Abstract
Understanding the effects of disturbances on aquatic biota is important for management of the world's rivers. Riverine deltas are among the most biologically productive ecosystems, yet the ecological effects of floods in deltas are poorly understood. Therefore, the goal of this study was to examine impacts of an historic flood in the Missouri River basin in 2011 on fishes and aquatic habitats in the Lewis and Clark Delta, located in South Dakota and Nebraska, USA. Fish community structural indices declined in the six years preceding the flood, with species richness and diversity (Fisher's α) decreasing from 25 to 22 and 4.56 to 3.48, respectively. However, the fish community exhibited short-term resistance to the flood as both metrics were similar to pre-flood levels after the disturbance in 2012. Evenness (J′) declined from 0.88 to 0.73 before the flood but increased to 0.84 after the disturbance. The majority of species exhibited greater relative abundance after the flood regardless of age class (i.e., juvenile, adult), morphology (i.e., small-bodied, large-bodied), introduction history (i.e., introduced, native), or recreational importance (i.e., sport fish, non-recreational). However, the flood reduced relative abundance of juvenile freshwater drum (Aplodinotus grunniens) and white crappie (Pomoxis annularis) and did not affect the three small-bodied species that were studied, including emerald shiner (Notropis atherinoides), red shiner (Cyprinella lutrensis), and spotfin shiner (Cyprinella spiloptera). The flood decreased side channel and backwater frequency and width/area but increased sandbar abundance. Physical alterations evidently had minimal effects on structural indices of the fish community. However, an overall increase in relative abundance across species suggests that interspersed fluvial and slackwater habitats in the delta provided refuge from floodwaters during the disturbance. Maintaining habitat connectivity in deltas during and after floods is particularly important for fisheries conservation. Illustrating the ecological effects and implications of a major flood, this study contributes to the nascent field of delta ecology.
Introduction
Floods cause environmental variability and structure biotic communities in large floodplain rivers (Poff et al. Citation1997; Ward et al. Citation1999), yet opportunities to examine their ecological effects with pre- and post-disturbance data are scarce (Bischoff & Wolter Citation2001). Floods connect rivers with floodplains and supply nutrients and organic matter to aquatic--terrestrial transition zones, stimulating biological productivity and habitat heterogeneity (Junk et al. Citation1989; Ward & Stanford Citation1995; Tockner et al. Citation2000). River–floodplain connectivity is especially important in impounded rivers, where sediment imbalances associated with dam operation and tributary inputs cause sedimentary deltas to form (Graf et al. Citation2010). Deltas are among the most biologically productive, ecologically diverse ecosystems in the world (Long & Pavelsky Citation2013), yet they remain understudied (Volke et al. Citation2015). Basic information on fish communities, aquatic habitats, and ecological impacts of floods is necessary to advance delta management.
Floods induce environmental changes (e.g., greater habitat and resource availability) that can stimulate fish productivity and increase species abundance, richness, evenness, and diversity compared to stable flow regimes (Junk et al. Citation1989). However, structural indices of some fish communities may not be affected by floods, as in the upper Amazon River (Freitas et al. Citation2010). The effects of floods are mediated by the magnitude, frequency, duration, timing, and rate of change of water levels (Richter et al. Citation1996). In addition, flood impacts may vary according to fish age, morphology, physiology (e.g., turbidity tolerance, swimming strength), behavior (e.g., movement, habitat use), and community composition (e.g., species abundance, diversity) (Bischoff & Wolter Citation2001; Kano et al. Citation2011). For instance, a flood in the Lower Murray River decreased the abundance of small-bodied species (e.g., flat-head gudgeon [Philypnodon macrostomus], unspeckled hardyhead [Craterocephalus stercusmuscarum fulvus]) by removing submerged macrophytes but increased the abundance of large-bodied fishes (e.g., common carp [Cyprinus carpio], golden perch [Macquaria ambigua ambigua]) by promoting recruitment (Bice et al. Citation2014). Floods may also exert differential effects on introduced and native fishes. Although native fishes may be more adapted to flow regimes in their indigenous range (Minckley & Meffe Citation1987; Gido et al. Citation1997), both native and introduced species may benefit from river–floodplain connectivity and become more abundant after floods (Stoffels et al. Citation2014; Vilizzi et al. Citation2014). In some rivers, floods increase reproduction, recruitment, and population growth of introduced fishes to a greater extent than native species (Scheerer Citation2002; Barko et al. Citation2006; Sommer et al. Citation2014). Finally, from a fisheries management standpoint, it is important to distinguish flood effects on sport fishes and non-recreational species so that natural resource agencies can implement management strategies to increase sport fish production (Sammons et al. Citation2001).
Effects of floods on fishes and aquatic habitats in deltas are poorly understood. Knowledge of short-term (i.e., <1 year) flood impacts is particularly sparse (Jurajda et al. Citation2006) as most studies of this scale are conducted in small coldwater streams (Gerking Citation1950; Lobon-Cerviá Citation1996) and medium-sized rivers (Ross & Baker Citation1983; Matthews Citation1986). Floods increased the probability of fish entrainment into the interior Sacramento–San Joaquin River delta (Perry et al. Citation2015) and caused sediment erosion and associated physicochemical changes (e.g., low water transparency and dissolved oxygen) that reduced fish production and abundance in the Niger River delta (Akankali & Jamabo Citation2012). In contrast, floods increased fish reproduction, recruitment, and abundance in the Okavango River delta (Siziba et al. Citation2011). The Missouri River, USA, is an ideal system for studying the ecological effects of floods in deltas. Flowing 3767 km across one-sixth of the continental land area of the United States, it is the longest river in the country (Volke et al. Citation2015). The Missouri River reservoir system is the largest water storage system in North America, composed of six mainstem impoundments with a combined storage capacity of 90.5 km3 (US Army Corps of Engineers Citation2006). Over the last 40 years, sedimentary deltas have formed where the mainstem river flows into reservoir pools (mainstem deltas), at the mouths of tributaries (tributary deltas), and where tributaries enter near the upstream end of reservoirs (tributary–mainstem combination deltas; Volke et al. Citation2015). Deltas contain warm, turbid water and manifold habitats (e.g., side channels, backwaters, embayments) that simulate shallow-water environments of the historic riverscape (Graeb et al. Citation2009). In turn, habitat complexity increases fish abundance and diversity in deltas compared to riverine or reservoir environments (Kaemingk et al. Citation2007; Spindler et al. Citation2009, Citation2012).
Despite their ecological importance, deltas decrease reservoir storage capacity and impede waterfront access in Missouri River impoundments. The US Army Corps of Engineers is currently examining the feasibility of sediment management strategies (e.g., water drawdown and sediment flushing, channelization, dredging, tributary diversion, US Army Corps of Engineers Citation2011), but projecting the ecological effects of these actions is difficult. A historic flood occurred in 2011 and offered a rare opportunity to study short-term ecological impacts of high discharge. Late-melting snow in the Rocky Mountains and heavy rainfall in the Great Plains caused record flooding from May to August. Runoff totaled 75.2 billion m3 and exceeded the system capacity by >20% above Sioux City, IA, USA (US Army Corps of Engineers Citation2012). Discharge through all six mainstem reservoir dams was historically high. Preliminary research indicates substantial socioeconomic effects and physical alterations to reservoirs, including bed degradation, thalweg migration, and sandbar development and expansion (Cowman Citation2012). However, the ecological effects of the flood in deltas have not been investigated.
The purpose of this study was to evaluate short-term effects of the 2011 flood on fishes and aquatic habitats in a Missouri River delta to promote science-based ecosystem management. Our first objective was to assess how the flood impacted fish species richness, evenness, and diversity. We hypothesized that the flood would increase evenness and diversity without affecting richness (Freitas et al. Citation2010; Gomes et al. Citation2012; Steffensen et al. Citation2014). Our second objective was to evaluate how the flood affected relative abundance of fishes as categorized by age class (i.e., juvenile, adult), morphology (i.e., small-bodied, large-bodied), introduction history (i.e., introduced, native), and recreational importance (i.e., sport fish, non-recreational species). We predicted that trends in juvenile relative abundance would vary among species, whereas adult relative abundance would increase for all species. We expected that small-bodied species (i.e., typical adult length < 100 mm TL) would become less abundant and large-bodied fishes (i.e., not small-bodied) more abundant after the flood (Bice et al. Citation2014). Moreover, we hypothesized that relative abundance of both introduced and native species and sport and non-recreational fishes would increase after the flood (Stoffels et al. Citation2014; Vilizzi et al. Citation2014). Our third objective was to examine the effects of the flood on aquatic habitats. We hypothesized that the flood would increase sandbar abundance but decrease side channel frequency and width and backwater frequency and area (Cowman Citation2012).
Methods
Study area
Lewis and Clark Lake is the smallest and southernmost mainstem Missouri River impoundment (). Formed after completion of Gavins Point Dam in 1955, it has a surface area of 105 km2, maximum depth of 16.7 m, and mean depth of 5.0 m (Wickstrom Citation2004; US Army Corps of Engineers Citation2006). Approximately 70 km of riverine habitat extend upstream from the reservoir to Fort Randall Dam, which impounds Lake Francis Case.
Figure 1. Missouri River basin and location of the Lewis and Clark Delta along the South Dakota–Nebraska border, USA. The upstream end of the Delta is located near its confluence with the Niobrara River (576907 N, 4734800 E). The downstream end of the Delta is located where the reservoir portion of Lewis and Clark Lake begins (594446 E, 4743533 N).
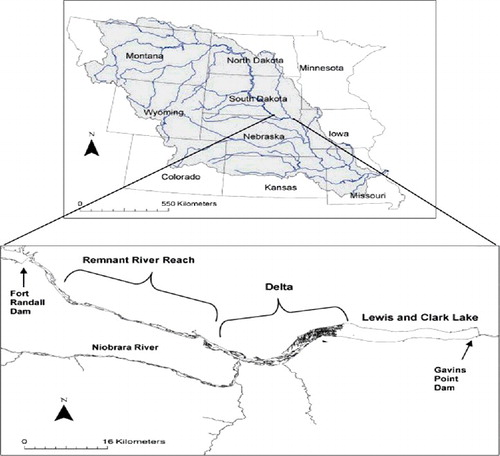
The Lewis and Clark Delta (hereafter Delta) is a tributary–mainstem combination delta (Volke et al. Citation2015) that extends 34 km above the reservoir portion of Lewis and Clark Lake (). The Missouri National Recreation River, a remnant reach, encompasses the remaining 36 km to Fort Randall Dam (Graeb et al. Citation2009). The Delta was formed by sediment deposition from the Niobrara River, which annually delivers 2.6 million metric tons of sediment to Lewis and Clark Lake (US Army Corps of Engineers Citation2001). The Delta enlarges by 105 m (Elliott & Jacobson Citation2006) and loses 2400 acre-feet of water per year (US Army Corps of Engineers Citation2011), making it the most visible and most studied of the Missouri River deltas (Coker et al. Citation2009; National Research Council Citation2011; US Army Corps of Engineers Citation2013). It is characterized by diverse habitats (i.e., braided side channels, backwaters, embayments, shallow pools near sandbars), warm temperatures, and high turbidity. During the 2011 flood, gage height in the Delta was >4.2 m, the highest on record since 1980 ().
Fish sampling
Juvenile large-bodied species were collected during pre-flood (i.e., 2004–2010), flood (i.e., 2011), and post-flood (i.e., 2012) periods in summer (mid-July) and late summer (late August/early September) using modified mini-fyke nets (MFNs, 1.2 m × 0.6 m frames, 3.2 mm bar mesh). During each season, eight nets were deployed for one net night at each of 10 standardized Delta locations representing different habitats (i.e., braided side channels, backwaters, embayments). Catch data were obtained from the United States Fish and Wildlife Service (USFWS, unpublished data). Adult large-bodied fishes and small-bodied species were collected during pre-flood (i.e., 2005, 2008, 2009) and post-flood (i.e., 2012) periods in summer (late May–July), late summer (late July–early August), and autumn (late September–early October) using boat-mounted electrofishing (EF, Coffelt VVP-15 control unit; C-phase, pulsed-DC current). During each season, 10-minute EF runs were performed at each of 12 standardized locations representing different habitats (i.e., braided side channels, backwaters, embayments). Pre-flood data were obtained from previous studies in the Delta, including 2005 data (Kaemingk et al. Citation2007) and 2008–2009 data (Schreck Citation2010). Post-flood data were collected for this study. All pre-flood and post-flood MFN and EF data were collected in normal flow conditions, whereas flood MFN data were obtained under elevated discharge. Sampling efficiency was not affected by the flood as MFNs were set in shallow water with low current velocity where fishes were effectively captured.
Aquatic habitat analysis
Changes in Delta aquatic habitats resulting from the flood were evaluated using Landsat landcover geodatabases provided by the US Army Corps of Engineers. Aerial photographs were taken during months with comparable gage height (∼4.5 m) before (June 2010) and after (October 2011) the flood and displayed into ARCMap 10.1 (Environmental Systems Research Institute, Redlands, CA, USA). A point shapefile containing 30 equidistantly spaced transect lines (perpendicular to the main channel) between the Niobrara River confluence and the downstream end of the Delta was overlaid onto each of the images. Main channel width and the largest backwater area were measured along each transect. In addition, side channels, backwaters, and sandbars were enumerated because Delta fishes use these habitats for spawning, rearing, and foraging (Kaemingk et al. Citation2007; Spindler et al. Citation2009, Citation2012). Assessing the effects of the flood on these habitats may provide insight for understanding fish community trends.
Statistical analysis
Univariate and multivariate statistical methods were used to evaluate the effects of the flood on fishes and aquatic habitats. Pre-flood (i.e., 2005, 2008, 2009) and post-flood (i.e., 2012) EF data were combined among seasons within years to assess annual trends in structural indices like species richness, evenness (Shannon's Index, J′), and diversity (Fisher's α) of adult large-bodied fishes and small-bodied species. Although richness reflects diversity, it is affected by sample size (Preston Citation1962) and may be biased by incidental species. Thus, the entire species pool was condensed to common species, those that comprised >1% of the total sample at all locations, to remove the effects of rare species and species difficult to collect. Because sample sizes differed among sampling years, rarefaction was performed to compare species richness over time (Kwak & Peterson Citation2007). Evenness was calculated aswhere S is the number of species and pi is the proportion of the total sample represented by species i. Fisher's α is unaffected by sample size and effectively represents the species of intermediate abundance (Kempton & Taylor Citation1974), making it a more rigorous measure of species diversity than richness. Fisher's α was calculated as
where x is the iterative solution of S/N = (1 − x)/x[−ln(1 − x)] and N is the number of individuals. Temporal trends in structural indices were evaluated using a bootstrapping procedure in program PAST (PAleontological STatistics) analogous to a two-sample t-test. One-way analysis of variance (ANOVA) was used to compare pre-flood and post-flood mean habitat frequencies (e.g., side channels, backwaters, sandbars), main channel width, and the largest backwater area pooled across the 30 transects.
Relative abundance of common species was calculated as catch per unit effort (CPUE) based on seasonal MFN (i.e., log10[number/net night + 1]) and EF (i.e., log10[number/hr + 1]) data. Species-specific seasonal values were pooled within years and combined by time period (i.e., pre-flood, flood, post-flood). Seasonal CPUE measurements permit assessment of intra-annual changes in relative abundance valuable for short-term studies. However, with a relatively long-term data set (i.e., 2004–2012), our objective was to evaluate how the flood affected CPUE as measured on an annual basis before, during, and after the disturbance. Species were categorized into groups based on age class (i.e., juvenile, adult), morphology (i.e., large-bodied, small-bodied), introduction history (i.e., introduced, native), and recreational importance (i.e., sport fish, non-recreational species) to evaluate the effects of the flood on CPUE of different community segments: juvenile introduced, juvenile native, juvenile sport, adult introduced, adult native, adult sport, and small-bodied (Schreck Citation2010). Large-bodied fishes were those with typical adult lengths ≥ 100 mm TL, whereas small-bodied fishes were those with typical adult lengths < 100 mm TL (Bice et al. Citation2014). All species that were not sport fishes (i.e., introduced, native, small-bodied) were considered non-recreational. The effects of the flood on multivariate community segments were evaluated using nonmetric multidimensional scaling (NMS) with square-root-transformed Bray–Curtis distance. Relative abundance of juvenile large-bodied species was compared among pre-flood (2004–2010), flood (2011), and post-flood (2012) periods, whereas CPUE of adult large-bodied fishes and small-bodied species was compared between pre-flood (2005, 2008, 2009) and post-flood (2012) periods. The statistical significance of NMS ordinations was assessed using pseudo-F (F value by permutation; pF) and p values (α = 0.05) from permutational multivariate analyses of variance (PERMANOVA, N = 1000 permutations) performed in PRIMER (PRIMER-E Ltd, Plymouth, United Kingdom; Clarke & Gorley Citation2006). Individual species driving significant ordinations were identified by comparing species-specific CPUE among or between time periods using one-way ANOVAs with post hoc Tukey's Honestly Significant Difference tests (α = 0.05).
Results
Structural indices
A total of 7352 fishes representing 23 common species were collected in the Delta from 2004 to 2012 before, during, and after the flood (). Rarefied species richness decreased from 2005 (25) to 2008 (15) but was unaffected by the flood, remaining stable from 2009 (22) to 2012 (21, ). Evenness decreased from 2005 (0.88) to 2008 (0.80) and 2009 (0.73) but increased after the flood in 2012 (0.84). Diversity declined from 2005 (4.56) to 2008 (2.17), increased in 2009 (3.48), and declined slightly in 2012 (3.27).
Table 1. Numbers of introduced, native, sport, and small-bodied fishes collected from 2004 to 2012 in the Lewis and Clark Delta.
Table 2. Structural indices (species richness [S], evenness [J′], diversity [Fisher's α]) of common fishes collected during pre-flood (2005, 2008, 2009) and post-flood (2012) periods in the Lewis and Clark Delta.
Relative abundance
The effects of the flood on relative abundance of juvenile large-bodied fishes varied among community segments and species. Relative abundance of introduced fishes was greatest after the flood when common carp (C. carpio) CPUE increased (F2, 69 = 20.22, p < 0.01; ). Native fish relative abundance varied among time periods (PERMANOVA, pF2, 69 = 10.78, p < 0.01) as CPUE declined during the flood for freshwater drum (Aplodinotus grunniens) (ANOVA, F2,69 = 4.43, p < 0.01) and increased after the disturbance for river carpsucker (Carpiodes carpio) (F2,69 = 50.78, p < 0.01). Relative abundance of sport fishes also differed among time periods (PERMANOVA, pF2,69 = 4.02, p < 0.01). CPUE increased during the flood for black crappie (Pomoxis nigromaculatus, ANOVA: F2,69 = 19.70, p < 0.01) and after the disturbance for bluegill (Lepomis macrochirus, F2,69 = 12.53, p < 0.01; ). In contrast, relative abundance of smallmouth bass (Micropterus dolomieu, F2,69 = 5.48, p < 0.01) and white crappie (Pomoxis annularis, F2,69 = 6.08, p < 0.01) decreased during and after the flood, respectively.
Figure 3. Nonmetric multidimensional scaling (NMS) ordinations of mean catch per unit effort (CPUE) for juvenile native and sport fishes during pre-flood (2004–2010), flood (2011), and post-flood (2012) periods in the Lewis and Clark Delta, South Dakota–Nebraska, USA. Multivariate heterogeneity is explained by changes in CPUE of individual species (bottom panel). Different letters indicate significant differences in mean CPUE among time periods for particular species. CAP = common carp; FRD = freshwater drum; RIC = river carpsucker; BGL = bluegill; BLC = black crappie; SMB = smallmouth bass; WHC = white crappie.
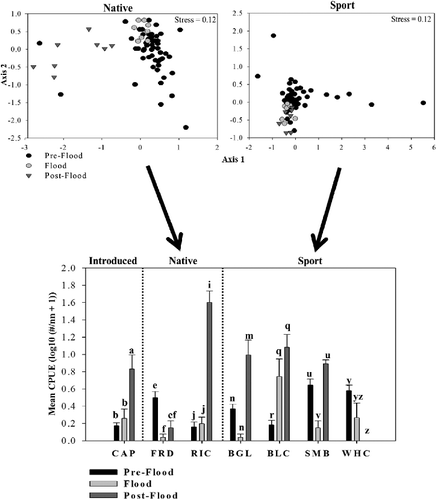
Relative abundance increased after the flood for adult large-bodied fishes and remained stable for small-bodied species. Post-flood CPUE of introduced fishes was greater than pre-flood CPUE as common carp relative abundance increased after the disturbance (F1,169 = 18.22, p < 0.01; ). Native fish CPUE varied between time periods (PERMANOVA, pF1,169 = 12.34, p < 0.01) as relative abundance of freshwater drum (ANOVA, F1,169 = 59.59, p < 0.01), river carpsucker (F1,169 = 17.40, p < 0.01), and shorthead redhorse (Moxostoma macrolepidotum) (F1,169 = 15.66, p < 0.01) increased after the flood (). Relative abundance of sport fishes also differed between time periods (PERMANOVA, pF1,169 = 9.05, p < 0.01) as CPUE increased after the flood for bluegill (ANOVA, F1,174 = 7.00, p < 0.01), channel catfish (Ictalurus punctatus, F1,169 = 11.37, p < 0.01), largemouth bass (Micropterus salmoides, F1,169 = 17.36, p < 0.01), and sauger (Sander canadensis, F1,169 = 5.58, p = 0.02). In contrast, relative abundance was consistent between time periods for small-bodied fishes (PERMANOVA: pF1,169 = 1.40, p = 0.23), including emerald shiner (Notropis atherinoides) (F1,169 = 1.57, p = 0.22), red shiner (Cyprinella lutrensis) (F1,169 = 0.05, p = 0.82), and spotfin shiner (Cyprinella spiloptera) (F1,169 = 1.98, p = 0.16).
Figure 4. Nonmetric multidimensional scaling (NMS) ordinations of mean catch per unit effort (CPUE) for adult native and sport fishes and small-bodied species during pre-flood (2004–2010), flood (2011), and post-flood (2012) periods in the Lewis and Clark Delta, South Dakota–Nebraska, USA. Multivariate heterogeneity is explained by changes in CPUE of individual species (bottom panel). Different letters indicate significant differences in mean CPUE between time periods for particular species. CAP = common carp; FRD = freshwater drum; RIC = river carpsucker; SHR = shorthead redhorse; BGL = bluegill; CCF = channel catfish; LMB = largemouth bass; SAR = sauger; EMS = emerald shiner; RES = red shiner; SFS = spotfin shiner.
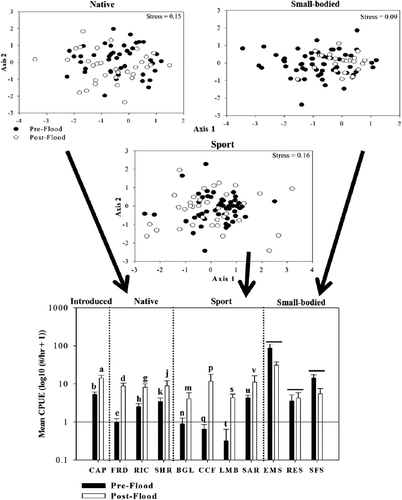
Aquatic habitats
The mean number of side channels per transect declined from 3.50 ± 0.26 (1 SE) before the flood to 2.63 ± 0.19 after the disturbance (ANOVA, F1,59 = 7.00, p = 0.01; ). Similarly, the mean number of backwaters per transect decreased from 1.93 ± 0.28 before the disturbance to 1.03 ± 0.14 afterwards (F1,59 = 8.30, p = 0.01). In contrast, the mean number of sandbars per transect increased from 0.03 ± 0.03 before the flood to 1.40 ± 0.18 after the disturbance (F1,59 = 28.02, p < 0.01). Main channel width decreased for a majority of transects (76.67%, n = 23) but was consistent as a mean across transects before (550 m) and after (489 m) the flood (F1,59 = 0.61, p = 0.44). The mean largest backwater area was also consistent (F1,59 = 0.35, p = 0.56) before (225,200 m2) and after (186,700 m2) the flood.
Figure 5. Pre-flood (June 2010) and post-flood (October 2011) frequency of side channels, backwaters, and sandbars in the Lewis and Clark Delta between the Niobrara River confluence and the downstream limit of the Delta in Springfield, SD. Habitat features were enumerated on 30 equidistantly spaced transects (perpendicular to the main channel) between the Niobrara River confluence and the downstream end of the Delta in ARCMap 10.1 using aerial photographs taken during months with comparable gage height (∼4.5 m).
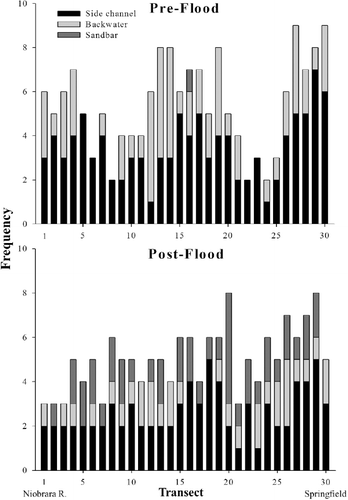
Discussion
Sedimentary deltas have remarkable biological productivity and species diversity, yet the effects of floods on fish communities and aquatic habitats in these ecosystems have not been thoroughly studied (Long & Pavelsky Citation2013; Volke et al. Citation2015). The Lewis and Clark Delta fish community exhibited short-term structural resistance to the flood as species richness and diversity were unaffected by the disturbance (Connell & Sousa Citation1983), reinforcing previous research in rivers and streams (Lojkasek et al. Citation2005; Franssen et al. Citation2006; Jurajda et al. Citation2006; Pires et al. Citation2008). Missouri River fishes are well adapted to thrive in a system that flooded frequently before it was impounded (Pflieger Citation1971; Erickson et al. Citation2008). Many species (e.g., channel catfish, river carpsucker, freshwater drum, bigmouth buffalo [Ictiobus cyprinellus], smallmouth buffalo [Ictiobus bubalus], paddlefish [Polyodon spathula]) regularly use floodplains and possess ecomorphological features (e.g., inferior mouth position, sickle-shaped or enlarged pectoral fins, reduced eyes, well-developed electrosensory and chemosensory organs) to live benthically in a swift, turbid river (Galat et al. Citation2005). These adaptations enable persistence in high flows and permitted the Delta fish community to be resistant to the 2011 flood, withstanding changes to species richness and diversity (Connell & Sousa Citation1983; Bischoff & Wolter Citation2001; DeBoer et al. Citation2011). In addition, evenness increased after the flood as species-specific abundances became more proportionally similar, supporting a concurrent Missouri River study in Nebraska, USA (Steffensen et al. Citation2014) and research in the upper Parana River (Gomes et al. Citation2012). We acknowledge that our study was limited spatially (one delta) and temporally (one year of post-flood fish community data) and recommend that future researchers assess long-term flood impacts in multiple deltas. However, our investigation provided novel information regarding short-term ecological effects of high discharge in these ecosystems (Jurajda et al. Citation2006; Volke et al. Citation2015).
The flood pulse concept postulates that fish abundance will increase after floods as river–floodplain connectivity augments habitat and resource availability (Junk et al. Citation1989). Our results supported this prediction and previous flood research (Jurajda et al. Citation2006; Zampatti & Leigh Citation2013; Stoffels et al. Citation2014) as relative abundance of most species increased after the flood. However, the flood had distinct effects on different segments of the fish community. Adult fishes were more abundant after the flood regardless of species, whereas juvenile CPUE decreased for freshwater drum and smallmouth bass during the flood and white crappie after the disturbance, supporting our hypothesis. Floodplain inundation likely promoted movement and provided favorable spawning and foraging conditions for adults (Steffensen et al. Citation2014), yet effects on juveniles varied in a species-specific manner.
As pelagic spawners, freshwater drum deposit eggs that float near the surface of the water (Becker Citation1983). It has been hypothesized that flood-induced egg drift causes hatching to occur primarily in productive backwaters located downstream from spawning areas (Koel & Sparks Citation2002). Freshwater drum spawn in the Delta from June to July (Swedberg & Walburg Citation1970), a time period overlapped by the 2011 flood, thus egg drift may have been a mechanism for low juvenile CPUE during the disturbance. Alternatively, the flood may have directly impaired reproduction and/or recruitment (Bednarski et al. Citation2008). For smallmouth bass, effects of high discharges on recruitment are well understood and nearly universally negative (Smith et al. Citation2005). Floods scour nests (Montgomery et al. Citation1980; Lukas & Orth Citation1995), displace fry (Harvey Citation1987; Simonson & Swensen Citation1990; Sabo & Orth Citation1994), and decrease availability of shallow pools (Aadland Citation1993). These effects reduce recruitment when floods occur from May to June and overlap spawning (Scott & Crossman Citation1973; Smith et al. Citation2005), as in the Missouri River in 2011. As Centrarchids, white crappies typically reproduce in floodplain areas <1.5m deep with sand and clay substrates and aquatic macrophytes (Miranda et al. Citation2015). Vegetation promotes Centrarchid spawning by providing protection from wave turbulence (Pope & Willis Citation1997). In contrast to bluegill and black crappie, which became more abundant after the flood, white crappie recruitment is not driven by macrophyte availability, because soon after hatching in May (Sheik et al. Citation1998), fry leave spawning areas for deeper water (Nelson et al. Citation1967; Beam Citation1983), where they are more vulnerable to downstream displacement by flooding (Harvey Citation1987; Bishoff & Wolter Citation2001). Thus, although river–floodplain connectivity may benefit some species by increasing vegetation availability, floods can ultimately decrease juvenile white crappie abundance (Boxrucker et al. Citation2005), as in our study.
Morphology, introduction history, and recreational importance were also informative categories for deciphering the effects of the flood on different segments of the Delta fish community. The flood increased relative abundance of large-bodied species but not small-bodied fishes. Although large-bodied species occupy the Delta primarily during the spawning season, a universal increase in relative abundance suggests movement into the Delta, where floodplain inundation likely facilitated reproduction (Gerking Citation1950; Trepanier et al. Citation1996; Bice et al. Citation2014). Consistent relative abundance of small-bodied species is predictable because the Delta contains interspersed fluvial and slackwater habitats favorable for spawning, foraging, and refuge from predators (Kaemingk et al. Citation2007; Schreck Citation2010). In other floodplain rivers, floods may decrease small-bodied fish abundance by restructuring and reducing macrophyte cover (Bice et al. Citation2014). Although we did not specifically evaluate the effects of the 2011 flood on macrophytes, stable relative abundance suggests that the Delta contained suitable habitats for small-bodied fishes during and after the disturbance. We acknowledge that our sampling methodology permitted collection of only three small-bodied species in sufficient abundance for long-term assessment of population trends. However, effects of floods on these species have not been investigated in the Delta and thus represent novel information (Schreck Citation2010).
The flood increased relative abundance of both introduced and native fishes, supporting previous studies in floodplain rivers (Stoffels et al. Citation2014; Vilizzi et al. Citation2014). Common carp, the only introduced fish that was sufficiently abundant for population assessment in this study, can have profound negative effects on aquatic ecosystems by resuspending sediments and increasing turbidity (Kloskowski Citation2011). With high adaptability and prolific spawning capacity, common carp readily exploit floodplain habitats under diverse hydrological conditions (Koel & Sparks Citation2002; Penne & Pierce Citation2008; Sommer et al. Citation2014). It is pertinent for fisheries managers to design water management strategies that benefit native species in deltas without simultaneously promoting introduced fishes (Stoffels et al. Citation2014). Floodplain inundation alone may not be sufficient to limit introduced species (Sommer et al. Citation2014). However, naturalization of the flow regime with respect to the magnitude, frequency, duration, timing, and rate of change of water levels (Richter et al. Citation1996) may offer native species a competitive advantage for food and habitat resources (Koel & Sparks Citation2002). From a fisheries management perspective, it is important to manage reservoirs in ways that maintain or enhance sport fish production (Sammons et al. Citation2001). Relative abundance of adult sport fishes (bluegill, channel catfish, largemouth bass, sauger) and non-recreational species (freshwater drum, river carpsucker, shorthead redhorse) increased after the flood in the Delta as floodplain inundation reconnected habitats and augmented resource availability. However, juvenile sport fish CPUE did not increase for all species (e.g., white crappie). We recommend fisheries and water managers collaboratively manage reservoirs to promote sport fish production across their life history. Drivers of juvenile and adult abundance need to be identified, and a seasonally variable flow regime will likely need to be maintained to promote spawning, rearing, and foraging at appropriate times throughout the year.
The effects of the flood on aquatic habitats in the Delta provided insight for interpreting fish community trends. Main channels, side channels, and backwaters became narrower, smaller, and less numerous after the flood, whereas sandbar occurrence increased. Such reduction in habitat complexity was evidently not detrimental to the structurally resistant fish community. This supports the flood pulse concept (Junk et al. Citation1989) and a contemporaneous Missouri River study (Steffensen et al. Citation2014) that concluded that the flood was beneficial for the fish community. Compared to the reservoir portion of Lewis and Clark Lake, the Delta contained abundant interspersed fluvial and slackwater habitats that provided refuge from floodwaters (Schwartz & Herricks Citation2005; Koizumi et al. Citation2013). Thus, in addition to its importance as a spawning and rearing location for many Missouri River fishes under normal hydrological conditions (Kaemingk et al. Citation2007; Graeb et al. Citation2009), the Delta served an important ecological function during an historic flood. This study underscores the importance of maintaining habitat connectivity in deltas during and after floods to promote diverse fish communities. However, delta ecology is a nascent field. Further study is needed to determine how these ecosystems may contribute to river restoration throughout the world. In particular, long-term effects and ecological implications of floods warrant further research to inform management of water, sediments, and fisheries in deltas.
Acknowledgements
We thank L. Heironimus, C. Hayer, T. Rapp, and J. Mecham from South Dakota State University for field assistance. We thank B. Hanten, H. Meyer, K. Potter, G. Knecht, J. Sorensen, and R. Trible from the South Dakota Department of Game, Fish and Parks for technical advice and assistance.
Disclosure statement
No potential conflict of interest was reported by the authors.
Additional information
Funding
References
- Aadland LP. 1993. Stream habitat types: their fish assemblages and relationship to flow. North Am J Fish Manag. 13:790–806.
- Akankali JA, Jamabo NA. 2012. Effects of flooding and erosion on fisheries resources in Niger Delta, Nigeria. Eur J Sci Res. 90:14–25.
- Barko VA, Herzog DP, O'Connell MT. 2006. Response of fishes to floodplain connectivity during and following a 500-year flood event in the unimpounded upper Mississippi River. Wetlands. 26:244–257.
- Beam JH. 1983. The effect of annual water level management on population trends of white crappie in Elk City Reservoir, Kansas. North Am J Fish Manag. 3:34–40.
- Becker GC. 1983. Fishes of Wisconsin. Madison (WI): University of Wisconsin Press.
- Bednarski J, Miller SE, Scarnecchia DL. 2008. Larval fish catches in the lower Milk River, Montana in relation to timing and magnitude of spring discharge. River Res Appl. 24:844–851.
- Bice CM, Gehrig SL, Zampatti BP, Nicol JM, Wilson P, Leigh SL, Marsland K. 2014. Flow-induced alterations to fish assemblages, habitat and fish-habitat associations in a regulated lowland river. Hydrobiologia. 722:205–222.
- Bischoff A, Wolter C. 2001. The flood of the century on the River Oder: effects on the 0+ fish community and implications for floodplain restoration. Regul Rivers. 17:171–190.
- Boxrucker JC, Summers GL, Gilliland ER. 2005. Effects of the extent and duration of seasonal flood pool inundation on recruitment of threadfin shad, white crappies, and largemouth bass in Hugo Reservoir, Oklahoma. North Am J Fish Manag. 25:709–716.
- Clarke KR, Gorley RN. 2006. PRIMER v6: user manual/tutorial. Plymouth: PRIMER-E.
- Coker EH, Hotchkiss RH, Johnson DA. 2009. Conversion of a Missouri River dam and reservoir to a sustainable system: sediment management. J Am Water Resour Assoc. 45:815–827.
- Connell JH, Sousa WP. 1983. On the evidence needed to judge ecological stability or persistence. Am Nat. 121:789–824.
- Cowman T. 2012. Impacts of the 2011 flood on the Missouri River channel. Missouri River Institute, University of South Dakota. Available from: http://www.usd.edu/missouri-river-institute/upload/Impactsof2011Flood.pdf
- DeBoer JA, Ogren SA, Holtgren JM, Snyder EB. 2011. A 100-year flood in a low-gradient stream: response of the resident and non-resident fish assemblages. Am Midl Nat. 166:446–452.
- Elliott CM, Jacobson RB. 2006. Geomorphic classification and assessment of channel dynamics in the Missouri National Recreational River, South Dakota and Nebraska. Washington (DC): US Geological Survey. (Scientific Investigations Report 2006-5313).
- Erickson JW, Rath MD, Best D. 2008. Operation of the Missouri River reservoir system and its effects on fisheries management. In: Allen MS, Sammons S, Maceina MJ, editors. Balancing fisheries management and water uses for impounded river systems. Bethesda (MD): American Fisheries Society; p. 117–134.
- Franssen NR, Gido KB, Guy CS, Tripe JA, Shrank SJ, Strakosh TR, Bertrand KN, Franssen CM, Pitts KL, Paukert CP. 2006. Effects of floods on fish assemblages in an intermittent prairie stream. Freshw Biol. 51:2072–2086.
- Freitas CEC, Siqueira-Souza FK, Guimaraes AR, Santos FA, Santos ILA. 2010. Interconnectedness during high water maintains similarity in fish assemblages of island floodplain lakes in the Amazonian Basin. Zoologia. 27:931–938.
- Galat DL, Berry CR, Gardner WM, Hendrickson JC, Mestl GE, Power GJ, Stone C, Winston MR. 2005. Spatiotemporal patterns and changes in Missouri River fishes. In: Rinne JN, Hughes RM, Calamusso B, editors. Historical changes in large river fish assemblages of the Americas. Bethesda (MD): American Fisheries Society; p. 249–291.
- Gerking SD. 1950. Stability of a stream fish population. J Wildlife Manage. 14:193–202.
- Gido KB, Propst DL, Molles MC. 1997. Spatial and temporal variation of fish communities in secondary channels of the San Juan River, New Mexico and Utah. Environ Biol Fish. 49:417–434.
- Gomes LC, Bulla CK, Agostinho AA, Vasconcelos LP, Miranda LE. 2012. Fish assemblage dynamics in a Neotropical floodplain relative to aquatic macrophytes and the homogenizing effect of a flood pulse. Hydrobiologia. 685:97–107.
- Graeb BDS, Willis DW, Spindler BD. 2009. Shifts in sauger spawning locations after 40 years of reservoir ageing: influence of a novel delta ecosystem in the Missouri River, USA. River Res Appl. 25:153–159.
- Graf WL, Wohl E, Sinha T, Sabo JL. 2010. Sedimentation and sustainability of western American reservoirs. Water Resour Res. 46:1–13.
- Harvey BC. 1987. Susceptibility of young-of-the-year fishes to downstream displacement by flooding. Trans Am Fish Soc. 116:851–855.
- Junk WJ, Bayley PB, Sparks RE. 1989. The flood pulse concept in river-floodplain systems. In: Dodge DP, editor. Proceedings of the international large river symposium. Ottawa: Canadian Special Publication of Fisheries and Aquatic Sciences; p. 110–127.
- Jurajda P, Reichard M, Smith C. 2006. Immediate impact of an extensive summer flood on the adult fish assemblage of a channelized lowland river. J Freshwater Ecol. 21:493–501.
- Kaemingk MA, Graeb BDS, Hoagstrom CW, Willis DW. 2007. Patterns of fish diversity in a mainstem Missouri River reservoir and associated delta in South Dakota and Nebraska, USA. River Res Appl. 23:786–791.
- Kano Y, Ohnishi K, Tomida Y, Ikeda N, Iwawaki N, Miyagawa M, Harada Y, Ichiyanagi H, Watanabe K. 2011. Fluctuation and variation in stream-fish assemblages after a catastrophic flood in the Miyagawa River, Japan. Environ Biol Fish. 92:447–460.
- Kempton RA, Taylor LR. 1974. Log-series and log-normal parameters as diversity discriminants for the Lepidoptera. J Anim Ecol. 43:381–399.
- Kloskowski J. 2011. Impact of common carp Cyprinus carpio on aquatic communities: direct trophic effects versus habitat deterioration. Fund Appl Limnol. 178:245–255.
- Koel TM, Sparks RE. 2002. Historical patterns of river stage and fish communities as criteria for operations of dams on the Illinois River. River Res Appl. 18:3–19.
- Koizumi I, Kanazawa Y, Tanaka Y. 2013. The fishermen were right: experimental evidence for tributary refuge hypothesis during floods. Zool Sci. 30:375–379.
- Kwak TJ, Peterson JT. 2007. Community indices, parameters, and comparisons. In: Guy CS, Brown ML, editors. Analysis and interpretation of freshwater fisheries data. Bethesda (MD): American Fisheries Society; p. 677–763.
- Lobon-Cerviá J. 1996. Response of a stream fish assemblage to a severe spate in northern Spain. Trans Am Fish Soc. 125:913–919.
- Lojkasek B, Lusk S, Halacka K, Luskova W, Drozd P. 2005. The impact of the extreme floods in July 1997 on the ichthyocenosis of the Oder Catchment area (Czech Republic). Hydrobiologia. 548:11–22.
- Long CM, Pavelsky TM. 2013. Remote sensing of suspended sediment concentration and hydrologic connectivity in a complex wetland environment. Remote Sens Environ. 129:197–209.
- Lukas JA, Orth DJ. 1995. Factors affecting nesting success of smallmouth bass in a regulated Virginia stream. Trans Am Fish Soc. 124:726–735.
- Matthews WJ. 1986. Fish faunal structure in an Ozark stream: stability, persistence and a catastrophic flood. Copeia. 1986:388–397.
- Minckley WL, Meffe GK. 1987. Differential selection by flooding in stream fish communities of the arid American southwest. In: Matthews WJ, Heins DC, editors. Community and evolutionary ecology of North American stream fishes. Norman: University of Oklahoma Press; p. 93–104.
- Miranda LE, Dagel JD, Kaczka LJ, Mower EB, Wigen SL. 2015. Floodplains within reservoirs promote earlier spawning of white crappies Pomoxis annularis. Environ Biol Fish. 98:469–476.
- Montgomery JC, Fickeisen DH, Becker CD. 1980. Factors influencing smallmouth bass production in the Hanford Area, Columbia River. Northwest Sci. 54:296–305.
- National Research Council. 2011. The Missouri River ecosystem: exploring the prospects for recovery. National Academies Press. Available from: http://www.nwd-mr.usace.army.mil/mmanual/MRMM_NAS_Study_EXSUMSections1%262.pdf
- Nelson WR, Siefert RE, Swedberg DV. 1967. Studies of the early life history of reservoir fishes. In: Reservoir Committee of the Southern Division, American Fisheries Society, editor. Reservoir fishery resources symposium. Athens: University of Georgia Press; p. 374–385.
- Penne CR, Pierce CL. 2008. Seasonal distribution, aggregation, anal habitat selection of common carp in Clear Lake, Iowa. Trans Am Fish Soc. 137:1050–1062.
- Perry RW, Brandes PL, Burau JR, Sandstrom PT, Skalski JR. 2015. Effect of tides, river flow, and gate operations on entrainment of juvenile salmon into the interior Sacramento-San Joaquin River delta. Trans Am Fish Soc. 144:445–455.
- Pflieger WF. 1971. A distributional study of Missouri fishes. Museum of natural history. Vol. 20. Lawrence: University of Kansas Publication; p. 225–570.
- Pires AM, Magalhaes MF, Da Costa LM, Alves MJ, Coelho MM. 2008. Effects of an extreme flash flood on the native fish assemblages across a Mediterranean catchment. Fish Manag Ecol. 15:49–58.
- Poff NL, Allan JD, Bain MB, Karr JR, Prestegaard KL, Richter BD, Sparks RE, Stromberg JC. 1997. The natural flow regime. Bioscience. 47:769–784.
- Pope KL, Willis DW. 1997. Environmental characteristics of black crappie (Pomoxis nigromaculatus) nesting sites in two South Dakota waters. Ecol Freshw Fish. 6:183–189.
- Preston FW. 1962. The canonical distribution of commonness and rarity: part I. Ecology. 43:85–215.
- Richter BD, Baumgartner JV, Powell J, Braun DP. 1996. A method for assessing hydrologic alteration within ecosystems. Conserv Biol. 10:1163–1174.
- Ross ST, Baker JA. 1983. The response of fishes to periodic spring floods in a southeastern stream. Am Midl Nat. 109:1–14.
- Sabo MJ, Orth DJ. 1994. Temporal variation in microhabitat use by age-0 smallmouth bass in the North Anna River, Virginia. Trans Am Fish Soc. 123:733–746.
- Sammons SM, Bettoli PW, Greear VA. 2001. Early life history characteristics of age-0 white crappies in response to hydrology and zooplankton densities in Normandy Reservoir, Tennessee. Trans Am Fish Soc. 130:442–449.
- Scheerer PD. 2002. Implications of floodplain isolation and connectivity on the conservation of an endangered minnow, Oregon chub, in the Willamette River, Oregon. Trans Am Fish Soc. 131:1070–1080.
- Schreck WJ. 2010. Seasonal use of Missouri River reservoir deltas by fishes [M.S. thesis]. Brookings (SD): South Dakota State University.
- Schwartz JS, Herricks EE. 2005. Fish use of stage-specific fluvial habitats as refuge patches during a flood in a low-gradient Illinois stream. Can J Fish Aquat Sci. 62:1540–1552.
- Scott WB, Crossman EJ. 1973. Freshwater fishes of Canada. Fisheries Research Board of Canada Bulletin, 184.
- Sheik RA, Fisher SJ, Willis DW. 1998. White crappie biology in an upper Missouri River backwater. Proc SD Acad Sci. 77:151–161.
- Simonson TD, Swensen WA. 1990. Critical stream velocities for young-of-year smallmouth bass in relation to habitat use. Trans Am Fish Soc. 119:902–909.
- Siziba N, Chimbari MJ, Mosepele K, Masundire H. 2011. Spatial and temporal variations in densities of small fishes across different temporary floodplain types of the lower Okavango Delta, Botswana. Afr J Aquat Sci. 36:309–320.
- Smith SM, Odenkirk JS, Reeser SJ. 2005. Smallmouth bass recruitment variability and its relation to stream discharge in three Virginia rivers. North Am J Fish Manag. 25:1112–1121.
- Sommer TR, Harrell WC, Feyrer F. 2014. Large-bodied fish migration and residency in a flood basin of the Sacramento River, California, USA. Ecol Freshwater Fish. 23:414–423.
- Spindler BD, Chipps SR, Klumb RA, Graeb BDS, Wimberly MC. 2012. Habitat and prey availability attributes associated with juvenile and early adult pallid sturgeon occurrence in the Missouri River, USA. Endanger Species Res. 16:225–234.
- Spindler BD, Chipps SR, Klumb RA, Wimberly MC. 2009. Spatial analysis of pallid sturgeon Scaphirhynchus albus distribution in the Missouri River, South Dakota. J Appl Ichthyol. 25:8–13.
- Steffensen KD, Eder BL, Pegg MA. 2014. Fish community response to floodplain inundation in a regulated river. J Freshw Ecol. 29:413–427.
- Stoffels RJ, Clarke KR, Rehwinkel RA, McCarthy BJ. 2014. Response of a floodplain fish community to river-floodplain connectivity: natural versus managed reconnection. Can J Fish Aquat Sci. 71:236–245.
- Swedberg DV, Walburg CH. 1970. Spawning and early life history of the freshwater drum in Lewis and Clark Lake, Missouri River. Trans Am Fish Soc. 99:560–570.
- Tockner K, Malard F, Ward JV. 2000. An extension of the flood pulse concept. Hydrol Process. 14:2861–2883.
- Trepanier S, Rodriguez MA, Magnan P. 1996. Spawning migrations in landlocked Atlantic salmon: time series modelling of river discharge and water temperature effects. J Fish Biol. 48:925–936.
- US Army Corps of Engineers. 2001. Niobrara and Missouri rivers, South Dakota and Nebraska: sediment strategies. Omaha (NE): US Army Corps of Engineers.
- US Army Corps of Engineers. 2006. Missouri River mainstem reservoir system master water control manual. Omaha (NE): US Army Corps of Engineers, Northwestern Division – Missouri River Basin.
- US Army Corps of Engineers. 2011. Lewis and Clark Lake sediment management study: modeling summary. Omaha (NE): US Army Corps of Engineers.
- US Army Corps of Engineers. 2012. Post 2011 flood event analysis of Missouri River mainstem flood control storage. Omaha (NE): US Army Corps of Engineers.
- US Army Corps of Engineers. 2013. Lewis and Clark Lake Sediment Management Study (LCLSMS). Part III: HEC-RAS sediment modeling from Gavins Point Dam to Sioux City, IA. Omaha (NE): US Army Corps of Engineers.
- Vilizzi L, Thwaites LA, Smith BB. 2014. Exploitation by common carp (Cyprinus carpio) of a floodplain wetland of the lower River Murray under drought and flooding conditions. Trans R Soc S Aust. 138:113–123.
- Volke MA, Scott ML, Johnson WC, Dixon MD. 2015. The ecological significance of emerging deltas in regulated rivers. Bioscience. 65:598–611.
- Ward JV, Stanford JA. 1995. Ecological connectivity in alluvial river ecosystems and its disruption by flow regulation. Regul Rivers. 11:105–119.
- Ward JV, Tockner K, Schiemer F. 1999. Biodiversity of floodplain river ecosystems: ecotones and connectivity. Regul Rivers. 15:125–139.
- Wickstrom G. 2004. Annual fish population surveys of Lewis and Clark Lake. Pierre: South Dakota Department of Game, Fish and Parks. (Fisheries Division Report 05-15).
- Zampatti B, Leigh S. 2013. Effects of flooding on recruitment and abundance of golden perch (Macquaria ambigua ambigua) in the lower River Murray. Ecol Manag Rest. 14:135–143.