Citation2008) and long-term experiments (e.g., Fowler Citation1995; Weiher et al. Citation1996) are needed in the future to determine how the competitive hierarchy of response changes.
Conclusions
This study examined effects of hydrology regime and competition on growth of two important dominant species (C. lasiocarpa and D. angustifolia) in freshwater marshes in China. Hydrology exerts a strong effect on the growth of C. lasiocarpa and D. angustifolia. Both waterlogging and drought reduced plant community biomass. Considering that the biomass increased with declining water level, a hydrology regime change due to human activity and natural disturbances has the potential to stimulate plant growth and increase carbon sequestration in plants in temperate freshwater marshes. The differences in effects of hydrology regimes on the aboveground biomass of C. lasiocarpa between the monoculture and the mixtures, along with the differences in the relative yield of C.lasiocarpa and D. angustifolia among mixtures, suggest that competition is an important determinant of plant distribution and community structure in marshes of the Sanjiang Plain. Negative frequency dependent behavior suggests the coexistence mechanism of C.lasiocarpa and D.angustifolia, and further implies the maintenance mechanism of diversity in the marsh plant community in the Sanjiang Plain.
Acknowledgements
This research was supported by the National Basic Research Program (973) of China (No. 2010CB951304), National Natural Science Foundation of China (No. 31100357) and Strategic Priority Research Program (No. XDA05050508). We thank the anonymous reviewers for their time and suggestions.
References
- Aarssen LW, Keogh T. 2002. Conundrums of competitive ability in plants: what to measure? Oikos. 96:531–542.
- Armstrong W, Drew MC. 2002. Root growth and metabolism under oxygen deficiency. In: Waisel Y, Eshel A, Kafkafi U, editors. Plant roots: the hidden half. 3rd ed. New York (NY): Marcel Dekker. p. 729–761.
- Austin MP. 1990. Community theory and competition in vegetation. In: Grace JB, Tilman D, editors. Perspectives on plant competition. New York (NY): Academic Press. p. 215–238.
- Bartelheimer M, Gowing D, Silvertown J. 2010. Explaining hydrological niches: the decisive role of below-ground competition in two closely related Senecio species. Journal of Ecology. 98:126–136.
- Braakhekke WG. 1980. On coexistence: a causal approach to diversity and stability in grassland vegetation. Agricultural Research Reports. 902:1–164.
- Brock MA, Britton DL. 1995. The role of seed banks in the revegetation of Australian temporary wetlands. In: Wheeler BD, Shaw SC, Fojt W, Robertson RA, editors. Restoration of temperate wetlands. Cambridge (UK): John Wiley & Sons. p. 183–188.
- Casanova MT, Brock MA. 2000. How do depth, duration and frequency of flooding influence the establishment of wetland plant communities? Plant Ecology. 147:237–250.
- Colmer TD. 2003. Long-distance transport of gases in plants: a perspective on internal aeration and radial oxygen loss from roots. Plant, Cell and Environment. 26:17–36.
- Correa-Araneda FJ, Urrutia J, Soto-Mora Y, Figueroa R, Hauenstein E. 2012. Effects of the hydroperiod on the vegetative and community structure of freshwater forested wetlands, Chile. Journal of Freshwater Ecology. 27:459–470.
- de Wit CT. 1960. On competition. Verslagen van het Landbouwkundig Onderzoek in Nederland. 66:1–82.
- Engel EC, Weltzin JF. 2008. Can community composition be predicted from pairwise species interactions? Plant Ecology. 195:77–85.
- Engels JG, Rink F, Jensen K. 2011. Stress tolerance and biotic interactions determine plant zonation patterns in estuarine marshes during seedling emergence and early establishment. Journal of Ecology. 99:277–287.
- Fowler N. 1982. Competition and coexistence in a North Carolina grassland: III. Mixtures of component species. Journal of Ecology. 70:77–92.
- Fowler N. 1995. Density-dependent demography in two grasses: a five-year study. Ecology. 76:2145–2164.
- Garcia-Serrano H, Sans FX, Escarre J. 2007. Interspecific competition between alien and native congeneric species. Acta Oecologica. 31:69–78.
- Gaudet CL, Keddy PA. 1988. A comparative approach to predicting competitive ability from plant traits. Nature 334:242–243.
- Grace JB, Wetzel RG. 1981. Habitat partitioning and competitive displacement in cattails (Typha): experimental field studies. American Naturalist. 118:463–474.
- Harper JL. 1977. Population biology of plants. London: Academic Press.
- Hodgson JG, Wilson PJ Hunt R, Grime JP, Thompson K. 1999. Allocating C-S-R plant functional types: a soft approach to a hard problem. Oikos. 85:282–294.
- Jung V, Hoffmann L, Muller S. 2009. Ecophysiological responses of nine floodplain meadow species to changing hydrological conditions. Plant Ecology. 201:589–598.
- Keddy P, Nielsen K, Weiher E, Lawson R. 2002. Relative competitive performance of 63 species of terrestrial herbaceous plants. Journal of Vegetation Science. 13:5–16.
- Keddy PA, Reznicek AA. 1986. Great Lakes vegetation dynamics: the role of fluctuating water levels and buried seeds. Journal of Great Lakes Research. 12:25–36.
- Keddy PA, Twolan-Strutt L, Wisheu IC. 1994. Competitive effect and response rankings in 20 wetland plants: are they consistent across three environments? Journal of Ecology. 82:635–643.
- Kennedy MP, Milne JM, Murphy KJ. 2003. Experimental growth responses to groundwater level variation and competition in five British wetland plant species. Wetlands Ecology and Management. 11:383–396.
- Laird RA, Schamp BS. 2008. Does local competition increase the coexistence of species in intransitive networks? Ecology. 89:237–247.
- Lenssen J, Menting F, van der Putten W, Blom K. 1999. Control of plant species richness and zonation of functional groups along a freshwater flooding gradient. Oikos. 86:523–534.
- Li S, Pezeshki SR, Goodwin S, Shields FD. 2004. Physiological responses of black willow (Salix nigra) cuttings to a range of soil moisture regimes. Photosynthetica. 42:585–590.
- Luan Z, Zhang G, Deng W, Hu J, Zhou D. 2007. Study on the changes of air temperature and precipitation in the last 50 years in the Sanjiang Plain. Journal of Arid Land Resources and Environment. 21:39–43 (Chinese).
- Luo W, Song F, Xie Y. 2008. Trade-off between tolerance to drought and tolerance to flooding in three wetland plants. Wetlands. 28:866–873.
- Luo W, Xie Y, Chen X, Li F, Qin X. 2010. Competition and facilitation in three marsh plants in response to a water-level gradient. Wetlands. 30:525–530.
- Mokany K, Ash J. 2008. Are traits measured on pot grown plants representative of those in natural communities? Journal of Vegetation Science. 19:119–126.
- Niedermeier A, Robinson JS. 2007. Hydrological controls on soil redox dynamics in a peat-based, restored wetland. Geoderma. 137:318–326.
- Niu S, Li Z, Xia J, Han Y, Wu M, Wan S. 2008. Climatic warming changes plant photosynthesis and its temperature dependence in a temperate steppe of northern China. Environmental and Experimental Botany. 63:91–101.
- Pennings SC, Grant MB, Bertness MD. 2005. Plant zonation in low-latitude salt marshes: disentangling the roles of flooding, salinity and competition. Journal of Ecology. 93:159–167.
- Pezeshki SR. 2001. Wetland plant responses to soil flooding. Environmental and Experimental Botany. 46:299–312.
- Pezeshki SR, Anderson PH, Shields FD. 1998. Effects of soil moisture regimes on growth and survival of black willow (Salix nigra) posts (cuttings). Wetlands. 18:460–470.
- Sammul M, Kull K, Oksanen L, Veromann P. 2000. Competition intensity and its importance: results of field experiments with Anthoxanthum odoratum. Oecologia. 125:18–25.
- Seabloom EW, van der Valk AG, Moloney KA. 1998. The role of water depth and soil temperature in determining initial composition of prairie wetland coenoclines. Plant Ecology. 138:203–216.
- Snow AA, Vince SW. 1984. Plant zonation in an Alaskan salt marsh: II. An experimental study of the role of edaphic conditions. Journal of Ecology. 72:669–684.
- Song C, Xu X, Tian H, Wang Y. 2009. Ecosystem–atmosphere exchange of CH4 and N2O and ecosystem respiration in wetlands in the Sanjiang Plain, northeastern China. Global Change Biology. 15:692–705.
- SPSS Inc. 2004. SPSS 13.0 Base users guide. Chicago: SPSS Inc.
- Wang L, Song C, Hu J, Yang T. 2010. Response of regeneration diversity of Carex Lasiocarpa community to different water levels in Sanjiang Plain, China. Chinese Geographical Science. 20:37–42.
- Weiher E, Wisheu IC, Keddy PA, Moore DRJ. 1996. Establishment, persistence, and management implications of experimental wetland plant communities. Wetlands. 16:208–218.
- Wilson SD, Keddy PA. 1986. Species competitive ability and position along a natural stress/disturbance gradient. Ecology. 67:1236–1242.
- Xie Y, Luo W, Wang K, Ren B. 2008. Root growth dynamics of Deyeuxia angustifolia seedlings in response to water level. Aquatic Botany. 89:292–296.
- Yan M, Deng W, Ma X. 2001. Climate variation in the Sanjiang Plain disturbed by large scale reclamation during the last 45 years. Acta Geographica Sinica. 56:159–170 (Chinese).