Abstract
Lakeshore areas provide important habitat for aquatic invertebrates in shallow lakes. However, these zones are prone to anthropogenic disturbances that include shoreline development, urbanization, nutrient inputs, agricultural and(or) recreational use. Among recreational uses, public access sites are often developed to accommodate boaters and facilitate lake access via boat ramps. Although the ‘foot print’ associated with boat ramp structures can be relatively small compared to total shoreline coverage, little is known about the relative effects of boating activity on littoral zones and macroinvertebrate communities. In this study, we assess the relative impact of boating-related activities on aquatic macroinvertebrate communities at high-use (primary) and low-use (secondary) boat ramps on five glacial lakes of eastern South Dakota. Macroinvertebrate assemblages were dominated by few taxa that included Chironomidae, Corixidae, Caenidae, and Amphipoda. Moreover, boat ramp use did not influence abundance or diversity of aquatic macroinvertebrates. Habitat, specifically substrate composition, was also similar between primary and secondary boat ramps despite more intense use associated with primary boat ramps. Our results support related findings that aquatic invertebrate assemblages in the Prairie Pothole Region are structured by regional environmental variability (climate, habitat, and water quality) resulting in communities with characteristically low diversity and tolerant taxa. This may explain why small-scale disturbance associated with boating activity has no discernable effect on macroinvertebrate assemblages in glacial lakes of the Prairie Pothole Region.
Introduction
Powerboating is a popular, recreational activity on many lakes and rivers. In areas that experience high boating-related activity, physical disturbance from boat wakes can affect near-shore habitats and associated biota (Liddle and Scorgie Citation1980; Mosisch and Arthinton Citation1998; Hardiman and Burgin Citation2011). Boat wakes can impact aquatic macroinvertebrates by disturbing sediments (Bishop and Chapman Citation2004) or dislodging them from macrophytes or other structures (Bishop Citation2005; Bishop Citation2008). Disturbance caused by wave and propeller action can also contribute to shoreline erosion, decreased water clarity, and increased nutrient concentrations via sediment suspension (Liddle and Scorgie Citation1980; Mosisch and Arthinton Citation1998; Hardiman and Burgin Citation2011). Several U.S. states prohibit power loading of boats onto trailers because prop wash can erode the lake bed and re-distribute sediments around boat ramps. Additional boating-related impacts to aquatic ecosystems include contamination by fuel, oil, and exhaust emissions as well as introduction and spread of non-native organisms for which boats can serve as vectors (Liddle and Scorgie Citation1980; Mosisch and Arthinton Citation1998; Hardiman and Burgin Citation2011).
Public boat ramps provide important lake access for recreational activities such as boating, water skiing, and angling. Indeed, boat ramp construction and maintenance represents an important part of annual operating budgets for many fish and wildlife agencies. Parking lots and amenities such as restrooms and fish cleaning stations provide benefits that attract boating enthusiasts to public boat ramps (Siderelis et al. Citation1995; Reed-Andersen et al. Citation2000). In turn, public access areas can concentrate boating-related disturbances compared to other near-shore areas of a lake, such as private or fee-based boat access.
In this study, we evaluate effects of boating activity on aquatic invertebrate communities in natural lakes. Aquatic invertebrate abundance and composition were compared between boat ramps with two levels of boating intensity: high versus low use. We postulated that at high-use (primary) boat ramps, aquatic invertebrate communities are negatively impacted by increased frequency and magnitude of disturbance compared to that of low use (secondary) boat ramps with similar physical characteristics (i.e. presence of a boat ramp). We measured abundance and diversity of aquatic macroinvertebrates as an index of boating-related disturbance because of their importance in aquatic food webs and their widespread use in bioassessment studies (Covich et al. Citation1999).
Materials and methods
Study area
Five glacial lakes in the Prairie Pothole Region (PPR) of eastern South Dakota were chosen for study and included lakes Kampeska, Madison, Pelican, Poinsett, and Thompson (). All five lakes are popular destinations for anglers, boaters, water skiers and other water sport enthusiasts (http://gfp.sd.gov/state-parks/directory). These study lakes are comparatively large relative to many glacial lakes in the region with surface areas ranging from 1131 to 5041 ha with maximum; water depths range from 2.4 to 6.1 m (Stueven and Stewart Citation1996). Private, lake-shore development varies appreciably among the lakes, with Lake Thompson being the least-developed (1.1 private boat docks/km) and Lake Madison the most-developed (19.8 private boat docks/km). The lakes are classified as eutrophic (trophic state index > 50; Carlson Citation1977) and regularly experience late-summer cyanobacterial blooms. Shoreline development indices range from 1.3 for Lake Poinsett to 2.9 for Pelican Lake. Due to relatively shallow water depths and long fetch (≥7 km), all lakes exhibit polymictic mixing and do not develop distinct thermoclines in summer months.
Sample site selection
Each lake had at least two, public-access boat ramps that could be classified as either a primary access or secondary access based on the frequency and magnitude of use. If more than one primary or secondary boat ramp was available at a lake, we randomly selected a boat ramp to sample. Boat ramps were classified based on location and size of boat ramp access, number of parking slips (>15 for primary boat ramps), and presence/absence of infrastructure facilities (). The relative intensity of boater use at each boat ramp was assessed using Google Earth imagery and corroborated by interviews with South Dakota Department of Game Fish and Parks conservation officers. Available imagery for June to September, 2003–2016 was examined for each lake and the maximum number of vehicles with boat trailers (VBT) was recorded ().
Table 1. Attributes of primary and secondary boat ramps for five glacial lakes in eastern South Dakota.
Habitat and invertebrate sampling
Sampling was conducted during the third week of September 2012. Physical habitat was quantified within a 1-m radius of each sample site by measuring substrate composition (% silt, sand, gravel, cobble, and boulder) and percent submerged vegetation coverage following guidelines outlined by Simonson et al. (Citation1994).
Aquatic macroinvertebrates were collected using two gear types; a D-frame sweep net, hereafter referred to as a sweep net, and a stovepipe sampler (Merritt et al. Citation1984). We used sweep nets to sample invertebrates in the water column (i.e. lentic). Our sampling technique involved using a 500-μm mesh sweep net (H × L [cm]: 34 × 50], to sweep a 2 m arc from the water surface to near bottom, and then back to the surface in order to sample the entire water column (Turner and Trexler Citation1997; Meyer et al. Citation2011). Stovepipe samplers (H:60 cm, diameter: 34 cm) were used to sample benthic macroinvertebrates at each site by pushing the sampler into the bottom substrate, then using a small dip net (H × L [cm]:12 × 14, mesh [mm]: 1.0) to collect invertebrates inside the sampler (Merritt et al. Citation1984). We collected five sweep net and five stove pipe samples at each site within 10 m of the outside edge of a boat ramp. To reduce bias associated with water depth, we collected invertebrates in water depths ranging from 50 to 70 cm at each site. Invertebrates collected from each sample were placed in 250–500 ml plastic bottles containing 90% ETOH and transported to South Dakota State University where they were sorted and identified using dissecting microscopes (Anderson Citation1959). Organisms were identified to subclass (i.e. Hirudinea, Oligochaeta), order (i.e. Amphipoda) or family (e.g. Caenidae, Chironomidae, Corixidae, Spaeriidae).
Statistical analysis
Paired t-tests were used to evaluate differences in substrate types between primary and secondary boat ramps. A Bonferroni adjustment (α = 0.01) was used to maintain a table-wide alpha level of 5% for the five substrate categories measured (Rice Citation1989). We compared invertebrate taxa richness between primary and secondary boat ramps using a paired t-test (Cody and Smith Citation2006). Macroinvertebrate community similarity was evaluated by comparing mean, taxa-specific abundance of invertebrates collected at primary boat ramps to those collected at secondary boat ramps. Pearson’s correlation coefficient was used as an index of community similarity (Krebs Citation1999) where a value of −1 indicates complete negative correlation between communities and +1 indicates complete positive correlation between communities (Sokal and Rohlf Citation1969). Macroinvertebrate taxa included in analyses were either fully aquatic or represented an aquatic life-stage (i.e. insects).
The influence of boat ramp type (i.e. primary or secondary) on macroinvertebrate composition across lakes was evaluated using multivariate analysis of variance (MANOVA) on log(x + 1) transformed data (Cody and Smith Citation2006). If a MANOVA test was significant (α < 0.05), we compared abundance of individual invertebrate taxa among lakes using a mixed-model analysis of variance test to identify those taxa contributing to the observed difference. All statistical analyses were performed using SAS software, version 9.4 (SAS Institute Inc. Citation2013).
Results
Substrate composition did not differ between primary and secondary boat ramps (). In general, sand and gravel were the dominant substrate types representing >80% of the substrate composition at boat ramps. Similarly, aquatic macrophytes were rarely encountered, representing <1.4% coverage at any site.
Table 2. Mean substrate composition at primary and secondary boat ramp sites for five eastern South Dakota glacial lakes.
Seven macroinvertebrate taxa (i.e. Amphipoda, Caenidae, Chironomidae, Corixidae, Hirudinea, Oligochaeta, and Sphaeriidae) collectively represented >88% of individuals collected with stovepipe samplers and >98% of individuals collected with sweepnets. Stovepipe samplers generally collected more taxa, especially benthic taxa (i.e. Caenidae, Chironomidae, and Hirudinea), whereas sweep nets collected fewer taxa but were selective for highly mobile littoral macroinvertebrates including Amphipoda and Corixidae. Several taxa were excluded from our analyses because they were semiaquatic (e.g. Collembola) or were found at only one site (e.g. Ostracoda, Planorbidae).
Mean taxa richness was similar among primary (5.8, n = 5, SE = 1.3) and secondary (6.4, n = 5, SE = 1.0) boat ramp sites for invertebrates collected using a stovepipe sampler (paired t-test, df = 4, t = 2.77, P = 0.73). Similarly, mean taxa richness for invertebrates collected using sweep nets was similar at primary (4.4, n = 5, SE = 0.9) and secondary (5.6, n = 5, SE = 1.0) boat ramp sites (df = 4, t = 2.77, P = 0.28; ). Invertebrate composition at primary boat ramps was similar to that observed at secondary boat ramps based on either stovepipe samples (MANOVA, Wilk's λ = 0.21, F7,2=1.08, P = 0.56) or sweep nets (Wilk's λ = 0.35, F6,3=0.90, P = 0.58). Similarly, mean abundance of invertebrates collected at primary boat ramps was significantly correlated with mean abundance of invertebrates captured at secondary boat ramps for stovepipe samples (correlation analysis; n = 47, r =0.56, P < 0.0001) and sweep nets (r = 0.67, P = 0.0001; ). Overall, we found similar habitat, macroinvertebrate abundance, and community similarity between primary and secondary boat ramps in glacial lakes.
Figure 2. Relationship between mean invertebrate abundance measured at primary and secondary boat ramps in five glacial lakes in eastern South Dakota (see ). Invertebrates were collected using a stovepipe sampler (solid circles, no./m2) or sweep net sampler (solid triangles, no./sweepnet).
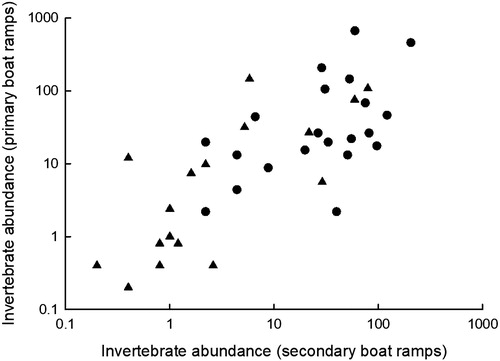
Table 3. Mean abundance of aquatic macroinvertebrates collected using a stovepipe sampler (no./m2) or sweep net (no./sweep) at primary and secondary boat ramps in five eastern South Dakota lakes.
Discussion
We found that littoral macroinvertebrate assemblages were not significantly influenced by boating-related activity at primary or secondary boat ramps. We expected to observe differences in macroinvertebrate abundance and diversity related to changes in habitat structure, namely benthic substrate used by invertebrates for shelter (Cummins and Merritt Citation1996). Instead, substrate composition was similar among sites and dominated by sand and gravel. Larger, primary boat ramps with greater parking capacity and amenities facilitated more intense use that did not result in changes to substrate composition or macroinvertebrate community structure. Our study was restricted to only one sampling period, but the sampling window coincided with the late summer boating season, and allowed us to assess potential impacts during a high-use period.
Few studies have attempted to document impacts of boating-related activity on littoral macroinvertebrates of natural lakes. A related study found family-level differences in macroinvertebrate assemblages between boat ramp sites and nearby control sites and attributed the differences to localized pollution from boating activity (Forster Citation2005). Other potential mechanisms known to impact littoral macroinvertebrates are related to physical disturbance. Boating activity related to launching and loading boats can temporarily suspend sediments around boat ramps (Moss Citation1977; Liddle and Scorgie Citation1980; Hilton and Philips Citation1982; Kuss et al. Citation1990) that can cause respiratory impairment in invertebrates (Murphy et al. Citation1995; Liddle Citation1998). In naturally turbid systems, however, it was shown that short-term exposure to high turbidity levels has no adverse effect on damselflies, mayflies, and caddisflies that are adapted to high sediment load in the water column (Suren et al. Citation2005). Boating activity is also known to displace aquatic invertebrates, but they can quickly re-colonize areas after disturbances (Bishop Citation2008). A likely reason why invertebrate communities were similar between primary and secondary boat ramps was the depauperate nature of invertebrate assemblages in the study lakes. Although the low taxonomic resolution in our analysis may have masked some variability in invertebrate composition, particularly for Chironomidae (Carter and Resh Citation2001; Jones Citation2008), this effect likely had little impact on our results given the relatively low number of invertebrate taxa collected (i.e. seven dominant taxa), many of which are typically represented by only a few species in the PPR (e.g. Amphipoda, Caenidae, Corixidae, Hirudinea, and Sphaeriidae).
Environmental conditions of the PPR tend to support aquatic invertebrate communities with limited diversity comprised of highly adaptive taxa (Tangen et al. Citation2003). Aquatic invertebrate communities of the PPR inhabit a mosaic of dynamic freshwater habitats (e.g. temporary wetlands, permanent wetlands, and lakes) that vary widely in salinity, water-level fluctuation regimes, temperature, and seasonal oxygen levels (Meding and Jackson Citation2003; Euliss et al. Citation2004). A study of thirty shallow lakes of the Nebraska Sandhills Region by Paukert and Willis (Citation2003) revealed that the lakes contained a depauperate macroinvertebrate assemblage, dominated by Gastropoda (68.9%), Chironomidae (18.9%), and Oligochaeta (8.0%).
Aquatic macroinvertebrate communities in the PPR have also proven resilient to large-scale anthropogenic changes, illustrating the robust nature of these communities to disturbance. Bataille and Baldassarre (Citation1993) found that aquatic invertebrates were abundant and widely distributed across three wetland types (i.e. seasonal, semi-permanent, and permanent) following a three-year drought, indicating the resilience of aquatic invertebrates in the region to disturbance. In an attempt to develop an aquatic macroinvertebrate index of biotic integrity for semi-permanent wetlands in North Dakota, Tangen et al. (Citation2003) found similar invertebrate communities across disturbance regimes ranging from 100% grassland to 100% row-crop agriculture. In the PPR climatic disturbances including drought and deluge periods may play a larger role in shaping invertebrate communities than land use. For example, Chironomidae communities of lowland Saskatchewan lakes were more heavily influenced by climatic conditions than agricultural or urban influences (Quinlan et al. Citation2002).
A major influence related to boating activity is wave action and sediment disturbance in near-shore areas of lakes. Similarly, large, shallow glacial lakes in our study typically experience significant wind/wave action owing to natural, climatic conditions in the PPR. Thus, invertebrate communities in these lakes are likely well-adapted to wind/wave action in littoral areas and may represent poor indicators of shoreline disturbance associated with boating activity.
Given the relatively small scale of shoreline disturbance, any impacts related to boat ramps and associated activities are likely localized and temporary. Conversely, environmental conditions such as climate, water quality, and(or) habitats of the PPR likely overwhelm any influence that small-scale shoreline disturbance would have on aquatic invertebrate communities. Aquatic invertebrate communities in glacial lakes may be well-adapted to a large range of environmental conditions, and thus not affected by relatively small-scale disturbances associated with recreational boating activity near public-use boat ramps. Our investigation of the effects of boating activity revealed that resident macroinvertebrate communities were similar between low and high use areas. Standardized sampling across multiple sampling periods would be needed to more fully assess the influence of boating activity and environmental factors on invertebrate communities.
Notes on contributors
Bradley Smith is a fish biologist with the U.S. Fish and Wildlife Service based out of Green Bay, WI and primarily works on issues related to aquatic ecology and aquatic invasive species in the Laurentian Great Lakes.
Steven Chipps is the Unit Leader, U.S. Geological Survey, South Dakota Cooperative Fish and Wildlife Research, and is an adjunct professor in the Department of Natural Resource Management at South Dakota State University. His primary interests include feeding ecology and energetics of fishes.
Jeffrey Grote is a fisheries biologist at NOAA Point Adams Research Station in Hammond, OR.
Jake Mecham is an aquatic biologist with the Utah Division of Wildlife Resources in Green River, UT.
Tanner Stevens is a fisheries biologist and assistant area supervisor with the Minnesota Department of Natural Resources in Hutchinson, MN.
Tobias Rapp is a fisheries scientist at the Mecklenburg-Vorpommern Research Centre for Agriculture and Fisheries in Germany. His research interests include aquaculture, fisheries ecology, and fisheries management.
Acknowledgements
This project was made possible by the Department of Natural Resource Management and the USGS, South Dakota Cooperative Fish and Wildlife Research Unit at South Dakota State University. The South Dakota Cooperative Fish and Wildlife Research Unit is jointly sponsored by the U.S. Geological Survey, South Dakota Department of Game, Fish and Parks, South Dakota State University, the Wildlife Management Institute, and the U.S. Fish and Wildlife Service. Any use of trade names is for descriptive purposes only and does not imply endorsement by the U.S. Government.
Disclosure statement
No potential conflict of interest was reported by the authors.
Funding
Support for this project was provided by the Department of Natural Resource Management at South Dakota State University. Logistical support was provided by the USGS, South Dakota Cooperative Fish and Wildlife Research Unit at South Dakota State University.
References
- Anderson RO. 1959. A modified flotation technique for sorting bottom fauna samples. Limnol Oceanogr. 4(2):223–225.
- Bataille KJ, Baldassarre GA. 1993. Distribution and abundance of aquatic macroinvertebrates following drought in three prairie pothole wetlands. Wetlands. 13(4):260–269.
- Bishop MJ. 2005. Compensatory effects of boat wake and dredge spoil disposal on assemblages of macroinvertebrates. Estuaries. 28(4):510–518.
- Bishop MJ. 2008. Displacement of epifauna from seagrass blades by boat wake. J Exp Mar Biol Ecol. 354(1):111–118.
- Bishop MJ, Chapman MG. 2004. Managerial decisions as experiments: an opportunity to determine the ecological impact of boat-generated waves on microbenthic infauna. Estuar Coast Shelf S. 61(4):613–622.
- Carlson RE. 1977. A trophic state index for lakes. Limnol Oceanogr. 22(2):361–369.
- Carter JL, Resh VH. 2001. After site selection and before data analysis: sampling, sorting, and laboratory procedures used in stream benthic macroinvertebrate monitoring programs by U.S.A. state agencies. J N Am Benthol Soc. 20(4):658–682.
- Cody RP, Smith JK. editors 2006. Applied statistics and the SAS programming language, 5th edition. Upper Saddle River, New Jersey: Pearson Prentice Hall.
- Covich AP, Palmer MA, Crowl TA. 1999. The role of benthic invertebrate species in freshwater ecosystems: zoobenthic species influence energy flows and nutrient cycling. BioScience. 49(2):119–127.
- Cummins KW, Merritt RW. 1996. Ecology and distribution of aquatic insects. In: Merritt RW, Cummins KW, editors. An introduction to the aquatic insects of North America. Dubuque, Iowa: Kendall/Hunt. p. 74–86.
- Euliss NH, LaBaugh JW, Fredrickson LH, Mushet DM, Laubhan MK, Swanson GA, Winter TC, Rosenberry DO, Nelson RD. 2004. The wetland continuum: a conceptual framework for interpreting biological studies. Wetlands. 24(2):448–458.
- Forster LE. 2005. Effect of boat launches on benthic macroinvertebrates in a lentic system. Ecol Res. 7:8–12.
- Hardiman N, Burgin S. 2011. The direct physical, chemical, and biotic impacts on Australian coastal waters due to recreational boating. Biodivers Conserv. 20:683–701.
- Hilton J, Phillips GL. 1982. The effect of boat activity on turbidity in a shallow broadland river. J Appl Ecol. 19(1):143–150.
- Jones FC. 2008. Taxonomic sufficiency: the influence of taxonomic resolution on freshwater bioassessments using benthic macroinvertebrates. Environ Rev. 16:45–69.
- Krebs CJ. 1999. Ecological methodology. Menlo Park, CA: Benjamin Cummings.
- Kuss E, Graefe A, Vaske J. 1990. Visitor impact management, Vol. I. Washington, DC: National Parks and Conservation Association.
- Liddle M. 1998. Recreation ecology – the ecological impact of outdoor recreation and ecotourism. London: Chapman and Hall.
- Liddle MJ, Scorgie HRA. 1980. The effects of recreation on freshwater plants and animals: a review. Biol Conserv. 17(3):183–206.
- Meding ME, Jackson LJ. 2003. Biotic, chemical, and morphometric factors contributing to winter anoxia in prairie lakes. Limnol Oceanogr. 48(4):1633–1642.
- Merritt WK, Cummins W, Resh VH. 1984. Collecting, sampling, and rearing methods for aquatic insects. In: Merritt RW, Cummins KW, editors. An introduction to the aquatic insects of North America. Dubuque, IA: Kendall/Hunt. p. 11–26.
- Meyer CK, Peterson SD, Whiles MR. 2011. Quantitative assessment of yield, precision, and cost-effectiveness of three wetland invertebrate sampling techniques. Wetlands. 31(1):101–112.
- Mosisch TD, Arthinton AH. 1998. The impacts of power boating and water skiing on lakes and reservoirs. Lakes Reserv Res Manag. 3(1):1–17.
- Moss B. 1977. Conservation problems in the Norfolk Broads and rivers of East Anglia, England – phytoplankton, boats and the causes of turbidity. Biol Cons. 12(2):95–114.
- Murphy KJ, Willby NJ, Eaton JW. 1995. Ecological impacts and management of boat traffic on navigable inland waterways. In: Harper DM, Ferguson AJD, editors. The ecological basis for river management. Chichester: John Wiley and Sons. p. 427–442.
- Paukert CP, Willis DW. 2003. Aquatic invertebrate assemblages in shallow prairie lakes: fish and environmental influences. J Freshw Ecol. 18(4):523–536.
- Quinlan R, Leavitt PR, Dixit AS, Hall RI, Smol JP. 2002. Landscape effects of climate, agriculture, and urbanization on benthic invertebrate communities of Canadian prairie lakes. Limnol Oceanogr. 47(2):378–391.
- Reed-Andersen TE, Bennett M, Jorgensen BS, Lauster G, Lewis DB, Nowacek D, Riera JL, Sanderson BL, Stedman R. 2000. Distribution of recreational boating across lakes: do landscape variables affect recreational use? Freshwater Biol. 43:439–448.
- Rice WR. 1989. Analyzing tables of statistical tests. Evolution. 43(1):223–225.
- SAS Institute Inc 2013. SAS 9.4 Help and Documentation, Cary, NC.
- Siderelis C, Brothers G, Rea P. 1995. A boating choice model for the variation of lake access. J Leis Res. 27(3):264–282.
- Simonson TD, Lyons J, Kanehl PD. 1994. Guidelines for evaluating fish habitat in Wisconsin streams. St. Paul, MN: United States Department of Agriculture, General Technical Report NC-164.
- Sokal RR, Rohlf FJ, editors. 1969. Biometry. San Francisco, CA: W. H. Freeman and Company.
- Stueven E, Stewart WC. 1996. South Dakota lakes assessment final report. Pierre, SD: South Dakota Department of Environment and Natural Resources.
- Suren AM, Martin ML, Smith BJ. 2005. Short-term effects of high suspended sediments on six common New Zealand stream invertebrates. Hydrobiologia. 548(1):67–74.
- Tangen BA, Butler MG, Ell MJ. 2003. Weak correspondence between macroinvertebrate assemblages and land use in prairie pothole region wetlands, USA. Wetlands. 23:104–115.
- Turner AM, Trexler JC. 1997. Sampling aquatic invertebrates from marshes: evaluating the options. J N Am Benthol Soc. 16(3):694–709.