Abstract
We investigated how the richness and ecological characteristics of non-marine ostracod species varied across nine 100-m elevation bands (from 549 to 1457 m) in the Çankırı region. We collected a total of 37 taxa (25 living and 12 sub-recent) from 114 of 130 aquatic sites sampled during September of 2011; 34 of the taxa were new records for the region. Eight widely distributed ‘cosmopolitan’ species (Candona neglecta, Cypridopsis vidua, Heterocypris incongruens, Ilyocypris bradyi, Limnocythere inopinata, Potamocypris villosa, Prionocypris zenkeri, and Psychrodromus olivaceus) were found more frequently than other species. The first two axes of canonical correspondence analyses (CCA) explained 73.2% of the correlation between 11 species and 5 environmental variables. Of which, electrical conductivity (F = 3.99, p = 0.028) and altitude (F = 2.69, p = 0.004) were the most explanatory (p < 0.05) variables for species. Optimum altitude and water temperature values of the cosmopolitan species were relatively higher than the other species. Significant regression models (p < 0.01, r2 = 0.81) indicate that the frequency of occurrence was affected by changes in numbers of samplings sites from 549 to 1457 m. When highest numbers of species (15 spp.) were found at the range of 1231–1332 m, numbers of asexual species (10 spp.) were twice of the sexual species (5 spp.) with no statistical difference between numbers of swimming (7 spp.) and non-swimming (8 spp.) species. Results were discussed based on the ecological conditions that individual species prefer.
Introduction
Ostracoda is one of the oldest crustacean groups; fossils of Ostracoda date back to the Cambrian age (Neil & Bell Citation2006). Because their carapace consists mainly of CaCO3, fossilized forms can persist for long periods in sediments and soil. Ambient water is the main source of calcium for ostracods (Turpen & Angell Citation1971; Rosenfeld Citation1982; Majoran et al. Citation1999); therefore, having information about carapace chemistry allows comparisons with water chemistry. Because most (if not all) ostracod species prefer certain types of conditions in different aquatic habitats, ostracods can serve as indicator species of water quality. For example, Heterocypris incongruens was suggested as an indicator of organic pollution (Rosenfeld and Ortal Citation1983; Mezquita et al. Citation1999) since this well-known, cosmopolitan species has been reported from different types of fresh and brackish waters. Eventually, knowledge of species distributions can also be used to estimate present and future conditions in such habitats. Indeed, ostracods can be found in diverse aquatic bodies that span from the deep sea (4000 m below sea level; Steineck et al. Citation1990) to sea level (Külköylüoğlu et al. Citation1993; Pieri et al. Citation2009) to high elevations (Laprida et al. Citation2006; Wrozyna et al. Citation2009; Guo et al. Citation2013). For example, Wrozyna et al. (Citation2009) reported both living and fossil ostracod assemblages from Lake Nam Co in Tibet at about 4718 m above sea level. Although these studies illustrate that ostracods can inhabit different aquatic bodies spanning >9000 m of elevational range, we still have little knowledge about how species composition and abundance vary with elevation. Külköylüoğlu, Sarı, and Akdemir (Citation2012) Külköylüoğlu, Akdemir, and Yüce (Citation2012) and Uçak et al. (Citation2014) did not find significant relationships between elevation and ostracod species distribution in Turkey. In contrast, a few studies on ostracods (e.g., see Mezquita et al. Citation1999; Pieri et al. Citation2009) and zooplankton (Shehu et al. Citation2009) illustrated the opposite in Spain, Italy, and Albania-Greece, respectively. According to these studies, the topic is still controversial and needs to be studied in detail. Such lack of knowledge requires critical questions regarding ostracods's (1) modes of dispersal (e.g., active vs. passive dispersal), (2) ecological adaptive values along with suitability of aquatic conditions (e.g., ecological optimum and tolerance ranges), and (3) variation in population assemblages (e.g., diversity, abundance, and reproductive success). The aims of this study were to (1) perform the first extensive survey of ostracods in the Çankırı region (Turkey), (2) elucidate the species’ elevational distribution, and (3) quantify the relationships between environmental variables and species distribution.
Methods
Site description and sampling
A total of 130 sites () that included 12 different types of aquatic habitats (lake, reservoir, pond, pool, wetland, well, spring, creek, stream, irrigation canal, trough, and ditch) was randomly visited in Çankırı province (40° 36′ N, 33° 37′ E, 7388 km2 of surface area) during 10–13 September 2011. At each site, we recorded data on seven aquatic variables (see Supplemental Table S1), including dissolved oxygen (DO), percent oxygen saturation, electrical conductivity (EC), total dissolved solids, salinity, pH, and water temperature (Tw) using a handheld meter (Professional Plus, YSI, Inc., http://www.ysi.com). We recorded air temperature (°C), wind speed (km h−1), and relative humidity (%, Model 410-2, Testo, http://www.testo.com). Additionally, geographic data (e.g., elevation, latitude, and longitude) were recorded with GPS (eTrex Vista HCX, Garmin, http://www.garmin.com). To prevent the possible consequences of pseudoreplication (Hurlbert Citation1984), all variables were measured before sampling. Samples were collected from each site with a standard-sized hand net (mesh size = 200 µm) and stored in 250-mL plastic bottles in a 70% alcohol solution for fixing. In the laboratory, samples were filtered through four standardized sieves (0.25, 0.5, 1.0, and 1.5 mm mesh size) under tap water and then kept in 70% alcohol for further studies. Sieves were used to separate ostracods from debris in different sizes. Ostracods were separated from sediments under a stereomicroscope (Olympus SZX-TR30) and dissected in lactophenol solution for taxonomic identification. We retained the carapace and valves on micropaleontological slides. Species identification primarily followed taxonomic keys of Meisch (Citation2000) and Bronshtein (Citation1988). All biological samples were accessioned in the Limnology Laboratory of Abant İzzet Baysal University, Bolu, Turkey.
Statistical Analysis
We examined the data with detrended correspondence analysis (DCA) to see its suitability for canonical correspondence analysis (CCA). DCA is one of the most commonly used unconstrained ordination methods in which the length of the first axis corresponds to the heterogeneity or homogeneity of the data. Therefore, this length is used to make decisions about the use of linear (length < 3) or unimodal (length > 4) ordination methods. A gradient length > 3 suggests suitability of data for CCA (Ter Braak & Barendregt Citation1986; Ter Braak Citation1987; Birks et al. Citation1990). Our DCA length was 4.488 suggesting a good fit of the data. Then, CCA was used to display relationships between 11 species (with 3 or more occurrences) and the 5 most commonly used environmental variables (EC, Tw, DO, pH, and elevation). To eliminate the influence of multicolinearity and arc-effect, rare species were removed and the data was log-transformed and tested with the Monte Carlo permutation test (499 permutations).
The C2 program was used to measure ecological tolerance and optimum estimates of each species for different environmental variables after using a transfer function of weighted averaging (WA) regression (Juggins Citation2003). Application of C2 analyses consisted of 11 species that occurred at least three times from the sampling sites where data on environmental variables were available. During WA, optimum values for each species are considered as the mean of all sites where the species occurs, weighted by its abundance in each. Herein, it is assumed that all species express unimodal responses to the environmental variables selected. Thus, a species can be most abundant in sampling sites where the values are closest to their optimum estimates (Ter Braak & Barendregt Citation1986).
We also used regression analyses in Microsoft Excel 2010 to display the distribution of numbers of species and sampling sites among nine elevational ranges from 549 to 1457 m. We only used living adult species in all of our statistical analyses while juveniles, damaged specimens, and subfossils were eliminated from the analyses.
Results
During this study, we detected 37 taxa collected from 114 of our 130 sites. Among them, 34 taxa are new records for the region; they include 25 species from 89 sites (see Supplemental Table S2). Including the earlier reports, the area is now known to contain at least 39 ostracod taxa, including 27 living species. Eight species (Candona neglecta, Cypridopsis vidua, H. incongruens, Ilyocypris bradyi, Limnocythere inopinata, Potamocypris villosa, Prionocypris zenkeri, and Psychrodromus olivaceus) occurred more frequently than the others. Most of these cosmopolitan species showed higher optimum values for altitude (Alt) and Tw than the means of the other species (). Regression analyses displayed positive correlation between species occurrence and sampling sites (p < 0.01) such that 81.5% of the variation in numbers of species was explained by variation in numbers of sampling sites. Accordingly, numbers of species per station were slightly decreased. CCA exhibited 73.2% of the correlation between 11 species and 5 environmental variables, EC (F = 3.99, p = 0.028) and Alt (F = 2.69, p = 0.004) were found to be the two most effective (p < 0.05) factors explaining species occurrence (, ). We detected the greatest number of species (15 spp.) in the range from 1231 to 1332 m (). Within this elevational range, the number of asexual species (10 spp.) was twice that of the sexual species (5 spp.). There was no statistical difference in the numbers of swimming (seven spp.) and non-swimming (eight spp.) species within this range (1231–1332 m). Although swimming species were found in all elevational ranges, non-swimming species were absent from 525 to 726 m. Numbers of non-swimming species were always higher than the swimming species from 828 to 1332 m.
Table 1. Optimum (Opt) and tolerance (Tol) values of 11 ostracods for five environmental variables along with the mean, maximum (Max) and minimum (Min) levels. Abbreviations: Count (numbers of occurrences), Maxi (maximum numbers of individuals), N2 (Hill's coefficient value), Alt (altitude, m), Tw (water temperature, °C), DO (dissolved oxygen, mg/L), EC (electrical conductivity, µS/cm).
Figure 2. CCA diagrams of (a) five environmental variables (arrows) and 11 species in (b) 88 sampling sites. Abbreviations (also see Suuplemental Tables S1 and S2): Cn, Candona neglecta; Cv, Cypridopsis vidua; Hi, Heterocypris incongruens; Hs, H. salina; Ib, Ilyocypris bradyi; Ig, Ilyocypris gibba; Li, Limnocythere inopinata; Pf, Psychrodromus fontinalis; Po, P. olivaceus; Pv, Potamocypris villosa; Pzi, Prionocypris zenkeri; Alt, altitude (m); DO, dissolved oxygen (mg/l); EC, electrical conductivity (µs/cm); Tw, water temperature (°C).
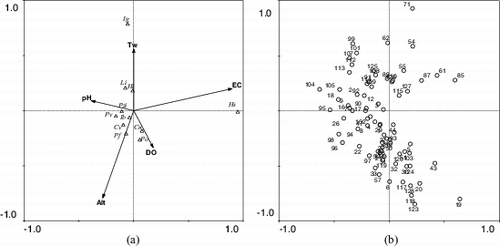
Table 2. Summary of CCA result. First two axes explained 73.2% of the relationships between 11 species and 5 environmental variables. Rare species are down weighted and log-transformed. *Shows the results of DCA.
Discussion
Before this study, only four nonmarine ostracod species were known from the Çankırı region. Schäfer (Citation1952) was the first to report two species (Ilyocypris brehmi and Potamocypris arcuata), and Gülen et al. (Citation1994) listed two more species (Ilyocypris gibba and Heterocypris salina) from Lake Kırkpınar. Note that Schäfer (Citation1952) did not give details about his sampling locations, except that he pointed out the name ‘Ilgar – Sea’ as the sampling site. After extensive searches regarding the location, we have not found any location named ‘Ilgar – Sea’ (‘Ilgaz Gölü’ in Turkish). Therefore, the type of localities of I. brehmi and P. arcuata reported in 1952 by Schäfer are not exactly determined. Two of these species (I. gibba and H. salina) were again encountered in this study. Including the earlier report, the area now comprises 27 living species.
Comparing with the other studies done in Turkey, species diversity of Çankırı is relatively low. For example, the surface area of Çankırı is about 7388 km2 where a total of 27 species are known from 122 sites. This means that about 0.22 species can be found in each sampling site along with 0.0036 species per km2. However, these ratios differ from the Van region (Külköylüoğl, Sarı, and Akdemir Citation2012) where a total of 30 species from 78 sites was reported within 10,667 km2 of surface area covered (note that this is half of the actual size since almost half of the area was sampled), in which numbers of species per sampling site was 0.38 along with 0.003 species per km2. Similarly, numbers of species per sampling site was 0.33 and numbers of species per km2 was about 0.002 in Diyarbakır (15,722 km2, 29 species from 90 sites) (Akdemir & Külköylüoğlu Citation2011). As was the case in Kahramanmaraş (14,346 km2, 95 sites; Külköylüoğlu, Akdemir, and Yüce Citation2012), the number of species per sampling site was 0.33 with 0.002 species per km2. In contrast, these values are similar between Çankırı and Adıyaman (7164 km2, 120 sites; Yavuzatmaca et al. Citation2015) where numbers of species per sampling site was 0.22 with 0.003 species per km2. Several abiotic factors can affect species diversity. We did not include biotic factors in our study but we can at least mention three other factors that likely play critical roles in shaping species diversity.
Seasonality
Our field sampling spanned only four days in September 2011. Thus, we have not addressed any possible effects of seasonality here. However, it is well known that several ostracods do exhibit seasonality within their life cycle (Külköylüoğlu Citation1998; Mezquita et al. Citation2002; Külköylüoğlu et al. Citation2007; Dügel et al. Citation2008), and such seasonality can be species specific. Therefore, performing sampling across more portions of the year may likely increase the numbers of species known from the region.
Sampling effect
Low species diversity may reflect the numbers of sampling sites. We did not perform any a-posteriori power analyses but increasing the number of sites sampled could well increase the number of species (i.e., a ‘sampling effect’; Hill et al. Citation1994). However, comparative analyses among this and previous studies do not support this view. For example, in Europe, Nagorskaya & Keyser (Citation2005) found 62 species from 156 different sites of Belarus while Pieri et al. (Citation2009) reported 53 living species of 74 taxa collected from 200 sites (with 320 samples) of Friuli Venezia Giulia (Italy) region. In Brazil (South America), 54 ostracods were collected from 48 sites (with 132 samples) by Higuti et al. (Citation2010). In Turkey, Külköylüoğlu, Sarı, and Akdemir (Citation2012), Külköylüoğlu, Sarı, Akdemir, Yavuzatmaca, and Altınbağ (Citation2012), Külköylüoğlu, Yavuzatmaca, Akdemir, Sarı (Citation2012), and Uçak et al. (Citation2014) reported 29, 26, 30, and 31 species from 57, 133, 68, and 152 sites in northern Van, Ordu, Kahramanmaraş, and Ankara, respectively. As seen from these studies, increasing the number of sites sampled does not necessarily increase the number of species detected. Nonetheless, performing species accumulation curves and sampling more sites together may better elucidate patterns of richness.
Habitat suitability
According to Williams (Citation1943), species richness is related to the availability of different habitats, such that richness increases with increasing available habitats (i.e., the ‘habitat diversity hypothesis’). The most recent studies of ostracods in Turkey (Külköylüoğlu, Yavuzatmaca, Akdemir, and Sarı Citation2012 and Uçak et al. Citation2014) support this hypothesis. Besides, similar results were found in this study but these results are not generalized here for the following reasons. For example, different ecological conditions (e.g., water quality) and geographical (e.g., elevation) factors or both affect species richness and diversity. As stated above, ostracod species do have species-specific characteristics such that each species has a range of ecological tolerance and optimum values for different environmental variables. Among the species, cosmopolitans seem to have an advantage over noncosmopolitans because they usually have high levels of tolerance and optimum ranges. This is also the case in this study (see ) where 10 of 11 cosmopolitan species occurred more frequently than the other species. Besides, cosmopolitans generally showed higher ecological optimum and tolerance values than the mean values of the species shown in . Indeed, the CCA diagram () illustrates that most species are located on the left-hand side of the diagram closer to the center while three species were placed on the right-hand side. Among the species, I. gibba and H. salina were located closer to temperature and EC at the ends. Thus, CCA analyses () suggest that Alt and EC (which may be referred to as salinity) were influential variables on species.
Among the species, a cosmopolitan species, H. salina is known to live in a wide range of aquatic bodies from brackish to freshwater bodies including coasts (Ganning Citation1971), springs, wells (Meisch Citation2000; Külköylüoğlu and Vinyard Citation2000), troughs, ephemeral ditches, and shallow water bodies. The species has a wide elevational distribution, ranging from sea level to over 1316 m (Uçak et al. Citation2014) and this may even reach up to 2500 m (unpublished data). According to Uçak et al. (Citation2014), the species displayed the highest tolerance and optimum values for salinity and DO, supporting its wide range of occurrences in different habitats. Such ability to tolerate high salinity may have historical background. For example, Mischke et al. (Citation2012) reported the species occurring in a wide salinity range from Pleistocene lake sediments in the hyperarid region of Israel and Jordan where the species did not show specific preferences for anion and/or cation dominated waters. These previous reports are actually supported by the findings of this study where H. salina revealed the highest tolerance (and optimum) values for EC. Previously, another cosmopolitan species, I. gibba, has been reported with a wide range of temperature (and EC) values (7.5 °C to 42 °C) from almost sea level (1 m) to 3194 m of elevation (Külköylüoğlu Citation2013). Most recently, this species was reported from a shallow lake (Lake Çubuk, Turkey) during the Fall season (Külköylüoğlu et al. Citation2014) where the species had the highest optimum values for turbidity, DO but lowest tolerance values for pH, redox potential, and salinity. Although these values were found within the known ranges mentioned above, some of them conflict with the findings of this work. For example, we encountered I. gibba from three different water bodies (i.e., trough, pond, and creek) with high tolerance and optimum values for EC and Tw. The species is located on the upper part of CCA diagram (), closer to the arrow of Tw, supporting its affinity for temperature. Hence, all these reports support its wide tolerance ranges to different environmental variables but further data on its ecological preferences should be addressed to clarify the situation.
There are two other cosmopolitan species (L. inopinata and H. incongruens) located close to the Tw vector on the CCA diagram. Both species are well known for their wide range of tolerance values for different environmental variables (Meisch Citation2000; Pieri et al. Citation2009). Our findings correspond to the known tolerance ranges for both species. H. incongruens was the most common species, found in all types of aquatic bodies, except lake and dam lakes, whereas L. inopinata was only found in streams and creeks. In Turkey, L. inopinata has been more frequently reported in lentic water bodies (i.e., lakes, ponds, etc.) than H. incongruens which is rarely reported from these habitats. However, when the conditions are suitable, both species can also coexist in the same community.
According to , two other cosmopolitan species (P. zenkeri and I. bradyi) are located closer to the center of the CCA diagram. This may suggest that these species are not affected by environmental variables used in this study due to their wide tolerances to those variables. Indeed, I. bradyi being the second most frequently occurring species (found in all habitats except dam lake, wetland, and irrigation canals) showed higher tolerance values for EC, Tw, pH, and Alt than P. zenkeri. Unlike I. bradyi, P. zenkeri was found in wetland, creeks, streams, and troughs. This coincides with the previous reports as underlined by Meisch (Citation2000). According to him, P. zenkeri prefers slow flowing waters with rich vegetation but sometimes occurs in lakes due to passive dispersion from streams nearby. Two other cosmopolitan species (P. olivaceus and C. neglecta) are illustrated near the arrow of DO. C. neglecta was mostly encountered from pools, springs, creeks, streams, and troughs while P. olivaceus was collected from springs, creeks, irrigation canal, and troughs. Both species have been reported from the waters with relatively wide ranges of environmental variables. For example, living adults of C. neglecta have been collected from almost anoxic (0.32 mg/L) to oxygenated waters (15.4 mg/L) when P. olivaceus was described from low (1.74 mg/L) to well oxygenated (20 mg/L) water bodies (Külköylüoğlu 2013). These wide spectra of oxygen values suggest distributional succession of both species. Accordingly, elevational distribution of both species overlaps at some levels. C. neglecta is known from 172 (Rieradevall & Roca Citation1995) to 3194 m (Li et al. Citation2010) elevation, whereas P. olivacues can be found from almost sea level to 1380 m (Roca & Baltanás Citation1993). Our measurements are found within these ranges.
The last three species (P. villosa, C. vidua, and P. fontinalis) are placed on the left bottom side of CCA diagram where species are found closer to the Alt arrow. Among the species, C. vidua and P. villosa are well known from almost sea level to 2175 and 3194 m of elevation, respectively. C. vidua displayed wide occurrences from dam lake, ponds, and streams and P. villosa was reported from two types of habitats such as troughs and ponds during this study. Detailed information about their tolerance levels can be compared with the results of Külköylüoğlu (2013). Unlike these two species, ecological information about P. fontinalis is scarce. We collected the species from ponds, springs, creeks and troughs. This species is so far known from different types of springs (Akdemir & Külköylüoğlu Citation2011) and creeks in Turkey (Dügel et al. Citation2008) where the species was reported from about 996 and 1350 m of elevation, respectively. Dügel et al. (Citation2008) reported the species from a relatively cool (19.8 °C to 23.9 °C), alkaline (pH 7.97 and 7.62), freshwater creek within the range of low (4.98 mg/L) to moderately (10.27 mg/L) oxygenated water. Nevertheless, it appears that P. fontinalis has a much more limited distribution than the other two species but it tends to prefer relatively cool water bodies related to lotic systems.
Numbers of habitat types varied among the elevational ranges. For example, there were seven different habitats with the highest species richness (15 spp.) and number of stations (22 stations) in the range of 1232–1332 m. In contrast, there was only one type of habitat (wetland) with one species in one station in the range of 626–726 m. On the other hand, regression analyses failed to show a significant relationship (p > 0.05) between number of species and 11 habitat types (no species was recorded in the wells). For example, there were six different habitat types in the ranges of 828–928 m and 1131–1,231 m but the number of species were 10 and 14 in these ranges, respectively. When we looked at the relationship between numbers of habitat types and species, we observed 15 and 14 species from 18 creeks and 35 troughs, respectively (Supplemental Table S1). The implication of these findings is that increasing numbers of sampling sites does not necessarily elevate numbers of species. Hence, the quantitative value of sampling site (i.e., numbers of sites) may not be a good indicator for explaining species distribution (and diversity) among different elevational ranges. Rather, following the community perspective, qualitative value of the habitats (i.e., suitability of habitats) may provide better results for such correlation. This view is also supported with CCA diagram ((a), Supplemental Table S2). Indeed, five species (P. villosa, I. bradyi, C. vidua, P. fontinalis, and P. zenkeri) seem to be closely related to elevation, located on the lower left-hand side of the diagram where a total of 25 sampling sites with 8 different types of habitats were listed. In contrast, 22, 10, and 16 sites were located on the upper left, upper right, and lower right of the diagram, respectively.
Although we found significant (p < 0.01, r2 = 0.81) correlation between species occurrence frequency and number of sampled sites between 549 and 1457 m, there was no significant difference among some elevational ranges. For example, 14 and 15 species collected from 22 sites each were found between the ranges 1131–1231 and 1232–1332 m, respectively (). At the same ranges, we identified almost two times more asexual (9 and 10 spp.) species than the sexual (5 and 5 spp.) species. The results of the elevational band with greatest richness partially correspond with the study of Külköylüoğlu, Sarı, Akdemir, Yavuzatmaca, and Altınbağ (Citation2012), who reported 12 and 13 species at the range of 1000–1200 and 1200–1400 m when asexual (8 spp.) species were more than sexual (5 spp.) species. The authors also underlined the occurrence of both swimming and non-swimming species in all elevational ranges. During this study, we did not find a statistical difference between the numbers of swimming (7 spp.) and non-swimming (8 spp.) species from 1231 to 1332 m but the numbers of non-swimming species were always higher than the swimming species from 828 to 1332 m. Although swimming species were found in all elevational ranges, non-swimming species were absent from 525 to 726 m. Despite the range differences between these two studies, it is interesting to see a decline in about half of the numbers of sexual species. We did not have a clear answer for why such a reduction occurred in sexual ostracods but this may be associated with the swimming ability of most of the asexual species. According to Külköylüoğlu, Sarı, Akdemir, Yavuzatmaca, and Altınbağ (Citation2012) sexual ostracods with swimming setae may have advantage over most of the asexual species in active movement. However, there are others ways of passive dispersion (e.g., birds, frogs, humans, wind, etc.) for the asexual species. Although not certain, this may imply to find asexual species in all elevational ranges studied in this work.
Finally, there can be several biotic and abiotic factors that can reduce species richness in aquatic habitats at high elevation. However, it appears that the effects of such factors do not work in the same way on ostracods. This is because ostracods display species-specific responses to different environmental factors. This study found elevation to be one of the most important factors among the factors we investigated but such a conclusion cannot be generalized beyond our study sites or study period. This is because ecosystems are large and complex, and there is rarely just one factor that affects species richness and distribution. Although our current knowledge of ostracod species is still somewhat limited in time and place, this study implies that most (if not all) of the cosmopolitan ostracod species appear to have wide geographical distribution achieved by active or passive dispersal abilities that are not limited by elevation.
Supplemental data
Supplemental data for this article can be accessed at http://dx.doi.org/10.1080/02705060.2015.1050467.
SUPPLEMENTAL_S2.doc
Download MS Word (650 KB)SUPPLEMENTAL_S1__1_.doc
Download MS Word (305 KB)Acknowledgement
We thank Dr Erik Beever (USGS Northern Rocky Mountain Science Center) for critical review and comments on the first draft of the manuscript and help with English. Also, we thank Samet Uçak for his help during fieldwork.
Disclosure statement
No potential conflict of interest was reported by the authors.
References
- Akdemir D, Külköylüoğlu O. 2011. Freshwater Ostracoda (Crustacea) of Diyarbakır Province, including a new report for Turkey. Turk J Zool. 35:671–675.
- Birks HJB, Line JM, Juggins S, Stevenson AC, Ter Braak CJF. 1990. Diatoms and pH reconstruction. Philos Trans R Soc. 327:263–278.
- Bronshtein ZS. 1988. Freshwater Ostracoda. Fauna of the USSR. Crustaceans. Vol. II, No. 1 ( Russian Translation Series, 64. New Series, 31). Rotterdam: A. A. Balkema. pp. 470.
- Dügel M, Külköylüoğlu O, Kılıç M. 2008. Species assemblages and habitat preferences of Ostracoda (Crustacea) in Lake Abant (Bolu, Turkey). Belgian J Zool. 138:50–59.
- Ganning B. 1971. On the ecology of Heterocypris salinus, H. incongruens and Cypridopsis aculeata (Crustacea: Ostracoda) from Baltic brackish-water rockpools. Marine Biol. 8:271–279.
- Gülen D, Altınsaçlı S, Kubanç C, Kılıç M. 1994. Türkiye Ostracoda (Crustacea) Faunası [The Ostracoda (Crustacea) fauna of Turkey]. Ankara: TÜBİTAK. (Unpublished project report TBAG-989).
- Guo Y, Frenzel P, Börner N, Akita LG, Zhu L. 2013. Recent Ostracoda of Taro Co (Western Tibetan Plateau). Il Naturalista Siciliano. IV, XXXVII(1): 161–162 (Abstract). 17th International Symposium on Ostracoda, Roma, Italy.
- Higuti J, Declerck SAJ, Lansac-Tôha FA, Velho LFM, Martens K. 2010. Variation in ostracod Crustacea, Ostracoda) communities in the alluvial valley of the upper Parana´ River Brazil) in relation to substrate. Hydrobiologia. 644:261–278.
- Hill JL, Curran PJ, Foody GM. 1994. the effect of sampling on the species-area curve. Global Ecol Biogeography Lett. 4:97–106.
- Hurlbert SJ. 1984. Pseudoreplication and the design of ecological field experiments. Ecol Monogr. 54:187–211.
- Juggins S. 2003. C2 user guide. Software for ecological and palaeoecological data analysis and visualization. Newcastle upon Tyne, UK: University of Newcastle.
- Külköylüoğlu O. 1998. Freshwater Ostracoda (Crustacea) and their quarterly occurrence in Şamlar Lake (Istanbul, Turkey). Limnologica. 28:229–235.
- Külköylüoğlu O. 2013. Diversity, distribution and ecology of nonmarine Ostracoda (Crustacea) in Turkey: Application of pseudorichness and cosmoecious species concepts. Recent Res Devel Ecol. 4:1–18.
- Külköylüoğlu O, Akdemir D, Yüce R. 2012. Distribution, ecological tolerance and optimum levels of freshwater Ostracoda (Crustacea) from Diyarbakır, Turkey. Limnology. 13:73–80.
- Külköylüoğlu O, Altınsaçlı S, Kubanç C. 1993. The Ostracoda (Crustacea) fauna of Küçükçekmece Lake (İstanbul) and seasonal distributions. Turk J Zool. 17:19–27.
- Külköylüoğlu O, Dügel M, Kılıç M. 2007. Ecological Requirements of Ostracoda (Crustacea) in a heavily polluted shallow lake, Lake Yeniçağa (Bolu, Turkey). Hydrobiologia. 585:119–133.
- Külköylüoğlu O, Sarı N, Akdemir D. 2012. Distribution and ecological requirements of Ostracods (Crustacea) at high elevational ranges in Northeastern Van (Turkey). Ann Limnol [Int J Limnology]. 48:39–51.
- Külköylüoğlu O, Sarı N, Akdemir D, Yavuzatmaca M, Altınbağ C. 2012. On the relationship between altitudinal changes and ostracod species reproductive mode in Ordu (Turkey). High Alt Med Biol. 13:126–137.
- Külköylüoğlu O, Sar℩ N, Dügel M, Dere Ş, Dalk℩ran N, Aygen C, Dinçer SÇ. 2014. Effects of limnoecological changes on the Ostracoda (Crustacea) community in a shallow lake (Lake Çubuk, Turkey). Limnologica. 46:99–108.
- Külköylüoğlu O, Vinyard GL. 2000. Distribution and ecology of freshwater Ostracoda (Crustacea) collected from springs of Nevada, Utah, and Oregon: A preliminary study. West North Amer Natur. 60:291–303.
- Külköylüoğlu O, Yavuzatmaca M, Akdemir D, Sarı N. 2012. Distribution and local species diversity of freshwater Ostracoda in relation to habitat in the Kahramanmaraş Province of Turkey. Int Revue Hydrobiol. 97:247–261.
- Külköylüoğlu O, Sar℩ N, Dügel M, Dere Ş, Dalk℩ran N, Aygen C, Dinçer SÇ. 2014. Effects of limnoecological changes on the Ostracoda (Crustacea) community in a shallow lake (Lake Çubuk, Turkey). Limnologica. 46:99–108.
- Laprida C, Díaz A, Ratto N. 2006. Ostracods (Crustacea) from thermal waters, southern Altiplano, Argentina. Micropaleontology. 52:177–188.
- Li X, Liu W, Zhang L, Sun Z. 2010. Distribution of Recent Ostracod species in the Lake Qinghai area in northwestern China and its ecological significance. Ecol Indicat. 10:880–890.
- Majoran S, Agrenius S, Dwyer GS. 1999. The effect of temperature on the geochemical composition of the valves of the ostracod species Krithe praetexta praetexta. Geosound. 35:93–113.
- Meisch C. 2000. Freshwater Ostracoda of Western and Central Europe. In: Schwoerbel J, Zwick P, editors. Suesswasserfauna von Mitteleuropa, Vol. 8/3. Heidelberg, Berlin: Spektrum Akademischer Verlag; pp. 522.
- Mezquita F, Boronat MD, Miracle MR. 2002. The life history of Cyclocypris ovum (Ostracoda) in a permanent karstic lake. Arch Hydrobiol. 155:687–704.
- Mezquita F, Tapia G, Roca JR. 1999. Ostracoda from springs on the eastern Iberian Peninsula: ecology, biogeography and palaeolimnological implications. Paleogeography Paleoclimatology Paleeoecology. 148:65–85.
- Mischke S, Ginat H, Al-Saqarat B, Almogi-Labin A. 2012. Ostracods from water bodies in hyperarid Israel and Jordan as habitat and water chemistry indicators. Ecol Indicat. 14:87–99.
- Nagorskaya L, Keyser D. 2005. Habitat diversity and ostracod distribution patterns in Belarus. Hydrobiologia. 538:167–178.
- Neil JV, Bell KN. 2006. Microreticulation in Cambrian Ostracoda and its relation to similar patterns in tertiary and recent Ostracoda. Lethaia. 39:31–37.
- Pieri V, Martens K, Stoch F, Rossetti G. 2009. Distribution and ecology of non-marine ostracods (Crustacea, Ostracoda) from Friuli Venezia Giulia (NE Italy). J Limnology. 68:1–15.
- Rieradevall M, Roca JR. 1995. Distribution and population dynamics of ostracodes (Crustacea, Ostracoda) in a karstic lake: Lake Banyoles (Catalonia, Spain). Hydrobiologia. 310:189–196.
- Roca JR, Baltanás A. 1993. Ecology and distribution of Ostracoda in Pyrenean springs. J Crust Biol. 13:165–174.
- Rosenfeld A. 1982. The secretion process of the ostracod carapace. In: Bate RH, Robinson E, Sheppard LM, editors. Fossil and Recent Ostracods. Chichester: Ellis Horwood; pp. 12–24.
- Rosenfeld A. Ortal R. 1983. Ostracodes as indicators of water pollution in Nahal Harod, northern Israel. In: Maddocks RF, editor. Applications of Ostracoda, Proceedings of the 8th International Symposium on Ostracoda. Texas: University of Houston; pp. 229–237.
- Schäfer BHW. 1952. Über Süßwasserostracoden aus der Türkei [About freshwater ostracods from Turkey]. Hidrobiologia, İstanbul Univ. Fen Fakültesi, Hidrobiol Araştırma Enstit. Yayın Seri B. 1:7–32.
- Shehu M, Serravalle F, Alfonso G, Moscatello S, Belmonte G. 2009. The Alpine Lake Gistova (Mount Gramos, Albania-Greece border) biodiversity of an isolated microcosm. Thalassia Salent. 32:53–62.
- Steineck PL, Maddocks RF, Coles G, Whatley R. 1990. Xylophile Ostracoda in the deep sea. In: Whatley R, Maybury C, editors. Ostracoda and Global Events. New York (NY): Chapman and Hall; pp. 307–319.
- ter Braak CJF. 1987. The analysis of vegetation-environment relationships by canonical correspondence analysis. Vegetatio. 69:69–77.
- ter Braak CJF, Barendregt JG. 1986. Weighted averaging of species indicator values: its efficiency in environmental calibration. Math Biosci. 78:57–72.
- Turpen JB, Angell RW. 1971. Aspects of moulting and calcification in the ostracod Heterocypris. Biol Bull. 140:331–338.
- Uçak S, Külköylüoğlu O, Akdemir D, Başak E. 2014. Distribution, diversity and ecological characteristics of freshwater Ostracoda (Crustacea) in shallow aquatic bodies of the Ankara region, Turkey. Wetlands. 34:309–324.
- Williams CB. 1943. Area and number of species. Nature. 152:264–267.
- Wrozyna C, Frenzel P, Steeb L, Zhu P, Schwalb A. 2009. Recent lacustrine Ostracoda and a first transfer function for palaeo-water depth estimation in Nam Co, southern Tibetan Plateau. Rev Espanola de Micropaleontol. 41:1–20.
- Yavuzatmaca M, Külköylüoğlu O, Yılmaz O. 2015. Distributional patterns of non-marine Ostracoda (Crustacea) in Adiyaman Province (Turkey). Ann Limnol [Int J Limnology]. 51:101–113.