Abstract
To suppress the occurrence of phytoplankton blooms dominated by cyanobacteria, control measures were implemented during the bottleneck stages of algal succession, overwintering and pre-recruitment, using a combination of chemical and biological algicides. First, water and sediment samples were incubated in the laboratory to determine the threshold temperature for cyanobacteria recruitment. Second, the functional characteristics of candidate algicides were screened. Third, an in situ pilot experiment was conducted to test the feasibility of this method. Our results indicated that (1) the recruitment of cyanophytes was initiated at 15°C, (2) H2O2 and rice straw were the optimum algicides and their optimal concentrations were 10 mg/L and 1 g/L, respectively, and (3) following the combined application of H2O2 and rice straw during overwintering and pre-recruitment, the biomass of cyanobacteria decreased by 27.1% during recruitment and by 53.2% during the first algal bloom. These results suggested that the combined application of H2O2 and rice straw, during the bottleneck stages of algal cycling, suppressed the recruitment of cyanobacteria and decreased the magnitude of algal blooms, thus offering a promising choice for prevention of cyanobacterial blooms.
Introduction
Eutrophication, being aggravated in lakes and reservoirs, has led to massive occurrence of phytoplankton blooms. The occurrence of phytoplankton blooms reduces the recreational value of water bodies and causes serious ecological and social problems, such as loss of biodiversity and shortage of drinking water (Gangstad Citation1986; Vymazal Citation1995; Newman Citation1999; Guo Citation2007). Consequently, the prevention and controlling of algal blooms is of great importance. The abatement of nutrient levels in water bodies is thought to be fundamental for eradicating phytoplankton blooms; however, this is unrealizable in the short term (Johnes et al. Citation2007; Hudnell Citation2010). Therefore, emergency measures are urgently needed (Ridge et al. Citation1999).
Controlling measures of phytoplankton blooms usually fall into three categories: physical, chemical, and biological. Physical measures, such as sonication (Wu et al. Citation2011) and sedimentation with clay (Zou et al. Citation2006), are limited in practical application considering the huge cost and ineffectiveness in eradicating algal inocula. Chemical algicides are regarded as economical and effective in terms of their high efficiency and selective inhibition of target algae. However, traditional algicides, such as CuSO4 (Elder & Horne Citation1978) and butanoic acid (Nonomura et al. Citation2001), have been prohibited in some locales due to environmental safety concerns (Elder & Horne Citation1978). Therefore, new chemical algicides with fewer toxic side effects need to be explored or traditional chemical agents should be applied with additional safety measures, such as the sequestering of copper agents (Garris Citation2000).
Biological approaches mitigate the frequency and duration of algal blooms by employing a living species, such as a macrophyte, or its products. However, the direct establishment and restoration of macrophytes in water bodies experiencing eutrophication frequently fails due to harsh environments and the lower competitiveness of macrophytes. Alternatively, researchers have sought potential algicidal properties in botanical resources, such as barley straw (Welch et al. Citation1990; Newman & Barrett Citation1993), wheat (Ball et al. Citation2001), oak leaves (Ridge et al. Citation1999), and Ruta graveolens (Meepagala et al. Citation2005). In western countries, barley has been widely tested as a suppressant of algal blooms via allelopathy. In contrast, similar studies have not been carried out until recently in China, which suffers heavy cyanobacteria blooms (Xiao et al. Citation2010).
Typically, most control practices are implemented after the outbreak of algal blooms (Han & Kim Citation2001; Zou et al. Citation2006). In such cases, reversing the effects of large-scale algal blooms involves tremendous cost. Other mitigation strategies, such as managing algal development, were neglected (Sengco Citation2009). Based on the characteristics of algal growth in Lake Taihu, Kong and Gao Citation(2005) proposed the hypothesis of ‘four stages’ for bloom formation, in which overwintering and recruitment were identified as the bottleneck stages for algal development. During these periods, the amount of algae stock was greatly reduced and its distribution area was limited. Besides, cyanophytes could be more vulnerable to algicides when in the form of vegetative cells (Anderson Citation1976; Fallon & Brock Citation1981). Consequently, when control practices are implemented during these stages, the cost efficiency and efficiency of control could be greatly enhanced. Therefore, this practice can be categorized as a preventative strategy due to its implementation prior to the occurrence of algal blooms (Sengco Citation2009).
In this study, control measures were implemented during overwintering and recruitment stages in reservoir microcosms. First, the threshold temperature for the recruitment of cyanobacteria (the dominant bloom species in Lake Taihu) was identified by simulation of recruitment. Second, the functional characteristics of candidate algicides, including biological and chemical ones, were tested. Finally, using in situ enclosures, the optimal algicides were employed during bottleneck stages to verify removal effects. We hypothesized that after the combined application of chemical and biological algicides, the ‘seed bank’ of cyanobacteria could be abated and the recruitment of cyanobacteria could be suppressed in Lake Taihu.
Methods
Simulated recruitment
Algal inocula in the western and southwestern parts of Lake Taihu serve as a ‘seed bank’ for the cyanobacterial blooms (Kong et al. Citation2009), so the site ‘W1’ was selected as the sampling site for the recruitment study (). On 1 March 2010, the upper 0–2 cm of the sediment was collected with a polyethylene corer (10 cm diameter) and an integrated lake water sample (0–2 m in depth) was collected with a plastic column sampler (10 cm diameter). All samples were immediately transported to the laboratory.
In the laboratory, 18 flasks (three flasks per temperature gradient), each containing 25 ± 0.01 g (fresh weight) of homogenized sediment and 240 mL of lake water were sealed with a silica gel lid to allow free exchange of sterile air. Initially, flasks were placed in an incubation chamber in darkness at 6°C (average water temperature) for 5 days of adaptation. Thereafter, the cultivation conditions were adjusted to the following: a fluorescent lamp of 30 μmol photon m−2 s−1 light intensity on a 12:12 light:dark cycle with temperature increasing at a rate of 1°C every 5 days. All samples were kept still during the entire process. On the ending day of the series of temperature treatments, 6, 9, 12, 15, 18, and 21°C, the water column from each replicate flask was carefully removed for analysis.
The protocol for phycocyanin (PC) determination was as follows: 100 mL of water was filtered (Whatman, GF/C) and the filtration membrane was finely ground with 0.05 M Tris–HCl buffer at pH 7.0. The homogenate was stored in centrifuge tubes in darkness for 8–10 h at 4°C and then the supernatant liquid was separated by centrifugation at 6000 rpm for 20 min at 4°C. The detection of PC was conducted by colorimetry with a fluorescence spectrophotometer (RF-5301PC, Shimadzu Corporation, Japan). For the determination of chlorophyll a (Chl a) and chlorophyll b (Chl b), the same steps were repeated except for the replacement of Tris–HCl buffer at pH 7.0 by 90% acetone (v/v). The concentrations of Chl a, Chl b, and PC were indicative of the biomass of the total algae, non-cyanophytes, and cyanophytes, respectively (Cao et al. Citation2008).
Data were collected in triplicate and are presented as mean ± standard deviation (SD). The dynamics of algal recruitment were analyzed using a general linear model (univariate GLM; SPSS 13.3, SPSS Inc., USA) with temperature as the fixed variable. Differences between the means were considered significant when p < 0.05. The temperature of the first significant increase in pigments was considered as the beginning of algal recruitment.
Screening of algicides
The algicidal characteristics of four candidate algicides were tested: specifically, CuSO4 (99.0% purity; Nanjing Chemical Co., China), 30% H2O2 (m/m, Sigma), and the seed husk of rice and rice straw (cut into ∼1 cm sections) of cultivar Wuyujing (Japonica), which were freshly collected in the same year. Based on the dominant algal species in Lake Taihu, three axenic strains, including the target species, Microcystis aeruginosa (FACHB-915), Microcystis flos-aquae (FACHB-1028), and the control species Scenedesmus obliqnus (FACHB-416), were obtained from the Freshwater Algae Culture Collection of the Institute of Hydrobiology (Wuhan, China) for screening.
In the laboratory, algae in the exponential growth phase were inoculated into 100 mL of BG11 cultivation medium (Rippka et al. Citation1979) with a final cell concentration of approximately 5.0 × 105 cells/mL. Different concentration gradients of H2O2 and CuSO4 (0.1, 1, and 10 mg/L), rice husk and straw (0.01, 0.1, and 1 g/L, dry weight) were directly added to the treatments. All samples were grown in an incubation chamber with a fluorescent lamp of 30 μmol photon m−2 s−1 light intensity, on a 12:12 light:dark cycle at 25°C ± 1°C. The flasks were shaken twice a day. Cell numbers were counted after 0, 3, 6, 9, 12, 15, 18, 21, and 24 days of cultivation using a microscope with a 40× objective lens (CX41, Olympus Inc., Japan).
Data were collected in triplicate and presented as mean ± SD. The results are expressed as percentages of the control incubated without the addition of algicides:
where
and
represent the number of cells recorded in the treatment and in the control, respectively.
Pilot experiment
In recent years, Meiliang Bay has suffered heavy cyanobacteria blooms. So, site W2 was selected as the sampling site to test the efficacy of our method (). Near the bank, four adjacent enclosures (length × width × depth: 6 m × 5 m × 2.2 m) were employed, which were open to sediment with a water depth of about 1.8 m. Two of these enclosures were randomly selected as the controls and the other two as the treatments.
For treatments, the addition of 30% H2O2 was followed after 30 minutes (to avoid possible interactions) by rice straw. The rice straw was air-dried and cut into ∼20 cm segments and then packed into five plastic netting bags (‘straw sausages’, Newman Citation1999). In each enclosure, a total of five straw sausages (1.2 m in length) were attached to the four corners and the center. After the first occurrence of a phytoplankton bloom, the sausages were removed to avoid subsequent effects of the straw and to avoid inhibiting exchanges with the sediment. This procedure was conducted twice: once during overwintering and the other during the pre-recruitment period of cyanobacteria.
Based on the preliminary experiments, the optimal concentrations for H2O2 and rice straw were 10 mg/L and 1 g/L, respectively. Hence, the dosage of H2O2 and rice straw in the pilot experiment is expressed as
where
(6 m × 5 m × 1.8 m) and
represent the water volume in the enclosure and the optimal concentration of the algicides, respectively. Therefore, the dosage for 30% H2O2 (ρ = 1.11 g/cm3) and rice straw was 1460 mL and 54 kg, respectively.
The algicides were applied at half dosage twice. The first application was performed on 17 March 2011 (average water temperature = 9.95°C) on the condition that the average water temperature was below that of the initiation of algal recruitment. For the second application, a correlation was constructed between the forecast temperature, including the highest, average, and lowest air temperatures (http://qq.ip138.com/weather/jiangsu/WuXi.htm), and the actual average water temperature acquired from a meteorological station in Lake Taihu. Then, the date for the second application was chosen as 29 March 2011 (actual average water temperature = 13.45°C) because the predicted average water temperature was approaching the threshold for cyanobacteria recruitment.
Approximately 500 mL of integrated water samples (0–1.8 m depth) were collected weekly from 17 March 2011 to 2 June 2011 until the occurrence of the first algal bloom. Samples were immediately transported to the laboratory. Pigments were determined as describe above. Total nitrogen (TN) and total phosphorus (TP) were determined according to the Chinese Standard Methods for Lake Eutrophication Survey (Jin & Tu Citation1990) using a combined persulfate digestion followed by spectrophotometric analysis as described for soluble reactive phosphorus and nitrate. In addition, for the identification of algal species, 200 mL of the water samples were preserved with Lugol's iodine for 24 h and then the condensed deposits (30 mL) were collected and stored at 4°C until analysis. All samples were precipitated for 48 h before identification and the deposition was detected using an Olympus-CX41 microscope with a 40× objective lens.
The removal efficacy of cyanobacteria is expressed as
where
and
represent the PC concentrations in the treatment and in the control, respectively.
The removal efficacy of M. aeruginosa is expressed as follows:
where
and
represent the proportion of M. aeruginosa in the cyanobacteria and the concentration of PC in the treatment, and
and
represent the proportion of M. aeruginosa in the cyanobacteria and the PC concentration in the control, respectively. Data collected from enclosures are presented as mean ± SD. The difference between the treatment and control was analyzed by t-tests. Differences were considered significant when p < 0.05.
Results
The changes in the pigment concentrations during recruitment are shown in . Based on the time of the first significant changes in pigment concentrations, the recruitment of total algae, non-cyanophytes, and cyanophytes was initiated at 12, 12, and 15°C, respectively (ANOVA, p < 0.05).
Figure 2. Concentration of pigments during simulated recruitment. Data are the averages with the SD (error bars) of triplicates. Note: * represents the first significant increment.
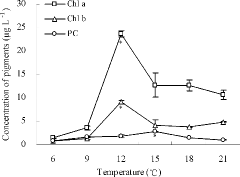
The effects of H2O2 and rice straw on M. aeruginosa, M. flos-aquae, and S. obliqnus are shown in . For H2O2 ((A)–3(C)), at 0.1 and 1 mg/L, the average percentages of inhibition on M. flos-aquae and M. aeruginosa during the entire process were below 50%. At 10 mg/L, the target inhibition percentage (>95%) was observed from the 3rd day to the 9th day for M. flos-aquae and from the 3rd day to the 15th day for M. aeruginosa. The average percentage of inhibition for S. obliqnus was 37.04%. Consequently, the optimal concentration of H2O2 was 10 mg/L and its duration was more than 9 days.
Figure 3. Effects of candidate algicides on algae. Panels A, B, and C represent the effects of H2O2 on Microcystis flos-aquae, M. aeruginosa, and Scenedesmus obliqnus, respectively. Panels D, E, and F represent the effects of rice straw on M. flos-aquae, M. aeruginosa, and S. obliqnus, respectively. Data are the averages with SD (error bars) of triplicates.
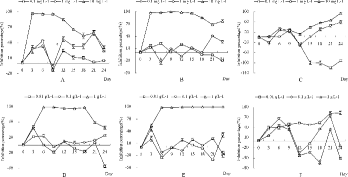
For rice straw ((D)–3(F)) at 0.01 and 0.1 g/L, the average percentages of inhibition of M. flos-aquae and M. aeruginosa during the entire process were below 50%. At 1 g/L, the expected percentage of inhibition (>95%) was observed from the 6th day to the 18th day for M. flos-aquae and from the 6th day to the end of the experiment for M. aeruginosa. The average percentage of inhibition was −6.21% for S. obliqnus. Consequently, the optimal concentration of rice straw was 1 g/L and its duration was more than 18 days.
For CuSO4 at 10 mg/L, the target percentage of inhibition (>95%) was observed from the 12th day until the end of the experiment for M. flos-aquae and M. aeruginosa. However, the percentage of inhibition for S. obliqnus reached 96.15% from the 6th day (data not shown). For rice husk, at 1 g/L, the average percentages of inhibition during the entire process were below 10% for both M. flos-aquae and M. aeruginosa, whereas the average percentage for S. obliqnus was 55.54% (data not shown). Consequently, H2O2 and rice straw were the optimum algicides that served as short-term and longer term algicides, respectively. CuSO4 and seed husk were abandoned because of their side effect on non-cyanophytes and their low inhibition of cyanophytes, respectively.
The changes in the concentrations of Chl a, Chl b, and PC in the pilot experiment are shown in (A) and 4(B). Algal recruitment was initiated on 24 April 2011, based on the significant changes in Chl a (t-test, p < 0.05) when the actual average water temperature was 17.45°C. The first algal bloom occurred on 2 June 2011 when the water column was full of algal cells based on field observation. On 24 April 2011, the concentrations of Chl a, Chl b, and PC in the control were 25.43 ± 0.35, 8.50 ± 0.26, and 4.01 ± 0.13 μg/L, respectively, whereas those in the treatment were 15.54 ± 0.38, 4.69 ± 0.28, and 2.92 ± 0.01 μg/L, respectively. The proportions of M. aeruginosa in the control and the treatment were 100% and 44.44%, respectively. Thus, the removal efficiencies for cyanobacteria and M. aeruginosa were 27.09% and 67.60%, respectively. On 2 June 2011, the concentrations of Chl a, Chl b, and PC in the control were 26.18 ± 0.69, 6.17 ± 0.46, and 5.45 ± 0.43 μg/L and 21.64 ± 0.35, 6.92 ± 0.11, and 2.55 ± 0.06 μg/L, respectively, in the treatment. The proportions of M. aeruginosa in the control and the treatment group were 93.39% and 92.37%, respectively. Hence, the removal efficiencies for cyanobacteria and M. aeruginosa were 53.18% and 53.69%, respectively.
Figure 4. Concentrations of pigments in in situ enclosures: (A) Chl a and Chl b; and (B) PC. Data are the averages with SD (error bars) of two replicates. Notes: denotes algal recruitment and denotes the first algal bloom.
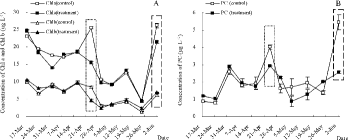
The proportion of M. aeruginosa among the cyanobacteria is shown in . During the entire experiment, four cyanobacteria species, Microcystis aeruginosa Kütz, Dactylococcopsis irreguloris Smith, Aphanizomenon gracile Lemmermann, and Pseudanabaena mucicola, were detected. The changes of TP and TN concentrations in the control and the treatment are shown in . No significant differences were detected between the treatment and the control (t-test, p < 0.05).
Table 1. Proportion of M. aeruginosa in cyanophytes (%) from integrated water samples from within the experimental enclosures. Treatment enclosures received H2O2 and rice straw.
Discussion
The results illustrate that the combined application of H2O2 and rice straw during overwintering and pre-recruitment of cyanobacteria successfully decreased the recruitment of cyanobacteria and mitigated the intensity of the subsequent algal bloom. Though our measures might only delay the occurrence of bloom formation, many fewer Microcystis cells were potentially available to build a new benthic phase in autumn and winter, thus leading to weaker algal blooms in the next year. In addition, in contrast with former studies (Mcknight et al. Citation1983; Zou et al. Citation2006; Barrington & Ghadouani Citation2008; Wu et al. Citation2011), this method takes the growth characteristics of algae into consideration and was implemented during the pre-algal boom, rather than the post-algal bloom, thus avoiding possible negative impacts caused by the outbreak of algal blooms.
To eliminate algal inocula in winter and inhibit algal recruitment in early spring, physical methods were not economical due to the scarcity of algae in the water column. Alternatively, chemical algicides could be effective because of their high efficiency and selective inhibition of algae (Elder & Horne Citation1978; McKnight et al. Citation1983; Pillinger et al. Citation1994; Nonomura et al. Citation2001; Barrington & Ghadouani Citation2008; Qian et al. Citation2010). However, to overcome the shortcomings of the transient duration of chemical reagents, complementary measures like the use of plant materials (Ridge et al. Citation1999) need to be adopted.
Currently, chemical reagents are widely prohibited due to increasing concerns for environmental safety. For example, CuSO4 is not biodegradable and is toxic to many aquatic organisms when accumulated to high levels (Roman et al. Citation2007). H2O2, however, is a natural photochemical product in water and is readily degraded into oxygen and water (Cooper & Zika Citation1983). In the field, the usage of H2O2 has been shown to be harmless to eukaryotic phytoplankton, zooplankton and macrofauna (Tang et al. Citation2005; Matthijs et al. Citation2012). Furthermore, H2O2 shows selective inhibition of the growth of cyanobacteria (McKnight et al. Citation1983; Barrington & Ghadouani Citation2008) by blocking the transcription of photosynthesis-related genes and destruction of photosynthetic pigments (Qian et al. Citation2010). More importantly, it can decrease the concentration of microcystin by down-regulating mcyD transcription (Qian et al. Citation2010). In the current experiments, H2O2 exhibited target inhibition of cyanobacteria (>95%) more rapidly than CuSO4 and the environmental safety after application could also be expected because of its short lifetime ((A)–3(C)).
Using the plant residue as an algicide offers several advantages: low cost, effectiveness, and environmental safety. Welch et al. Citation(1990) first reported the inhibitory ability of a crop residue, barley straw, on cyanobacteria. Its inhibitory ability results from allelochemicals, such as phenolics and quinines (Ridge & Pillinger Citation1996), directly released by straw or degraded from lignin via shikimate pathways (Falch Citation1996). Barley straw has been successfully applied to suppress the occurrence of algal blooms in many lakes (Welch et al. Citation1990; Caffrey & Monahan Citation1999), though some controversy exists because of the instability of inhibitors and the diverse resistance of algae (Pillinger et al. Citation1994; Ridge et al. Citation1999). Therefore, the potential algicidal ability of rice straw, also a member of the Poaceae family like barley, deserves to be explored. In China, rice (Oryza sativa) is the most important food, with a cultivation area of 29,241 × 106 ha (in 2010). Wuyujing (japonica) is widely cultivated in the lower reaches of the Yangtze River, especially in the areas surrounding Lake Taihu, for its excellent eating quality. Previously, rice straw was often burned in paddies and caused serious air pollution in local areas. So, rice straw is easily available and the proper utilization of rice straw was desirable. In current experiments, rice straw exhibited selective and strong inhibition on cyanobacteria growth (), and the nutrient levels were not altered significantly after the addition of rice straw ().
The biomass of cyanobacteria and M. aeruginosa decreased by 27.18% and 67.60%, respectively, during recruitment and by 53.18% and 53.69%, respectively, during the first algal bloom. These results are somewhat satisfactory, but the abatement of cyanobacteria stock and the cyanobacteria biomass from recruitment to an algal bloom did not appear as expected. The inhibition efficacy was notable only when algal biomass reached a comparatively high level, so we infer that algae in the propagation stage could be more susceptible to algicides, while varied sensitivity of different algal species exists (Ridge et al. Citation1995). Further studies to illustrate algal sensitivity in response to algicides in different developmental stages of algae are needed.
Aerobic conditions have been demonstrated to be vital for the release of allelochemicals from some plant residues (Gibson et al. Citation1990; Pillinger et al. Citation1994). In this experiment, the straw sausages were submerged. Therefore, the anoxic environment could have slowed the release of inhibitors and weakened the algicidal activity, while the duration of rice straw was extended to more than 66 days (from 29 March to 2 June 2011), much longer than that in the preliminary experiment. However, when applied on a large scale, more pronounced inhibition could be expected in the short term due to sufficient supply of dissolved oxygen in the water column by wind-stirring. On the other hand, the inhibition of chemical reagents is related to water chemistry (McKnight et al. Citation1983) and to the occurrence and reactions of synthetic organic compounds (Fitzgerald & Faust Citation1963; Manahan & Smith Citation1973). Hence, the inhibition ability of H2O2 could be partially undermined by the addition of rice straw.
The proper dosage of algicides is critical for removal efficacy. At the lowest concentration, the inhibition by algicides could be reversed into promotion (). The optimal concentration of rice straw (1.0 g/L) was much higher than that of barley (2.57 × 10−3 g/L, Newman & Barrett Citation1993) but lower than that of Tibetan hulless barley straw (2.0 to 8.0 g/L, Xiao et al. Citation2010), and barley straw (7.2 g/L, Ferrier et al. Citation2005). The optimal concentration of H2O2 (10 mg/L) was near that of other chemical agents (Elder & Horne Citation1978). In the pilot experiment, however, the algicide concentration could have been diluted by rainfall. Consequently, the proper dosage of algicides for best removal efficacy should be modified according to plant species, physico-chemical characteristics of the lake water, and weather conditions.
The correspondence of the time for cyanobacteria recruitment in both the simulation experiment and the field experiment was crucial for removal efficacy. The predicted date of the second application was assumed to be close to that of cyanobacteria recruitment in the field, but a time lag of up to 26 days emerged, from 29 March 2011 to 24 April 2011. This time lag could partially decrease algicidal ability because it deprived H2O2 and rice straw of the best opportunity to suppress cyanobacteria recruitment. This deviation resulted from the temperature difference of cyanobacteria recruitment between the simulated experiment (15°C) and the field conditions (17.5°C). In the field, the water temperature was averaged by real-time data, whereas in the laboratory it was constant over the day. Hence, part of the cumulative temperature (Cao et al. Citation2008) at higher temperatures in the field could be offset by energy compensation consumed at lower temperatures. Consequently, the characteristics of cyanobacteria recruitment under variable temperature need to be explicated in order to further optimize the date for the second application.
In conclusion, this method has several advantages: (a) the algicides of H2O2 and rice straw meet the requirements of selective inhibition of cyanobacteria, and environmental safety; (b) the control method implemented during the bottleneck stages of algal cycling is technically feasible, economical, and efficient; and (c) the combined application of H2O2 and rice straw inhibited cyanobacteria recruitment and mitigated the intensity of the early bloom. Although many details need to be optimized, this strategy is of theoretical importance because it offers a promising alternative for controlling algal blooms in a different way.
Acknowledgements
We are grateful to the Taihu Laboratory for Lake Ecosystem Research for their kindly provision of enclosures.
Additional information
Funding
References
- Anderson OR. 1976. Respiration and photosynthesis during resting cell formation in Amphora coffeaeformis (Ag.). Kütz Limnol Oceanogr. 21:452–456.
- Ball AS, Williams M, Vincent D, Robinson J. 2001. Algal growth control by a barley straw extract. Bioresour Technol. 77:177–181.
- Barrington DJ, Ghadouani A. 2008. Application of hydrogen peroxide for the removal of toxic cyanobacteria and other phytoplankton from wastewater. Environ Sci Technol. 42:8916–8921.
- Caffrey JM, Monahan C. 1999. Filamentous algal control using barley straw. Hydrobiologia. 415:315–318.
- Cao HS, Tao Y, Kong FX, Yang Z. 2008. Relationship between temperature and cyanobacterial recruitment from sediments in laboratory and field studies. J Freshwater Ecol. 23:405–412.
- Cooper WJ, Zika RG. 1983. Photochemical formation of H2O2 in surface and ground waters exposed to sunlight. Science. 220:711–712.
- Elder JF, Horne AJ. 1978. Copper cycles and CuSO4 algicidal capacity in two California lakes. Environ Manage. 2:17–30
- Falch B. 1996. Was steckt in Cyanobakterien? Pharm Unserer Zeit. 25:311–321.
- Fallon RD, Brock TD. 1981. Overwintering of Microcystis in Lake Mendota. Freshwater Biol. 11:217–226.
- Ferrier MD, Butler BR, Terlizzi DE, Lacouture R. 2005. The effects of barley straw (Hordeum vulgare) on the growth of freshwater algae. Bioresour Technol. 96:1788–1795.
- Fitzgerald GP, Faust SL. 1963. Factors affecting the algicidal and algistatic properties of copper. Appl Microbiol. 11:345–351.
- Gangstad EO. 1986. Freshwater vegetation management. Fresno (CA): Thomas Publications.
- Garris J. 2000. An algicide using anionic polymers to sequester and stabilize copper in an oxidizing aqueous environment. In: Amjad Z, editor. Advances in crystal growth inhibition technologies. New York: Springer; p. 207–213.
- Gibson MT, Welch IM, Barrett PRF, Ridge I. 1990. Barley straw as an inhibitor of algal growth II: laboratory studies. J Appl Phycol. 2:241–248.
- Guo L. 2007. Doing battle with the green monster of Taihu Lake. Science. 317:1166.
- Han MY, Kim W. 2001. A theoretical consideration of algae removal with clays. Microchem J. 68:157–161.
- Hudnell HK. 2010. The state of U.S. freshwater harmful algal blooms assessments, policy and legislation. Toxicon. 55:1024–1034.
- Jin X, Tu Q. 1990. The standard methods for observation and analysis in Lake Eutrophication, 2nd ed. ( in Chinese). Beijing: Chinese Environmental Science Press.
- Johnes PJ, Foy R, Butterfield D, Haygarth PM. 2007. Land use scenarios for England and Wales: evaluation of management options to support ‘good ecological status’ in surface fresh water. Soil Use Manage. 23:176–194.
- Kong F, Gao G. 2005. Hypothesis on cyanobacteria bloom-forming mechanism in large shallow eutrophic lakes (in Chinese). Acta Ecol Sin. 25:589–595.
- Kong F, Ma R, Gao J, Wu X. 2009. The theory and practice of prevention, forecast and warning on cyanobacteria bloom in Lake Taihu (in Chinese). J Lake Sci. 21:314–328.
- Manahan SE, Smith MJ. 1973. Copper micronutrient requirements for algae. Environ Sci Technol. 7:829–833.
- Matthijs HC, Visser PM, Reeze B, Meeuse J, Slot PC, Wijn G, Talens R, Huisman J. 2012. Selective suppression of harmful cyanobacteria in an entire lake with hydrogen peroxide. Water Res. 46:1460–1472.
- McKnight DM, Chisholm SW, Harleman DRF. 1983. CuSO4 treatment of nuisance algal blooms in drinking water reservoirs. Environ Manage. 7:311–320.
- Meepagala KM, Schrader KK, Wedge DE, Duke SO. 2005. Algicidal and antifungal compounds from the roots of Ruta graveolens and synthesis of their analogs. Phytochemistry. 66:2689–2695.
- Newman J. 1999. Control of algae with straw (Information Sheet 3). Berkshire:Centre for Aquatic Plant Management.
- Newman JR, Barrett PRF. 1993. Control of Microcystis aeruginosa by decomposing barley straw. J Aquat Plant Manage. 31:203–206.
- Nonomura T, Matsuda Y, Bingo M, Onishi M, Matsuda K, Harada S, Toyoda H. 2001. Algicidal effect of 3-(3-indolyl)butanoic acid, a control agent of the bacterial wilt pathogen, Ralstonia solanacearum. Crop Prot. 20:935–939.
- Pillinger JM, Cooper JA, Ridge I. 1994. Role of phenolic compounds in the antialgal activity of barley straw. J Chem Ecol. 20:1557–1569.
- Qian H, Yu S, Sun Z, Xie X, Liu W, Fu Z. 2010. Effects of copper sulfate, hydrogen peroxide and N-phenyl-2-naphthylamine on oxidative stress and the expression of genes involved photosynthesis and microcystin disposition in Microcystis aeruginosa. Aquat Toxicol. 99:405–412.
- Ridge I, Pillinger JM. 1996. Towards understanding the nature of algal inhibitors from barley straw. Hydrobiologia. 340:301–305.
- Ridge I, Pillinger JM, Walters J. 1995. Alleviating the problems of excessive algal growth. In: Harper DM, Ferguson AJD, editors. The ecological basis for river management. Chichester: John Wiley; p. 211–218.
- Ridge I, Walters J, Street M. 1999. Algal growth control by terrestrial leaf litter: a realistic tool? Hydrobiologia. 395–396:173–180.
- Rippka R, Deruelles J, Waterbury JB, Herdman M, Stanier RY. 1979. Generic assignments, strain histories and properties of pure cultures of cyanobacteria. Microbiology. 111:1–61.
- Roman YE, De Schamphelaere KAC, Nguyen LTH, Janssen CR. 2007. Chronic toxicity of copper to five benthic invertebrates in laboratory-formulated sediment: sensitivity comparison and preliminary risk assessment. Sci Total Environ. 387:128–140.
- Sengco MR. 2009. Prevention and control of Karenia brevis blooms. Harmful Algae. 8:623–628.
- Tang JYM, Anderson DM, Au DWT. 2005. Hydrogen peroxide is not the cause of fish kills associated with Chattonella marina: cytological and physiological evidence. Aquat Toxicol. 72:351–360.
- Vymazal J. 1995. Algae and element cycling in wetlands. Boca Raton (FL): Lewis Publishers.
- Welch IM, Barrett PRF, Gibson MT, Ridge I. 1990. Barley straw as an inhibitor of algae growth I: studies in the Chesterfield Canal. J Appl Phycol. 2:231–239.
- Wu X, Joyce EM, Mason TJ. 2011. The effects of ultrasound on cyanobacteria. Harmful Algae. 10:738–743.
- Xiao X, Chen Y, Liang X, Lou L, Tang X. 2010. Effects of Tibetan hulless barley on bloom-forming cyanobacterium (Microcystis aeruginosa) measured by different physiological and morphologic parameters. Chemosphere. 81:1118–1123.
- Zou H, Pan G, Chen H, Yuan X. 2006. Removal of cyanobacterial blooms in Taihu Lake using local soils. II. Effective removal of Microcystis aeruginosa using local soils and sediments modified by chitosan. Environ Pollut. 141:201–205.