ABSTRACT
As temperatures rise and precipitation patterns change, approaches are needed for early detection of the effects on ecosystems. Macroinvertebrate data collected from 81 sites with multiple habitat kick sampling were compiled for this study. We used a binary logistic regression model to predict the probability of local extinction or range expansion of aquatic macroinvertebrates with changes in temperature and precipitation in watersheds of Castilla-La Mancha, Spain. Aquatic insect families from the following orders were found to be sensitive to an increase in average spring temperatures: Ephemeroptera, Plecoptera, Megaloptera, Trichoptera, Coleoptera and Diptera. Taxa in Ephemeroptera, Trichoptera, Hemiptera and Coleoptera showed negative relationships with minimum (winter) temperature, maximum spring temperature and/or precipitation. One mayfly, Caenis, exhibited a positive relationship with minimum temperature. These results indicate that these aquatic communities are expected to change significantly in the short-term, with the potential to alter stream ecosystem functioning. Mediterranean ecosystems are considered biodiversity hotspots, and biotic communities in freshwater habitats in these areas may be particularly vulnerable to projected increases in temperature and decreases in precipitation. The ability of taxa to withstand these changes will depend on resistance, resilience and dispersal capabilities of the aquatic macroinvertebrates as well as available habitat.
Introduction
Mediterranean region streams have unique community structures adapted to natural disturbance, especially in relation to the markedly seasonal precipitation and periodic drought typical of this climate type, as well as a long history of anthropogenic disturbance (Gasith and Resh Citation1999; Bolle Citation2012; Filipe et al. Citation2012; IPCC Citation2013; Bonada and Resh Citation2013). Regional projections of climate patterns in the next decades include rising temperatures, less frequent and more severe precipitation events, more severe droughts and lower annual precipitation (Arnell Citation1999a, Citation1999b; Arnell Citation2004; Milly et al. Citation2005; IPCC Citation2013). These changes are expected to affect the quality and quantity of aquatic habitat through top-down and bottom-up mechanisms, altering ecosystem structure and function. Changes in these communities would be expected to have a large effect on the entire aquatic ecosystem, in part due to the important role aquatic macroinvertebrates play in aquatic and terrestrial food webs (Wallace and Webster Citation1996; Nakano et al. Citation1999; Nakano and Murakami Citation2001).
For the period 1970–1997 in the Segura Watershed, Spain, spring temperatures increased more than in any other season, annual maximum temperature increased by 0.125 °C year−1 (Horcas et al. Citation2001), and short-term projections indicate an increase by 0.3–0.7 °C for the period 2016–2035 relative to 1986–2005 (IPCC Citation2013). Regional climate models for Castilla-La Mancha, Spain, predict increases in temperatures in summer (5–7 °C) and winter (3–4 °C) by the year 2070 for the A2 scenario (high population growth, locally focused economic solutions), with approximately 1 °C lower for B2 scenario predictions (medium population growth with a focus on local solutions to environmental issues; PROMES, Gallardo et al. Citation2001, and PRUDENCE, De Castro et al. Citation2004; Christensen and Christensen Citation2007). By 2100, PRUDENCE results for the A2 scenario predict greater variability in temporal patterns and reductions in winter precipitation of 0–0.25 mm day−1 and summer precipitation of up to 0.5 mm day−1 in south-eastern Spain, which translates to 90–180 mm year−1 for some areas that receive only 400 mm year−1 on average (De Castro et al. Citation2004). This would shift Mediterranean climate regions to arid climates (Arnell Citation1999b). Threats to biodiversity as well as freshwater resources are predicted for all Mediterranean watersheds with these changes in precipitation and temperature (Vörösmarty et al. Citation2010).
Temperature is the main influencing factor on life cycles and metabolism of insects and non-insect macroinvertebrates (Howe Citation1967; Vannote et al. Citation1980; Brittain Citation1982; Merritt et al. Citation1982; Sweeney et al. Citation1992, Citation1995). Observational studies on the effects of rising temperatures on aquatic insect communities have documented northward range shifts in the United Kingdom (Hickling et al. Citation2005), increases or decreases in abundance of some taxa in Northern Europe (Burgmer et al. Citation2007), earlier emergence (Hogg and Williams Citation1996; Harper and Peckarsky Citation2006; Cid et al. Citation2008), declines in fitness (Peckarsky et al. Citation2001) related to metabolic costs and reduced taxa richness (Arai et al. Citation2015). Predictive models based on historical data on aquatic insect communities show potential for further changes, including decreases in abundance and local extinction (Bonada et al. Citation2007; Durance and Ormerod Citation2007) due to reduced availability of suitable habitat (Domisch et al. Citation2013). However, other studies show little to no predicted change in composition (Domisch et al. Citation2011). Therefore, analyses are needed for specific regions to detect threats and patterns.
The interaction between low precipitation and warm annual temperatures defines the Mediterranean region as water-stressed (UNCED Citation1993; Bates et al. Citation2008), and life histories of aquatic taxa are adapted to periods of drought with timing of less vulnerable life stages or use of refugia (Gasith and Resh Citation1999). Upland streams rely heavily on baseflow from groundwater, but they will be affected by decreased availability of groundwater and surface water through changes in precipitation patterns (Durance and Ormerod Citation2007; Xu et al. Citation2009; Kingston and Taylor Citation2010). Changes in precipitation will affect the quality and quantity of available habitat via changes in surface and groundwater quality and quantity, water temperature, wetted area, velocity regimes and seasonal discharge patterns, including more extreme floods and droughts (Swift et al. Citation1988; Boix-Fayos et al. Citation1998; Arnell Citation1999a, Citation1999b; Bonada et al. Citation2004; Filipe et al. Citation2012; Arai et al. Citation2015; Tang et al. Citation2015). Streamflow will be impacted greatly by increased temperatures as well due to relatively high evapotranspiration rates (Vicuña et al. Citation2011). Water quantity is an important aspect of available habitat given hydraulic preferences of taxa, seasonal changes in flow, temperature and water quality as well as the interconnectedness of these habitat characteristics (Poff et al. Citation1997; Chadwick et al. Citation2006).
Temperature tolerance data are available in some areas of the world for some aquatic insect taxa (Merritt et al. Citation2008; Tachet et al. Citation2010; Dallas and Rivers-Moore Citation2012; Chown et al. Citation2015), but this information is imprecise and/or scarce for Iberian taxa. Flow preferences have also been documented for types of waterways and flow categories (i.e. temporary or perennial streams, slow or fast-flow) (Extence et al. Citation1999; Tachet et al. Citation2010) or for setting ecological flows (Bovee Citation1982). Temperature and flow are major drivers of the distribution of an organism (Southwood Citation1977), and can be directly related to habitat and distribution with a statistical species distribution model (Durance and Ormerod Citation2007; Kearney and Porter Citation2009). Although the species distribution model approach has its criticisms (Araújo and Peterson Citation2012; Railsback Citation2016), it can be useful for a first estimate of the macroinvertebrates that are most sensitive to rising temperatures and decreasing precipitation, and it can be applied when the main assumptions are low influence of biotic interactions and dispersal limitations (Hampe Citation2004).
In this study, we used observed distribution patterns of aquatic macroinvertebrate taxa to predict the possible effects of increased temperatures and decreased precipitation in three watersheds in Castilla-La Mancha, Spain. To examine changes in temperature and precipitation in a few meaningful metrics, we used a one-degree increase in three temperature metrics (average spring temperature, minimum winter temperature and maximum spring temperature) and two different measures of precipitation (100 mm decrease in annual precipitation and an increase by 0.1 (unitless) in the winter North Atlantic Oscillation (NAO) Index values).
Hypotheses on aquatic macroinvertebrate sensitivity to changes in temperature and precipitation were based on functional traits (Extence et al. Citation1999; Doledec et al. Citation2007; Tachet et al. Citation2010) and our experience on the distributions of taxa, specifically those known to be present in small, cool streams (Mellado Citation2005; Navarro-Llácer Citation2006). The aquatic macroinvertebrates we expected to be relatively sensitive to a one-degree increase in temperature include all Plecoptera; Trichoptera: all genera within Limnephilidae, Sericostoma spp. (Sericostomatidae), Agapetus spp. (Glossosomatidae); Hemiptera: Aphelocheirus sp. (Aphelocheiridae), Ephemeroptera: Paraleptophlebia spp. (Leptophlebiidae); Diptera: Atrichops and Atherix spp. (Athericidae) and Sialis spp. (Sialidae) based on our work. Reduced precipitation is expected to negatively affect taxa that require fast-flowing waters: all Plecoptera; Ephemeroptera: Ecdyonurus, Epeorus, Heptagenia, Rithrogena spp. (Heptageniidae), Paraleptophlebia spp. (Paraleptophlebiidae); Trichoptera: Hydropsyche spp., Cheumatopsyche spp. (Hydropsychidae); Coleoptera: Dryops spp. (Dryopidae). We expected these taxa to respond to the predictors because they are likely to be the first affected by changes in temperature and precipitation. Macroinvertebrates not expected to show responses with temperature or precipitation include taxa that are ubiquitously distributed, disturbance tolerant or show wide variability in genus level traits: all other Diptera, Coleoptera and Hemiptera included, Baetidae, Caenis spp. (Caenidae); Potamanthus spp. (Potamanthidae); Hydroptila spp. (Hydroptilidae); Chimarra spp. (Philopotamidae); Setodes, Athripsodes spp. (Glossosomatidae), Lype, Metalype, Tinodes, Psychomyia spp. (Psychomyiidae), Gammaridae and Dugesiidae. The present study is a first step toward understanding short-term changes in freshwater biota with an increase in temperature and a decrease in precipitation in a Mediterranean climate, an important approach for detecting early signs of these changes (Filipe et al. Citation2012).
Methods
Biomonitoring data
The study area includes three watersheds in Castilla-La Mancha, Spain: the Tajo, Júcar and Segura Watersheds (). Annual precipitation in the headwaters and mid-reaches of all three watersheds studied ranges from 400 to 800 mm, with the highest amounts in the Júcar and Tajo Watersheds and lower values in the Segura Watershed. Average annual air temperature is between 12 and 15 °C for the entire study area for the period 1971–2000 (Moreno et al. Citation2005).
Figure 1. Map of study area: the Tajo, Júcar and Segura headwaters contained in Castilla-La Mancha Autonomous Community. Weather stations are marked with triangles, sample sites are marked with circles.
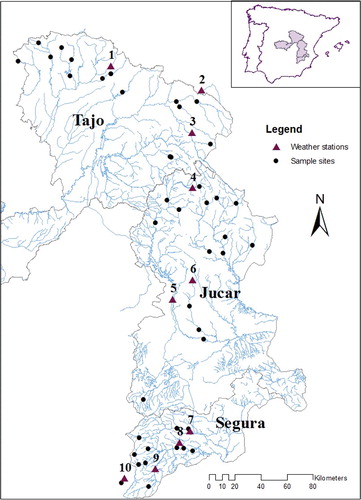
Macroinvertebrate data collected from 2001–2012 for regional stream biomonitoring were downloaded from the Regional Center for Water Studies database (CREA, University of Castilla-La Mancha, Albacete). Samples were collected with 250 μm mesh kick-nets and a multiple habitat approach through the use of two different methods: qualitative sampling following the GUADALMED/PREECE protocol (Jáimez-Cuéllar et al. Citation2002) and semi-quantitative sampling using the 20-kick multihabitat method (Barbour et al. Citation1999; Hering et al. Citation2004). A subset of 250 individuals was subsampled and identified to family or genus for both sampling methods. We combined data from the two sampling methods by converting abundance to presence–absence. One study comparing qualitative and semi-quantitative sampling methods failed to detect significant differences in biotic indices due to sampling protocol (Bonada et al. Citation2002).
Macroinvertebrate family data from 81 samples taken in spring, summer or fall from 39 sites in headwater streams were compiled from different years between 2001 and 2009 (). Some sites were sampled only once, others were sampled during different years (maximum 4). Although some seasonal variation has been observed, sampling any season except winter yields relatively similar communities in this region (Hering et al. Citation2004; Navarro-Llácer Citation2006; Vannucchi et al. Citation2017). Multiple samples at a site during different years are treated as independent because they are being related to annual weather metrics. These samples were identified to family, but trait information is available at the genus level. We researched the genera present within each family (Bonada et al. Citation2004; Mellado Citation2005; Navarro-Llácer Citation2006). To verify these genera, we identified subset of 13/81 of the samples to genus as well as 57 independent samples not included in this study, and these genera were used in the model, with probability of presence–absence as the response variables, and temperature and precipitation metrics as predictors. Macroinvertebrate families were chosen for the model if they were not present in all locations sampled because the statistical analyses require absence in some areas to derive regression relationships. We chose taxa for the analysis based on traits related to temperature and flow. These traits included respiration through tegument or gills, cold water or possibly eurythermic taxa, oligo- or mesotrophic, distribution in the crenon or rhithron, use of coarse substrate and lotic waters (Traits 10,11,13,18, 20, 22 in Tachet et al. Citation2010).
Researchers debate the best methods for application of species distribution models regarding presence–absence vs. abundance data (Howard et al. Citation2014), scale, and their accuracy and precision (Pearson and Dawson Citation2003), among other considerations. For aquatic macroinvertebrates, this approach can be confounded by local habitat effects. In this study, we chose sites from upper reaches where there is no apparent influence of flow regulation by dams and little developed land (), and therefore low direct influence of human activities on stream ecosystems. All sites are considered reference conditions (Navarro-Llácer Citation2006). Therefore, our assumptions are (1) that presence of a taxon is a relevant indicator of habitat suitability, (2) that there will be source populations nearby for taxa able to expand in range and (3) that taxa distributions can be directly related to climate metrics. Although using regression has its criticisms (García-Valdés et al. Citation2013), it has been applied in different areas of ecological research (Shaunbhogue and Gore Citation1987; Trexler and Travis Citation1993; Manel et al. Citation1999; Keating and Cherry Citation2004; Wilson Citation2009). Although logistic regression has not been used extensively for freshwater macroinvertebrate response to climate change, it is appropriate to explore which taxa might be the first affected by increasing temperature and decreasing precipitation, and can be used to suggest locations and taxa for more in-depth study.
Table 1. Summary of taxa included in study and number of samples present in each watershed.
Table 2. Number of samples, mean and standard deviation of climate variables and land use/land cover (Corine Citation2000). Abbreviations are: MinT: minimum winter temperature, Appt: annual precipitation, NAO winter: average NAO index value for December–February, Asprt: average spring temperature, Msprt: maximum spring temperature.
Meteorological data
Meteorological data for our temperature and precipitation metrics were obtained from the nearest weather station for all points from the National Meteorological Agency (AEMET Citation2012). Average and maximum spring temperatures were used because of their relationships with emergence cues and developmental rates, especially in reference to earlier emergence (Sweeney et al. Citation1995; Hogg and Williams Citation1996); small increases in temperatures can also affect egg hatching, growth rate, grouping or mating (Wright et al. Citation1982; Sweeney Citation1993; Romey and Rossman Citation1995; Harper and Peckarsky Citation2006; Haidekker and Hering Citation2008; Arribas et al. Citation2012) ().
Water temperature metrics
Water temperature tends to fluctuate with the same pattern as air temperature, but with smaller amplitude (Sweeney Citation1993; Mohseni and Stefan Citation1999). Continuous temperature data were collected from the Lietor sample site in the Segura Watershed for the period April 2009–September 2009 with HOBO® temperature data loggers. The Lietor site logger was close to weather station 7, so these data were used to derive air–water temperature relationships. We calculated the metrics average spring temperature (AsprT), maximum spring temperature (MsprT) and minimum temperature (MinT) of water from air temperatures (AirT) with three separate equations; linear and polynomial lines were tested because extreme values have been found to show better fit with a polynomial relationship, while average values tend to fit a linear relationship (see Mohseni and Stefan Citation1999). The air–water temperature relationship fit a linear equation for average (AsprT = 0.53 × AirT + 4.67, R2 = 0.92) and maximum (MsprT = 1.85 × AirT − 1.85, R2 = 0.79) spring temperatures. A polynomial equation related minimum water and air temperatures: MinT = 0.009 × AirT2 + 0.82 × AirT + 0.584, R2 = 0.91.
Statistical analyses
We examined the relationships between water chemistry parameters (dissolved oxygen, pH, specific conductance, total nitrogen, total phosphorus, sulfate and major ions) and macroinvertebrate presence/absence for each taxon with a Generalized Linear Model: the only significant relationship was between Sericostoma spp. and specific conductance (results not shown). A Kruskal–Wallis, non-parametric analysis was performed with Mann–Whitney pairwise comparisons to detect differences in the presence/absence macroinvertebrate data among watersheds. We found no significant relationships with the taxa of interest. Thus, our assumptions for subsequent analyses were that water chemistry and watershed were not distinct factors.
Average spring temperature (AsprT), maximum spring temperature (MSprT), minimum winter temperature (MinT), NAO winter index value (NAOI) and annual precipitation (Appt) were used in binary logistic regression models for each taxon selected. Binary logistic regression uses a logistic distribution to predict the probability of change in the response variable (values 0 or 1), with a one-unit increase in a continuous predictor variable (Rawlings et al. Citation1998; Peng et al. Citation2002). A one-degree increase in air temperature can be expected to occur between 20 and 50 years from now (De Castro et al. Citation2004; IPCC Citation2013). Precipitation was divided by −100 so the model would predict change with a 100 mm decrease in precipitation. NAO values oscillate between 2 and −2, so a one-unit increase is not very likely in the near future. NAO index values were divided by 10 so the regression would examine the changes in taxa with a 0.1 unit increase in NAO (small increase in temperature and decrease in precipitation; Hurrell Citation1995). A forward conditional stepwise selection of predictor variables was applied so that the model contained only significant predictor variables (p < 0.05). Unless specified above, all analyses were performed with SPSS software 17.0 (Somers, NY). The output of the regression models was used to determine how changes in temperature and precipitation relate to the probability of presence of the taxa studied.
Results
The binary logistic regression models with climate variables as predictors were significant for predicting the probability of the presence or absence of 15 families (28 potential genera; and ). The other 18 families (21 potential genera) had non-significant binary logistic regression models. The output of logistic regression is the probability that the dependent variable (each taxon) will change from 0 to 1 or 1 to 0 with a one-unit increase in the predictor variable or variables. The constant e raised to the power of the model coefficient (eB) describes the probability of change. When eB > 1, the odds ratio (OR) = eB, and the taxon is likely to appear/be present (change from 0 to 1) with a one-unit increase in the predictor variable at the probability indicated by eB. For example, with eB of 1.11, Caenis is 1.11 times likely to be present with a one-unit increase in minimum winter temperature, or 111% likely to be present (change from belonging to group 0 to 1). When 0 < eB < 1, OR = 1/ eB, as in the case of Gyrinidae (eB = 0.72), the probability of taxon loss (change from 1 to 0) with one-unit change in the predictor variable, average spring temperature, calculated as the inverse of eB (1/0.721), is 1.389, or 139% likely to be absent ().
Table 3. Full binary logistic regression model statistics for variables with significant relationships. H-L stands for Hosmer–Lemeshow. All other abbreviations are as in 2.
Table 4. Binary logistic regression coefficients for taxa (response) and climate variables (predictor) of significant regression models. When eB only is reported, it represents the odds ratios. A separate OR column (odds ratio) is reported for eB values between 0 and 1. In these cases, OR = 1/eB. All other abbreviations are as in 2.
The taxa predicted by the binary logistic models as more likely to be absent with a one-degree increase in average spring temperature (AsprT): Nemouridae, Perlidae, Gyrinidae, Athericidae, Glossosomatidae, Limnephilidae, Sericostomatidae and Sialidae (). Caenis are likely to be found in more sites with a 1°C increase in MinT, while Aphelocheirus are likely to be absent from some sites with a 1°C increase in MsprT. The results for changes in taxa predicted by more than one independent climate variable appear to be contradicting at first glance but indicate more narrow temperature ranges: the model for Hydraenidae shows absence 210% likely due to a 1 °C increase in AsprT and presence 169% likely with a 1 °C increase in MsprT (). When this variable was analyzed for lower and upper values separately, with the median value (10.7 °C) as a threshold, the model was only significant when AsprT was greater than the median. Therefore, these patterns are predicted in areas with average temperatures higher than 10.7 °C. Similar explanations apply to the two-predictor-variable responses of Ephemerellidae, Philopotamidae and Psychomyiidae. Ephemerellidae and Philopotamidae are likely to be present with a 1 oC increase in MinT and likely to be absent for 1 oC higher AsprT. Psychomyiidae are predicted to be present with a 1 °C increase in MinT and likely to be absent with an increase in MsprT. No significant patterns were observed for any taxa with annual precipitation as the predictor variable, but Dryops responded to a change in winter NAO index.
Overall, no short-term change is expected in the taxonomic composition of the macroinvertebrate community for eight sites, located in the Segura (6) and Júcar (2) watersheds. Across all three watersheds, 23 samples from 15 sites may experience changes in 1–4 taxa, 54 samples from 26 sites contain 6–9 taxa that may be affected and 10 samples from 6 sites may experience a change in 10–13 of the taxa studied () under these conditions. Different responses were observed at the same sites from different years, shown by concentric circles in .
Discussion
For the aquatic macroinvertebrates studied, average spring temperature appears to be the variable most closely related to patterns of presence and absence. Cool-water adapted taxa with significant models in the Segura, Júcar and Tajo Watersheds include all Plecoptera, Hydraenidae, Athericidae, Limnephilidae and Sialidae. Extirpation of temperature-sensitive taxa may occur if habitat is not available in cooler areas upstream or in nearby headwater streams (Poff et al. Citation2002; Hering et al. Citation2009; De Figueroa et al. Citation2010). Similar patterns have been detected in Sweden for some of the same families and genera (Burgmer et al. Citation2007). To give one example of site-specific effects, at the Bornova River site in the Tajo Watershed, changes are expected for 13 taxa. While Caenis and Chimarra are expected to increase their range, 11 families may become absent from the Bornova River with increases in temperature (Ephemerellidae, Nemouridae, Perlidae, Dryopidae, Aphelocheiridae, Gyrinidae, Hydraenidae, Athericidae, Glossosomatidae, Limnephilidae and Sialidae). At this same site, 12 other families did not show significant patterns with climate metrics (Leptophlebiidae, Aeshnidae, Gomphidae, Leuctridae, Gerridae, Tabanidae, Tipulidae, Hydropsychidae, Hydroptilidae, Polycentropodidae, Rhyacophilidae and Dugesiidae).
Taxa tolerant to higher temperatures and changes in habitat will be able to increase in abundance and/or broaden their range (Burgmer et al. Citation2007), including invasive taxa (Rahel and Olden Citation2008), and particularly if other taxa occupying a similar niche in their communities or insect and fish predators become locally extinct. Range expansion of Caenis is expected to occur with a 1 °C increase in temperature in watersheds of Castilla-La Mancha. The possibility of range expansion to warmer reaches downstream will also rely on habitat availability; in many of the downstream reaches habitat quality is altered by dams, urban and agricultural inputs, altered flow regimes and degraded river morphology resulting from reservoir management (Vidal-Abarca Citation1990; Vidal-Abarca et al. Citation1992; Kroll et al. Citation2009; Navarro-Llácer et al. Citation2010; Kroll et al. Citation2013). Aside from warming temperatures, altered habitat downstream may also favor Caenis and other habitat generalists because they are well adapted to slow flows and fine substrate.
Taxa with poor dispersal capabilities or without access to suitable habitat nearby are expected to become locally extinct. The hemipteran Aphelocheirus aestivalus has no functional wings, and relies on drift for dispersal. Considering the already limited distribution of A. aestivalus in south-eastern Spain (10 sites), warmer waters are expected to be detrimental to populations. The capability for aerial dispersal may allow certain insects to disperse to new reaches of headwater streams in the same watershed or move to a nearby watershed. The headwaters of the Júcar and Tajo Watersheds are relatively close, as are the Segura and Guadiana Watershed headwaters (1.5–5 km apart in some areas). Nearly all taxa found to have significant relationships with temperature in this study have been documented to successfully disperse in the aquatic and aerial life stages (Tachet et al. Citation2010). Research within this region has shown increased dispersal in certain taxa of Coleoptera and Hemiptera with an increase in water temperature (Velasco and Millan Citation1998). Dispersal by flight, rather than drift, will become more important to range expansions or shifts under climate change scenarios in watersheds draining towards the poles and/or with anthropogenic stressors in downstream areas. If populations of macroinvertebrates can locate suitable habitat within the distance they can fly, range shifts are possible (Sweeney et al. Citation1995).
The present study is a first step toward understanding how the freshwater biota are expected to change with a one-degree increase in temperature in streams of south-eastern Spain. This study gives an understanding of short-term changes, as increases of up to 6 °C are projected by the end of this century (De Castro et al. Citation2004). This region has been widely cited as particularly susceptible to increases in temperature and decreases in precipitation over this century (Underwood et al. Citation2009; Filipe et al. Citation2012). Our method did not result in many relationships between taxa and precipitation. Therefore, it is likely that a more in-depth study would be needed regarding groundwater inputs, flow, available habitat, drought, flooding and precipitation. Our results could benefit from considering interactions with micro-habitat or meso-habitat not captured in this study, including aspects of water chemistry. Different predicted changes in taxa depending on the year of sampling is a finding that reinforces the need for multiple sampling events and considering annual variation in community analysis.
Macroinvertebrates present an important source of energy in freshwater ecosystems; changes in the community as a result of climate change would affect physiological processes such as metabolism and may induce top-down effects by local extinction of predators and bottom-up effects from altered nutrient inputs. This method can be expanded to include other organisms (fish, algae, macrophytes and other invertebrates) within these ecosystems for a more complete view of the expected changes in the structure and function of freshwater ecosystems as a result of increasing temperatures. The approach applied here offers insight on changes expected in the macroinvertebrate community due to increases in temperature using a statistical model. This method can be used to determine potential for the most sensitive taxa to be stressed by changes in temperature in order to detect early effects of changing temperatures and flow regimes.
Acknowledgments
The authors would like to thank the Region of Castilla-La Mancha for funding this research through PREG06-027 and our fellow researchers at CREA (UCLM). Additional thanks go to Mark Prince for help with the statistical analyses and Alain Maasri, Richard J. Horwitz and Narcis Prat for their comments on the manuscript.
Disclosure statement
There is no conflict of interest related with this research.
Additional information
Funding
Notes on contributors
Stefanie A. Kroll
Stefanie A. Kroll is an assistant research professor in the Drexel University Department of Biodiversity, Earth and Environmental Sciences and the Project Science Director of the Delaware River Watershed Initiative. Dr. Kroll oversees stream monitoring and research for this collaboration between over 50 nonprofit groups working in watershed conservation. She is on Advisory Boards for the Raritan Headwaters Association and the Tookany-Tacony-Frankford Watershed Partnership. She also works with Academy of Natural Sciences’ Women In Natural Sciences program.
Neil H. Ringler
Neil H. Ringler is Vice Provost and Executive Director of ESF Onondaga Lake Science Center. He has been named a Distinguished Teaching Professor and has served as Chairman of the Department of Environmental and Forest Biology at SUNY-ESF. Dr. Ringler is on the Editorial Board for the Journal Ecology of Freshwater Fish and the Board of Directors of Upstate Freshwater Institute.
Maria de la Cruz Cano Costa
Maria de la Cruz Cano Costa was a research technician at University of Castilla-La Mancha for over 7 years.
Jorge De las Heras Ibañez
Jorge De las Heras Ibáñez is a professor in the Department Producción Vegetal at the University of Castilla-La Mancha, Albacete campus. Dr. De las Heras Ibañez is Director of the research group ECOFOR as well as the Regional Water Research Center (CREA). He has served as the Dean of the Pharmacy School at UCLM.
References
- Agencia Estatal Meteorologico; Spanish State Meteorological Agency (AEMET). 2012. Spanish meteorological agency. Data provided upon request. Accessed July 2009.
- Arai R, Nukazawa K, Kazama S, Takemon Y. 2015. Variation in benthic invertebrate abundance along thermal gradients within headwater streams of a temperate basin in Japan. Hydrobiologia. 762:55–63.
- Araújo MB, Peterson AT. 2012. Uses and misuses of bioclimatic envelope modeling. Ecology. 93:1527–1539.
- Arnell NW. 1999a. Climate change and global water resources. Glob Environ Change. 9:S31–S49.
- Arnell NW. 1999b. The effect of climate change on hydrological regimes in Europe: a continental perspective. Glob Environ Change. 9:5–23.
- Arnell NW. 2004. Climate change and global water resources: SRES emissions and socio-economic scenarios. Glob Environ Change. 14:31–52.
- Arribas P, Velasco J, Abellán P, Sánchez-Fernández D, Andujar C, Calosi P, Millán A, Ribera I, Bilton DT. 2012. Dispersal ability rather than ecological tolerance drives differences in range size between lentic and lotic water beetles (Coleoptera: Hydrophilidae). J Biogeogr. 39:984–994.
- Barbour MT, Gerritsen J, Snyder BD, Stribling JB. 1999. Rapid bioassessment protocols for use in streams and wadeable rivers. Washington (DC): U.S. Environmental Protection Agency; Office of Water.
- Bates BC, Kundzewicz ZW, Wu S, Palutikof JP. 2008. Climate change and water technical paper of the intergovernmental panel on climate change (Geneva: IPCC Secretariat). Clim Change. 95:96.
- Boix-Fayos C, Calvo-Cases A, Imeson AC, Soriano-Soto MD, Tiemessen IR. 1998. Spatial and short-term temporal variations in runoff, soil aggregation and other soil properties along a Mediterranean climatological gradient. Catena. 33:123–138.
- Bolle HJ. 2012. Mediterranean climate: variability and trends. New York (NY): Springer Science & Business Media.
- Bonada N, Doledec S, Statzner B. 2007. Taxonomic and biological trait differences of stream macroinvertebrate communities between Mediterranean and temperate regions: implications for future climatic scenarios. Glob Change Biol. 13:1658–1671.
- Bonada N, Prat N, Munné A, Rieradevall M, Alba-Tercedor J, Álvarez M, Avilés J, Casas J, Jáimez-Cuéllar P, Mellado A, et al. 2002. Criterios para la selección de condiciones de referencia en los ríos mediterráneos. Resultados del proyecto GUADALMED [Criteria for selection of reference conditions in Mediterranean rivers. Results of the GUADALMED Project]. Limnetica. 21:99–114.
- Bonada N, Resh VH. 2013. Mediterranean-climate streams and rivers: geographically separated but ecologically comparable freshwater systems. Hydrobiologia. 719:1–29.
- Bonada N, Zamora-Muñoz C, Rieradevall M, Prat N. 2004. Trichoptera (Insecta) collected in Mediterranean river basins of the Iberian Peninsula: taxonomic remarks and notes on ecology. Graellsia. 60:41–69.
- Bovee KD. 1982. Instream flow methodology. St. Paul (MN): U.S. Fish and Wildlife Service. FWS/OBS-82-26. Available from: http://www.dnr.state.mn.us/waters/surfacewater_section/stream_hydro/instrflow.html
- Brittain JE. 1982. Biology of mayflies. Annu Rev Entomol. 27:119–147.
- Burgmer T, Hillebrand H, Pfenninger M. 2007. Effects of climate-driven temperature changes on the diversity of freshwater macroinvertebrates. Oecologia. 151:93–103.
- Chadwick MA, Dobberfuhl DR, Benke AC, Huryn AD, Suberkropp K, Thiele JE. 2006. Urbanization affects stream ecosystem function by altering hydrology, chemistry, and biotic richness. Ecol Appl. 16:1796–1807.
- Chown SL, Duffy GA, Sørensen JG. 2015. Upper thermal tolerance in aquatic insects. Curr Opin Insect Sci. 11:78–83.
- Christensen JH, Christensen OB. 2007. A summary of the PRUDENCE model projections of changes in European climate by the end of this century. Clim Change. 81:7–30.
- Cid N, Ibáñez C, Prat N. 2008. Life history and production of the burrowing mayfly Ephoron virgo (Olivier, 1791) (Ephemeroptera: Polymitarcyidae) in the lower Ebro river: a comparison after 18 years. Aquat Insects. 30:163–178.
- Corine Land Cover Database. 2000. Copenhagen: European Environment Agency. Accessed July 2009. Available from: http://land.copernicus.eu/pan-european/corine-land-cover/clc-2000.
- Dallas HF, Rivers-Moore NA. 2012. Critical thermal maxima of aquatic macroinvertebrates: towards identifying bioindicators of thermal alteration. Hydrobiologia. 679:61–76.
- De Castro M, Gallardo C, Calabria S. 2004. Regional IPCC projections until 2100 in the Mediterranean area. In: Marquina A, editor. Environmental Challenges in the Mediterranean 2000–2050. Dordrecht: Springer. NATO Science Series (Series IV: Earth and Environmental Sciences), vol 37.
- De Figueroa JT, López-Rodríguez MJ, Lorenz A, Graf W, Schmidt-Kloiber A, Hering D. 2010. Vulnerable taxa of European Plecoptera (Insecta) in the context of climate change. Biodivers Conserv. 19:1269–1277.
- Doledec S, Lamouroux N, Fuchs U, Merigoux S. 2007. Modelling the hydraulic preferences of benthic macroinvertebrates in small European streams. Freshw Biol. 52:145–164.
- Domisch S, Araújo MB, Bonada N, Pauls SU, Jähnig SC, Haase P. 2013. Modelling distribution in European stream macroinvertebrates under future climates. Glob Change Biol. 19:752–762.
- Domisch S, Jähnig SC, Haase P. 2011. Climate-change winners and losers: stream macroinvertebrates of a submontane region in Central Europe. Freshw Biol. 56:2009–2020.
- Durance I, Ormerod SJ. 2007. Climate change effects on upland stream macroinvertebrates over a 25-year period. Glob Change Biol. 13:942–957.
- Extence CA, Balbi DM, Chadd RP. 1999. River flow indexing using British benthic macroinvertebrates: a framework for setting hydroecological objectives. Regul Rivers Res Manag. 15:543–574.
- Filipe AF, Lawrence JE, Bonada N. 2012. Vulnerability of stream biota to climate change in Mediterranean climate regions: a synthesis of ecological responses and conservation challenges. Hydrobiologia. 719:331–351.
- Gallardo C, Arribas A, Prego JA, Gaertner MA, De Castro M. 2001. Multi-year simulations using a regional-climate model over the Iberian Peninsula: current climate and doubled CO2 scenario. Q J R Meteorol Soc. 127:1659–1681.
- García-Valdés R, Zavala MA, Araújo MB, Purves DW. 2013. Chasing a moving target: projecting climate change-induced shifts in non-equilibrial tree species distributions. J Ecol. 101:441–453.
- Gasith A, Resh VH. 1999. Streams in Mediterranean climate regions: abiotic influences and biotic responses to predictable seasonal events. Annu Rev Ecol Syst. 30:51–81.
- Haidekker A, Hering D. 2008. Relationship between benthic insects (Ephemeroptera, Plecoptera, Coleoptera, Trichoptera) and temperature in small and medium-sized streams in Germany: a multivariate study. Aquat Ecol. 42:463–481.
- Hampe A. 2004. Bioclimate envelope models: what they detect and what they hide. Glob Ecol Biogeogr. 13:469–471.
- Harper MP, Peckarsky BL. 2006. Emergence cues of a mayfly in a high-altitude stream ecosystem: potential response to climate change. Ecol Appl. 16:612–621.
- Hering D, Moog O, Sandin L, Verdonschot PF. 2004. Overview and application of the AQEM assessment system. Hydrobiologia. 516:1–20.
- Hering D, Schmidt-Kloiber A, Murphy J, Lücke S, Zamora-Munoz C, López-Rodríguez MJ, Huber T, Graf W. 2009. Potential impact of climate change on aquatic insects: a sensitivity analysis for European caddisflies (Trichoptera) based on distribution patterns and ecological preferences. Aquat Sci. 71:3–14.
- Hickling R, Roy DB, Hill JK, Thomas CD. 2005. A northward shift of range margins in British Odonata. Glob Change Biol. 11:502–506.
- Hogg ID, Williams DD. 1996. Response of stream invertebrates to a global-warming thermal regime: an ecosystem-level manipulation. Ecology. 77:395–407.
- Horcas R, Rasilla D, Fernández-García F. 2001. Temperature variations and trends in the Segura River Basin. An exploratory analysis. In: Brunet M, Lopez Bonillo D, editors. Detecting and modelling regional climate change. New York (NY): Springer; p. 133–142.
- Howard C, Stephens PA, Pearce-Higgins JW, Gregory RD, Willis SG. 2014. Improving species distribution models: the value of data on abundance. Methods Ecol Evol. 5:506–513.
- Howe RW. 1967. Temperature effects on embryonic development in insects. Annu Rev Entomol. 12:15–42.
- Hurrell JW. 1995. Decadal trends in the North Atlantic Oscillation: regional temperatures and precipitation. Science. 269:676–679.
- IPCC (Intergovernmental Panel on Climate Change). 2013. Climate change 2013: the physical science basis. Contribution of working group I to the Fifth Assessment Report of the Intergovernmental Panel on Climate Change In: Stocker TF, Qin Q, Plattner G-K, Tignor M, Allen SK, Boschung J, Nauels A, Xia Y, Bex V Midgley PM, editors. Fifth Assessment Report of the Intergovernmental Panel on Climate Change. Cambridge and New York (NY): Cambridge University Press; p. 1535.
- Jáimez-Cuéllar P, Vivas S, Bonada N, Robles S, Mellado A, Álvarez M, Avilés J, Casas J, Ortega M, Pardo I, et al. 2002. Protocolo GUADALMED (PRECE). Limnetica. 21:187–204.
- Kearney M, Porter W. 2009. Mechanistic niche modelling: combining physiological and spatial data to predict species’ ranges. Ecol Lett. 12:334–350.
- Keating KA, Cherry S. 2004. Use and interpretation of logistic regression in habitat-selection studies. J Wildl Manag. 68:774–789.
- Kingston DG, Taylor RG. 2010. Sources of uncertainty in climate change impacts on river discharge and groundwater in a headwater catchment of the Upper Nile Basin, Uganda. Hydrol Earth Syst Sci. 14:1297–1308.
- Kroll SA, Cano M de la C, de las Heras J, Navarro Llacer C. 2009. The influence of land use on water quality and macroinvertebrate biotic indices in rivers within Castilla-La Mancha (Spain). Limnetica. 28:203–214.
- Kroll SA, Ringler NH, De las Heras J, Goméz-Alday JJ, Moratalla A, Briggs RD. 2013. Analysis of anthropogenic pressures in the Segura Watershed (SE Spain), with a focus on inter-basin transfer. Ecohydrology. 6:878–888.
- Manel S, Dias J-M, Ormerod SJ. 1999. Comparing discriminant analysis, neural networks and logistic regression for predicting species distributions: a case study with a Himalayan river bird. Ecol Model. 120:337–347.
- Mellado A. 2005. The ecology of stream macroinvertebrate assemblages from the Segura River Basin (SE Spain). Environmental factors, spatio-temporal variability, indicator taxa, diversity trends, biological-ecological traits and applications for bioassessment [PhD thesis]. Murcia: University of Murcia.
- Merritt RW, Cummins KW, Berg MB. 2008. Introduction to the aquatic insects of North America. Dubuque: Kendall Hunt Publishing.
- Merritt RW, Ross DH, Larson GJ. 1982. Influence of stream temperature and seston on the growth and production of overwintering larval black flies (Diptera: Simuliidae). Ecology. 63:1322–1331.
- Milly PCD, Dunne KA, Vecchia AV. 2005. Global pattern of trends in streamflow and water availability in a changing climate. Nature. 438:347–350.
- Mohseni O, Stefan HG. 1999. Stream temperature/air temperature relationship: a physical interpretation. J Hydrol. 218:128–141.
- Moreno JM, De la Rosa D, Zazo C. 2005. Evaluación preliminar de los impactos en España por efecto del cambio climático [Preliminary evaluation of the effects of climate change in Spain]. Madrid: Consejo Superior de Investigaciones Científicas. Boletín CF+S 38/39. Available from: http://digital.csic.es/handle/10261/79951
- Nakano S, Miyasaka H, Kuhara N. 1999. Terrestrial–aquatic linkages: riparian arthropod inputs alter trophic cascades in a stream food web. Ecology. 80:2435–2441.
- Nakano S, Murakami M. 2001. Reciprocal subsidies: dynamic interdependence between terrestrial and aquatic food webs. Proc Natl Acad Sci. 98:166–170.
- Navarro-Llácer C. 2006. Estado ecológico de los ríos de Castilla-La Mancha [Ecological status of the rivers of Castilla-La Mancha] [ doctoral dissertation]. Albacete: University of Castilla-La Mancha.
- Navarro-Llácer C, Baeza D, de las Heras J. 2010. Assessment of regulated rivers with indices based on macroinvertebrates, fish and riparian forest in the southeast of Spain. Ecol Indic. 10:935–942.
- Pearson RG, Dawson TP. 2003. Predicting the impacts of climate change on the distribution of species: are bioclimate envelope models useful? Glob Ecol Biogeogr. 12:361–371.
- Peckarsky BL, Taylor BW, McIntosh AR, McPeek MA, Lytle DA. 2001. Variation in mayfly size at metamorphosis as a developmental response to risk of predation. Ecology. 82:740–757.
- Peng C-YJ, Lee KL, Ingersoll GM. 2002. An introduction to logistic regression analysis and reporting. J Educ Res. 96:3–14.
- Poff NL, Allan JD, Bain MB, Karr JR, Prestegaard KL, Richter BD, Sparks RE, Stromberg JC. 1997. The natural flow regime. BioScience. 47:769–784.
- Poff NL, Brinson MM, Day JW. 2002. Aquatic ecosystems and global climate change: potential impacts on inland freshwater and coastal wetland ecosystems in the United States, Vol. 44. Arlington (VA): Pew Center on Global Climate Change.
- Rahel FJ, Olden JD. 2008. Assessing the effects of climate change on aquatic invasive species. Conserv Biol. 22:521–533.
- Railsback SF. 2016. Why it is time to put PHABSIM out to pasture?. Fish Mag. 41:720–725.
- Rawlings JO, Pantula SG, Dickey DA. 1998. Applied regression analysis: a research tool. New York (NY): Springer Science & Business Media.
- Romey WL, Rossman DS. 1995. Temperature and hunger alter grouping trade-offs in whirligig beetles. Am Midl Nat. 134:51–62.
- Shaunbhogue A, Gore AP. 1987. Using logistic regression in ecology. Curr Sci. 56:933–935.
- Southwood TRE. 1977. Habitat, the templet for ecological strategies? J Anim Ecol. 337–365.
- Sweeney BW. 1993. Effects of streamside vegetation on macroinvertebrate communities of White Clay Creek in eastern North America. Proc Acad Nat Sci Phila. 144:291–340.
- Sweeney BW, Jackson JK, Funk DH. 1995. Semivoltinism, seasonal emergence, and adult size variation in a tropical stream mayfly (Euthyplocia hecuba). J North Am Benthol Soc. 14:131–146.
- Sweeney BW, Jackson JK, Newbold JD, Funk DH. 1992. Climate change and the life histories and biogeography of aquatic insects in eastern North America. In: Firth P, Fisher SG, editors. Global climate change and freshwater ecosystems. New York (NY): Springer; p. 143–176.
- Swift LW, Cunningham GB, Douglass JE. 1988. Climatology and hydrology. In: Swank WT, Crossley DA, editors. Forest hydrology and ecology at Coweeta. Ecological studies (analysis and synthesis), Vol. 66. New York (NY): Springer.
- Tachet H, Richoux P, Bournaud M, Usseglio-Polatera P. 2010. Invertébrés d'eau douce: systématique, biologie, écologie. Paris: CNRS éditions.
- Tang C, Li Y, Jiang P, Yu Z, Acharya K. 2015. A coupled modeling approach to predict water quality in Lake Taihu, China: linkage to climate change projections. J Freshw Ecol. 30:59–73.
- Trexler JC, Travis J. 1993. Nontraditional regression analyses. Ecology. 74:1629–1637.
- UNCED (United Nations Conference on Environment and Development). 1993. Agenda 21: programme of action for sustainable development. New York (NY): United Nations, Publication 93.1.11.
- Underwood EC, Viers JH, Klausmeyer KR, Cox RL, Shaw MR. 2009. Threats and biodiversity in the Mediterranean biome. Divers Distrib. 15:188–197.
- Vannote RL, Minshall GW, Cummins KW, Sedell JR, Cushing CE. 1980. The river continuum concept. Can J Fish Aquat Sci. 37:130–137.
- Vannucchi PE, Peralta-Maraver I, de Figueroa JMT, López-Rodríguez MJ. 2017. Dynamics of the macroinvertebrate community and food web of a Mediterranean stream. J Freshw Ecol. 32:223–239.
- Velasco J, Millan A. 1998. Insect dispersal in a drying desert stream: effects of temperature and water loss. Southwest Nat. 43:80–87.
- Vicuña S, Garreaud RD, McPhee J. 2011. Climate change impacts on the hydrology of a snowmelt driven basin in semiarid Chile. Clim Change. 105:469–488.
- Vidal-Abarca MR. 1990. Rivers of arid and semiarid basins: a comparative and synthesis ecological view. Sci Gerund Spain. http://agris.fao.org/agris-search/search.do?recordID=ES9200054
- Vidal-Abarca MR, Suárez ML, Ramírez-Díaz L. 1992. Ecology of Spanish semiarid streams. Limnetica. 8:l6.
- Vörösmarty CJ, McIntyre PB, Gessner MO, Dudgeon D, Prusevich A, Green P, Glidden S, Bunn SE, Sullivan CA, Liermann CR, et al. 2010. Global threats to human water security and river biodiversity. Nature. 467:555–561.
- Wallace JB, Webster JR. 1996. The role of macroinvertebrates in stream ecosystem function. Annu Rev Entomol. 41:115–139.
- Wilson R. 2009. Impacts of Climate Change on European Invertebrates, with reference to the vulnerability of Bern Convention species. Proceedings of the 29th Meeting of the Standing Committee Convention on the Conservation of European Wildlife and Natural Habitats. Strasbourg: Council of Europe. Biodiversity and climate change: Reports and guidance developed under the Bern Convention.
- Wright LL, Mattice JS, Beauchamp JJ. 1982. Effect of temperature and sex on growth patterns in nymphs of the mayfly Hexagenia hilineata in the laboratory. Freshw Biol. 12:535–545.
- Xu ZX, Zhao FF, Li JY. 2009. Response of streamflow to climate change in the headwater catchment of the Yellow River basin. Quat Int. 208:62–75.