Abstract
Alarm cues are produced by an organism when injury occurs, and serve to signal danger when detected by conspecifics or to attract other predators that may disrupt the predation event. We exposed aquatic isopods (Caecidotea intermedius) and amphipods (Crangonyx setodactylus) to chemical stimuli from macerated conspecifics to test for the presence of alarm cues in these taxa. Three variables were examined during this experiment: time spent moving, time in refuge, and time in open. Despite previous evidence for the presence of alarm cues in both taxa, we found that only amphipods responded to alarm cues by decreasing overall movement. Isopods did not display any differences in behavior following exposure to alarm cues, which may be due to degradation of the active compounds in the alarm cues due to preparation and not the absence of these cues in general.
Introduction
A wide array of signals are used by terrestrial organisms to communicate information between individuals, including visual and auditory cues. In aquatic systems, however, receiving many of these signals can be difficult due to low visibility and/or poor noise conduction in turbid water or highly complex habitats (Brönmark & Hansson Citation2000). In these cases, aquatic organisms often rely on chemical signals extensively to relay information over long distances (Goodenough et al. Citation2010). The two main types of chemical signals are pheromones and allelochemicals (see reviews in Dicke & Sabelis Citation1988; Goodenough et al. Citation2010). Pheromones are produced by an organism to signal information to members of the same species and benefits the receivers (and in some cases, the sender as well) (Dicke & Sabelis Citation1988; Goodenough et al. Citation2010). The second type of chemical signal, an allelochemical, is produced by a member of one species but is received by a member of a different species and can benefit the sender or the receiver depending upon signal type (allomone or kairomone, respectively).
One category of chemical signals that are particularly important in aquatic systems is chemical alarm cues (see review in Ferrari et al. Citation2010). Alarm cues are signals that are released by an organism that has undergone physical trauma during a predation event. These signals are then received by conspecifics or other organisms in a similar prey guild, which are then alerted to potential danger and may initiate predator avoidance or antipredator behaviors (Ferrari et al. Citation2010). Anti-predator behavior occurs when a predation event has been initiated and the prey is in eminent threat of danger (Brodie et al. Citation1991). Predator avoidance behaviors allow the prey to avoid a potential predation event by remaining outside of a predator's perceptual field (Brodie et al. Citation1991). Although a prey's chance of survival decreases with each successive stage of a predation event (Endler Citation1986), alarm cues can function at any stage and can even enhance survival of individuals after being attacked by a predator (Mathis et al. Citation1995; Chivers et al. Citation1996).
Alarm cues have been documented in many aquatic organisms, including fish, amphibians, and invertebrates (Ferrari et al. Citation2010). Although much research has been done with aquatic invertebrates, little is known about freshwater crustaceans. This is surprising because freshwater crustaceans, specifically the orders Isopoda and Amphipoda, are ecologically important due to their unique ability to cycle energy and nutrients in open waters and streams (see review by Covich et al. Citation1999). For example, they process detritus, creating nutrient-rich waste that becomes accessible to autotrophs (Covich et al. Citation1999), while also being an important prey guild for higher trophic levels (Hynes Citation1956; Macneil et al. Citation1999). Although the orders Isopoda and Amphipoda are ecologically important, the chemical ecology of these groups is not well understood. To our knowledge, only one species of isopod and two species of amphipods (out of more than approximately 20,000 total species) have been tested for the presence of these cues. We conducted two experiments to determine whether the amphipod Crangonyx setodactylus and the isopod Caecidotea intermedius possess alarm cues and utilize them to reduce predation risk.
Methods
Animal collection and maintenance
Aquatic isopods (C. intermedius) and amphipods (C. setodactylus) were collected via dip-netting from small fishless seasonal pools near Hanover, IN, USA in January of 2015. Isopods and amphipods were transported together in 19-L buckets to Hanover College. Animals were then housed in 9.5-L aquaria with four parts deionized water to one part detritus from the collection site. Each chamber was provided an aerator, conditioned to 14 °C, and kept on a 12-h light and 12-h dark cycle. Isopods and amphipods were housed together for 24–48 h before the trials were conducted. Deionized water was conditioned to 14 °C and used during all trials and in alarm cue preparation.
Experimental protocol
The alarm cue treatment was prepared by macerating either 15 isopods or amphipods and homogenizing the mixture from a single species in 30 mL of deionized water. Alarm cue solutions were then stored on ice until testing, which occurred the same day; the storage of alarm cues on ice immediately after preparation is a common practice for testing the efficacy of these compounds (e.g., Mirza & Chivers Citation2001; Bleakley et al. Citation2006; Gall & Brodie Citation2009). The control treatment consisted of deionized water, without the addition of alarm cues.
Each test chamber was a plastic container (7 × 7 × 10 cm) with three sides covered with opaque tape in order to minimize external visual stimuli. The substrate consisted of 1 cm of coarse sand that was evenly distributed on the bottom of the container. Deionized water (200 mL) was then added to the chamber. The chambers had two distinct sections that were denoted by a line drawn vertically down the middle on the remaining uncovered side of the chamber. On one randomly chosen side we placed two pieces of aquatic plant (Elodea spp.) that was 7 cm long and anchored into the sand with lead strips to provide a site for refuge; refuge has been shown to be an important factor in the predator avoidance behavior of other crustaceans (Holomuzki & Short Citation1988, Citation1990; Wisenden et al. Citation1999, Citation2009). The other side was left open.
Either an isopod (n = 40) or an amphipod (n = 40) was introduced to the center of the chamber via a plastic pipette, then 2 mL of deionized water was added. Organisms were given a 1-min acclimation period followed by a 5-min pre-stimulus observation period. During the 5-min pre-stimulus period, we recorded the amount of time the organism spent moving and how long the organism remained on either side of the test chamber. We then dispensed 2 mL of a randomly chosen treatment (control or alarm cue) into the center of the chamber 2 cm below the surface of the water. A second 1-min acclimation period was initiated and then a 5-min post-stimulus observational period began. The same behaviors were recorded as in the pre-stimulus period. Once the trials were completed, the isopod or amphipod was removed and placed in a separate container; tested isopods and amphipods were never reused. The chambers and plants were rinsed with deionized water for 30 sec and the sand was replaced with clean sand.
Statistical analysis
We compared the change in time moving (post-stimulus period minus pre-stimulus period) and the change in time spent in refuge between isopods or amphipods exposed to the control or alarm cue treatments using Student's t-tests. A Shapiro–Wilks’ normality test was conducted along with a Levene median equal variance test to ensure these data met the assumptions of normality and homoscedasticity. All assumptions were adequately met by these data. For all analyses, α = 0.05.
Results
A t-test found a significant difference between the change in time moving by amphipods exposed to alarm cues from macerated conspecifics and a control (t = −3.38, df = 39, p < 0.002, (a)). In this case, amphipods reduced movement when alarm cues were introduced as compared to the control treatment ((a)). We also found that the amphipods exposed to alarm cues stayed in shelter longer and were in open sections of the water column less than amphipods exposed to a control; however, there was no statistical difference between the treatments for either variable (refuge: t = 0.46, df = 39, p = 0.650, (b); open: t = −0.731, df = 39, p = 0.469, (c)).
Figure 1. Mean change (±SE) in activity between amphipods (a–c) or isopods (d–f) exposed to deionized water (control) or cues from macerated conspecifics (alarm). The change in activity (time moving, time in refuge, or time in open) was calculated by subtracting time in each activity during the pre-stimulus period from the time in activity during post-stimulus period.
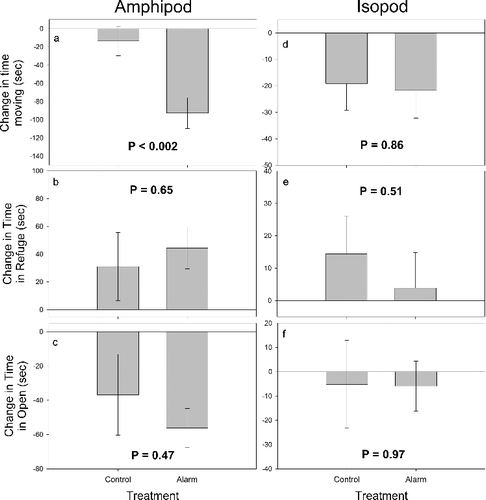
No significant differences were found between the change in time moving (t = −0.18, df = 39, p = 0.858, (d)), time spent in refuge (t = −0.66, df = 39, p = 0.513, (e)), or time in open (t = −0.037, df = 39, p = 0.97, (f)) for isopods exposed to the control or alarm cue treatments.
Discussion
The amphipod, C. setodactylus, significantly decreased overall movement when exposed to alarm cues from macerated conspecifics. Chemical communication for reproductive purposes is widespread among amphipods and isopods (Thiel Citation2011) and our results indicate that C. setodactylus possess alarm cues and respond with predator avoidance mechanisms. The recognition of alarm cues by aquatic species is crucial in predator avoidance because these cues can signal an impending attack by a predator (Ferrari et al. Citation2010). Common predator avoidance mechanisms in response to these cues in aquatic systems include reductions in activity, increased movement (i.e., fleeing), increased drift, shelter use, and increased schooling (Wooster & Sih Citation1995). These behaviors reduce the probability of a prey entering the predator's perceptual field or decreases the probability of an individual being selected from the group of prey. Chemical communication is particularly important in aquatic systems where visual acuity is often limited due to suspended sediment or dense vegetation. Nevertheless, aquatic predators have multiple sensory modalities at their disposal including visual, chemical, and lateral line information (Atema et al. Citation1988). Several studies have empirically tested whether chemical cues associated with predation risk assessment (e.g., alarm cues) enhance the survival of various prey species. For example, Mathis and Smith (Citation1993a, Citation1993b) conducted an experiment exposing predatory northern pike (Esox lucius) to fathead minnows (Pimephales promelas) and found that minnows exposed to alarm cues survived almost 40% longer relative to a control. Similarly, dragonfly naiads are less successful at capturing toad tadpoles, and northern pike are less successful at capturing trout (Salvelinus fontinalis) if the prey have been previously exposed to alarm cues from conspecifics (Hews Citation1988; Mirza & Chivers Citation2001). Specifically, in the order Amphipoda, previous work on the role of chemical alarm signals in response to predation have focused on the genus Gammarus. For example, Wisenden et al. (Citation1999) found that the amphipod Gammarus minus decreased movement and moved lower in the water column when exposed to alarm cues from damaged conspecifics. This modification in behavior was beneficial to the amphipods survival during encounters with green sunfish (Lepomis cyanellus) (Wisenden et al. Citation1999). Similarly, Gammarus lacustris exhibited antipredator responses toward cues from macerated conspecifics (Wisenden et al. Citation2009) and in a field study showed aversion to traps baited with these same cues (Wisenden et al. Citation2001).
In contrast to amphipods, isopods did not decrease movement, increase time in shelter or decrease time in open water when exposed to alarm cues. This finding is surprising due to the prevalence of alarm cues in aquatic taxa, as well as a recent study conducted in our lab, which showed a strong predator avoidance response toward alarm cues by this same species of isopod (Spivey et al. Citation2015). Although these studies are similar, several differences may explain the discrepancy between our results. Although unlikely to have an effect, the test chambers in the two studies differ dramatically and that used by Spivey et al. (Citation2015) may have given a more precise measure of overall activity; the chamber was circular and only allowed movement along a single plane. More likely, the difference in alarm cue preparation between the two studies may account for the contrasting results. The alarm cues in the study by Spivey et al. (Citation2015) were frozen immediately after preparation and were individually thawed prior to each trial. In our study, the alarm cues were prepared at the beginning of each day and were not frozen. Thus, the cues were prepared and left (on ice) for as much as 6 h prior to testing. Alarm cues are believed to be proteinaceous in some species and these cues do become biologically inactive over a period of time (Dodson Citation1988; Wisenden et al. Citation2009). In the study by Wisenden et al. (Citation2009), the active time of alarm cues from fathead minnows (P. promelas) and an amphipod (G. lacustris) was approximately 3 h for both species (at 18 °C). Although our cues were stored on ice prior to testing, it is possible that alarm cues from the species of isopod used in this study also degrade overtime and may do so at a faster rate than that observed for other species. Regardless, additional research is necessary to determine whether isopods possess alarm cues and if degradation of the cues over time can account for the absence of a response in our study.
Alarm cues are ecologically important compounds due to the variety of contexts in which prey species may use them to detect predatory threats (see review in Ferrari et al. Citation2010). For example, in addition to serving as direct indicators of a recent predatory attack (Mathis & Smith Citation1993a, Citation1993b), some species can detect these compounds in the diet of their predators (Keefe Citation1992; Jacobsen & Stabell Citation2004) or are only activated after passing through the predator's digestive system (Stabell et al. Citation2003). This alarm cue labeling should provide reliable information to potential prey by directly indicating which individual predators are actively foraging on that prey guild. In some cases, the release of alarm cues may provide direct benefits by attracting additional predators that disrupt the event (Mathis et al. Citation1995; Chivers et al. Citation1996; Wisenden & Thiel Citation2002) or potentially defending against pathogens and parasites (Chivers et al. Citation2007). Although additional research is necessary to understand which of these scenarios alarm cues function in for amphipods, our findings support the idea of alarm cue recognition in a newly tested genus of amphipod, yet fail to confirm this in the isopod C. intermedius. With increasing knowledge of the presence of alarm cues in aquatic species, the role of chemical communication in the predator–prey interactions of these species is being elucidated.
Acknowledgements
The authors thank the Department of Biology and Hanover College for funding and support. We also thank the 2015 Animal Behavior class at Hanover College for helping to facilitate this work.
Disclosure statement
No potential conflict of interest was reported by the authors.
Additional information
Funding
References
- Atema J, Fay RR, Popper AN, Tavolga WN. 1988. Sensory biology of aquatic animals. New York (NY): Springer-Verlag.
- Bleakley BH, Martell CM, Brodie ED, III. 2006. Variation in anti-predator behavior among five strains of inbred guppies, Poecilia reticulata. Behav Genet. 36:783–791.
- Brodie ED, Jr., Formanowicz DR, Jr., Brodie ED, III. 1991. Predator avoidance and antipredator mechanisms: distinct pathways to survival. Ethol Ecol Evol. 3:73–77.
- Brönmark C, Hansson L. 2000. Chemical communication in aquatic systems: an introduction. Oikos. 88:103–109.
- Chivers DP, Brown GE, Smith RJF. 1996. The evolution of chemical alarm signals: attracting predators benefits alarm signal senders. Am Nat. 148:649–659.
- Chivers DP, Wisenden BD, Hindman CJ, Michalak T, Kusch RC, Kaminskyj SW, Jack KL, Ferrari MCO, Pollock RJ, Halbgewachs CF, et al. 2007. Epidermal ‘alarm substance’ cells of fishes maintained by non-alarm functions: possible defence against pathogens, parasites and UVB radiation. Proc R Soc B. 274:2611–2619.
- Covich AP, Palmer MA, Crowl TA. 1999. The role of benthic invertebrate species in freshwater ecosystems: zoobenthic species influence energy flows and nutrient cycling. Bioscience. 49:119–127.
- Dicke M, Sabelis MW. 1988. Infochemical terminology: based on a cost–benefit analysis rather than origin of compounds? Funct Ecol. 2:131–139.
- Dodson S. 1988. The ecological role of chemical stimuli for the zooplankton: predator‐avoidance behavior in Daphnia. Limnol Oceanogr. 33:1431–1439.
- Endler JA. 1986. Defense against predators. In: Feder ME, Lauder GV, editors. Predator–prey relationships. Chicago: University of Chicago Press; p. 109–134.
- Ferrari MCO, Wisenden BD, Chivers DP. 2010. Chemical ecology of predator–prey interactions in aquatic ecosystems: a review and prospectus. Can J Zool. 88:698–724.
- Gall BG, Brodie ED, Jr. 2009. Behavioral avoidance of injured conspecific and predatory chemical stimuli by larvae of the aquatic caddisfly Hesperophylax occidentalis. Can J Zool. 87:1009–1015.
- Goodenough J, McGuire B, Jakob E. 2010. Perspectives on animal behavior. Hoboken (NJ): Wiley.
- Hews DK. 1988. Alarm response in larval western toads, Bufo boreas: release of larval chemicals by a natural predator and its effect on predator capture efficiency. Anim Behav. 36:125–133.
- Holomuzki JR, Short TM. 1988. Habitat use and fish avoidance behaviors by the stream-dwelling isopod Lirceus fontinalis. Oikos. 52:79–86.
- Holomuzki JR, Short TM. 1990. Ontogenetic shifts in habitat use and activity in a stream-dwelling isopod. Ecography. 13:300–307.
- Hynes HBN. 1956. British freshwater shrimps. New Biol. 21:25–42.
- Jacobsen HP, Stabell OB. 2004. Antipredator behaviour mediated by chemical cues: the role of conspecific alarm signalling and predator labelling in the avoidance response of a marine gastropod. Oikos. 104:43–50.
- Keefe ML. 1992. Chemically mediated avoidance behavior in wild brook trout, Salvelinus fontinalis: the response to familiar and unfamiliar predaceous fishes and the influence of fish diet. Can J Zool. 70:288–292.
- Macneil C, Dick JTA, Elwood RW. 1999. The dynamics of predation on Gammarus spp. (Crustacea: Amphipoda). Biol Rev. 74:375–395.
- Mathis A, Chivers DP, Smith RJF. 1995. Chemical alarm signals: predator deterrents or predator attractants? Am Nat. 145:994–1005.
- Mathis A, Smith RJF. 1993a. Chemical alarm signals increase the survival time of fathead minnows (Pimephales promelas) during encounters with northern pike (Esox lucius). Behav Ecol. 4:260–265.
- Mathis A, Smith RJF. 1993b. Intraspecific and cross-superorder responses to chemical alarm signals by brook stickleback. Ecology. 74:2395–2404.
- Mirza RS, Chivers DP. 2001. Chemical alarm signals enhance survival of Brook Charr (Salvelinus fontinalis) during encounters with predator chain pickerel (Esox niger). Ethology. 107:989–1005.
- Spivey KL, Chapman TL, Schmitz AL, Bast DE, Smith ALB, Gall BG. 2015. The alarm cue obstruction hypothesis: isopods respond to alarm cues, but do not respond to dietary chemical cues from predatory bluegill. Behaviour. 152:167–179.
- Stabell OB, Ogbebo F, Primicerio R. 2003. Inducible defenses in Daphnia depend on latent alarm signals from conspecific prey activated in predators. Chem Senses. 28:141–153.
- Thiel M. 2011. Chemical communication in peracarid crustaceans. Chemical communication in crustaceans. New York (NY): Springer; p. 199–218.
- Wisenden BD, Cline A, Sparkes TC. 1999. Survival benefit to antipredator behavior in the amphipod Gammarus minus (Crustacea: Amphipoda) in response to injury released chemical cues from conspecifics and heterospecifics. Ethology. 105:407–414.
- Wisenden BD, Pohlman SG, Watkin EE. 2001. Avoidance of conspecific injury-released chemical cues by free-ranging Gammarus lacustris (Crustacea: Amphipoda). J Chem Ecol. 27:1249–1258.
- Wisenden BD, Rugg ML, Korpi NL, Fuselier LC. 2009. Lab and field estimates of active time of chemical alarm cues of a cyprinid fish and an amphipod crustacean. Behaviour. 146:1423–1442.
- Wisenden BD, Thiel TA. 2002. Field verification of predator attraction to minnow alarm substance. J Chem Ecol. 28:433–438.
- Wooster D, Sih A. 1995. A review of the drift and activity responses of stream prey to predator presence. Oikos. 73:3–8.