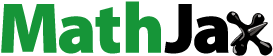
Abstract
The knowledge of food web spatial heterogeneity is important for ecologists and lake managers to understand ecosystem complexity and lake management. Lake Taihu, a large shallow eutrophic lake in China, has two distinct zones: algae- and macrophyte-dominated. In this study, we assessed the spatial heterogeneity of food webs in the two lake zones using stable isotope analysis and isotope mixing model. The basal sources and consumers showed significant differences in δ13C and δ15N ratios between the two lake zones, except for the filter-feeding fishes. Overall, more δ13C-depleted and δ15N-enriched ratios were found in the algae- than the macrophyte-dominated zones for basal sources and consumers. Although the consumers in the algae-dominant zone had higher average δ15N values, the food web of the macrophyte-dominated zone had longer food chain length and more diverse trophic linkages. These spatial differences may have resulted from resource availability and environmental stress of Lake Taihu ecosystem. Factors associated with spatial trophic heterogeneity should be considered for the management and restoration of this shallow eutrophic lake.
1. Introduction
Food web is the network of feeding interactions and its structure may affect the entire ecosystem functions (Thompson et al. Citation2012). Numerous researchers have found that complex spatial heterogeneity existed in food web (Scharroba et al. Citation2016; Hetherington et al. Citation2017; Kopec et al. Citation2019). Theoretical analyses and modeling exercises suggest that there are two main factors shape the spatial heterogeneity of food web structure: resource availability and environmental stress (Anderson and Cabana Citation2009). Resource availability hypothesis states that the wider carbon range of basal sources may bring more complex pathways for the transfer of matter and energy (Wang and Brose Citation2018). On the other hand, food chain length would decline with increased stress, due to reduce the energy flow to consumers or alter species compositions (Vander Zanden et al. Citation1999). These hypothesis explain the spatial heterogeneity formation mechanisms of food web structure, however, the related empirical evidence is still need to be supplemented.
Large shallow lakes have been recognized as one of the most productive ecosystems. They provide different ecosystem services to humans such as maintaining biodiversity, providing water sources and controlling floods (Woodward Citation2009). Due to variable geomorphological condition, hydrodynamic complexity, and human disturbance of different lake zones or sub-basins, multiple types of stable states often coexist in a single lake (Scheffer and Jeppesen Citation2007; Janssen et al. Citation2014). Some lake zones may be dominated by abundant macrophytes with clean water while others may be dominated by dense algae blooms with turbid water (van Nes et al. Citation2007; Kuiper et al. Citation2017). These create more complexities in the study of large shallow lakes, especially those related to eutrophication management.
The stable states in shallow lakes provide an unique perspective for exploring the spatial heterogeneity of food web strucutre. The various basal sources composition and environment stress (e.g., pollutant inputs or water depth) of different stable states within large shallow lakes may affect the formation of corresponding food webs (Hobbs et al. Citation2014). However, many food web studies of stable states in shallow lakes attempted to explore the overall ecosystem characteristics, and ignored the spatial differences of food web structure (Kong et al. Citation2017; Lopez Moreira Mazacotte et al. Citation2018). Indeed, exploring the spatial heterogeneity of the food web could help to explore the trophic structure and energy pathways under multiple stable states in one complex shallow lake ecosystem.
Stable isotope technologies have been used as powerful and complementary tools for understanding the food web structure and energy pathways in aquatic ecosystems (Syvaranta et al. Citation2011; Wang et al. Citation2013). Both δ13C and δ15N can reveal the assimilated portion of consumers’ diets at the time of synthesis (France Citation1995). On the one hand, δ13C changes little when it fractionates through the food chain, so it could be used to identify the food source (Mao et al. Citation2016). On the other hand, δ15N of the predator increases approximately 2‰–3.5‰ relative to its prey, and is usually used to estimate the trophic positions of species (Cornelissen et al. Citation2018). Isotope mixing models based on Bayesian procedures can be used to calculate the relative contribution of the multiple potential food sources to consumers (Solomon et al. Citation2011). Therefore, stable isotope analysis and isotope mixing models provide a possible strategy for quantitatively illustrating the food web structure for a large shallow lake.
In this study, we used stable isotope analysis and an isotope mixing model to evaluate the spatial heterogeneity of the food web structure in a large shallow eutrophic lake, which has two coexisting stable states: the algae- and macrophyte-dominated stable states in two different lake zones. Our main goals were to (1) examine and compare the spatial heterogeneity of carbon and nitrogen stable isotope ratios of basal food sources and consumers; (2) elucidate the food chain length and key trophic links of energy flow through food webs in the two different lake zones. Stable isotope data will help to understand the role of resource availability and environmental stress in shaping food web structure of these two coexisting stable states.
2. Materials and methods
2.1. Study area
Lake Taihu, the third largest freshwater lake in China, is located in the lower reaches of the Yangtze River (). It has a surface area of 2,338 km2, with a length from north to south of 68.5 km, and a width from east to west of 56 km. The average water depth of the whole lake was 1.9 m, while the average water depth of the eastern bay is 0.9 m (Qin et al. Citation2004). As a shallow lake, its water volume was approximately 51.5 × 108 m3 (Qu et al. Citation2011). Influenced by the subtropical monsoon climate, the annual mean temperature of Lake Taihu varies from 14.9 to 16.2 °C, and its annual average precipitation is between 1,000 and 1,400 mm (Li et al. Citation2010).
Figure 1. Lake Taihu and sampling locations (ST1, ST2 and ST3 are in the algae-dominated zone and ST4, ST5 and ST6 are in the macrophyte-dominated zone) in China.
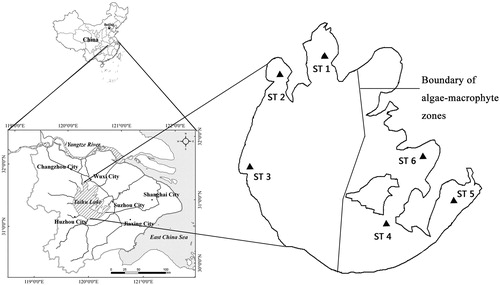
Two distinct lake zones under different eutrophication conditions coexist in Lake Taihu. The northwestern zone is an algae-dominated zone that has been largely affected by industrial and agricultural pollution from its catchment (Xu et al. Citation2016). Since the late 1980s, this lake zone has been highly eutrophic with frequent algal blooms (Guo Citation2007). In contrast, the southeastern zone is macrophyte-dominated and located in the outflow area of the whole lake (Qin et al. Citation2007). Numerous submerged plants are present in this lake zone. The large area of these submerged plants helps clean the aquaculture-related pollution in this zone (Hu et al. Citation2010). The limnological parameters of these two lake zones are shown in .
Table 1. Limnological characteristics of the two lake zones in Lake Taihu.
2.2. Sample collection and process
Field sampling was conducted from September 12–16, 2010 from six sampling stations in Taihu Lake (). The first three stations (ST1–3) were in the algae-dominated zone, while the other three stations (ST 4–6) were in the macrophyte-dominated zone. We collected samples of basal sources and the most abundant consumers that were broadly representative of the respective biological communities. Suspended particulate organic matter (SPOM), phytoplankton and sediment organic matter (SOM) were considered as common basal sources in the two lake zones, and submerged plant was added as the fourth basal source in the macrophyte-dominate zone. The consumers in the two lake zones included zooplankton, zoobenthos, shrimp and fish. For zooplankton, rotifer (e.g., Brachionus calyciflorus) and cladocera (e.g., Bosmina longirostris and Moina rectirostris) were found to be the most common groups in the algae-dominated zone, whereas copepoda (e.g., Eucyclops serrulatus and Harpacticoida Sars) was the most common group in the macrophyte-dominated zone. Among zoobenthos, Corbicula fluminea was highly abundant in the algae-dominated zone while Bellamya purificata and Radix swinhoei were dominant species in the macrophyte-dominated zone. For shrimp, Macrobrachium nipponense was the dominant species in both lake zones. Furthermore, four feeding types of fishes were analyzed: herbivorous (only found in the macrophyte-dominated zone), filter-feeding, omnivorous and carnivorous fish. The carnivorous fish in the algae-dominated zone were divided into two groups: small and big. The small carnivorous fish species were mainly composed of Coilia ectenes taihuensis and Protosalanx chinensis and were dominant in the algae-dominate zone.
SPOM was collected by filtering surface lake water through pre-combusted (450 °C for 4 h) Whatman GF/F glass fiber filters with three replicates at each sampling site (Zeng et al. Citation2007). The Peterson Grab was used to collect sediment from the surface sediment for analysis of the stable isotope ratio of SOM. Phytoplankton was collected using a plankton net (64 µm mesh) and separated from other materials by centrifugation (Hamilton et al. Citation2005). Submerged plant material was collected along with the sediment, washed and then cold stored.
Zooplankton was collected using plankton nets at the surface water of each station for 10 min with three replicates. Samples were sorted in the laboratory using a stereomicroscope (Vizzini and Mazzola Citation2006). Zoobenthos were collected from the surface sediment using the Peterson Grab, and the muscle tissues harvested for large species, whereas the whole body was collected by removing the viscera and shells for small species. All zooplankton and zoobenthos samples were kept alive in polythene bottles for 24 h to enable them excrete the gut contents (Rawcliffe et al. Citation2010). Fish were obtained using a trawl net with a mesh size of 1 mm in the algae-dominated zone, and with traps in the macrophyte-dominated zone because the trawl net cannot be operated normally under dense submerged macrophyte. For large fish species (individual length > 10 cm), a small transverse sample was cut from the dorsal muscle tissue after the fish were dead (Pinnegar and Polunin Citation1999). For small fish species (individual length < 10 cm), the whole body of several individuals were pooled into one sample after the viscera were removed. Shrimp were collected along with fish, shucked, and the flesh posterior to the heads were excised for analysis.
To reduce disturbance of the inorganic carbon content to δ13C, we used the acidification process (i.e., adding 1 mol/L hydrochloric acid [HCl], dropwise) on SOM samples that were rich in carbonates prior to the analysis. Prior to stable isotope analyses, all samples were freeze-dried, powdered, sifted and weighed to the nearest 0.01 mg into tin capsules.
2.3. Stable isotope analysis
Carbon and nitrogen isotope ratios were determined at the Chinese Academy of Forestry Laboratory using isotope ratio mass spectrometry (Finnigan MAT Delta V advantage). The results are expressed in conventional delta (δ) notation as parts per thousand (‰) defined as follows:
where R represents 13C/12C or 15N/14N. The PeeDee Belemnite (PDB) was used as the standard for 13C, and atmospheric N2 (AIR) was used as the standard for 15N. The analysis precision was within 0.3‰ for both δ13C and δ15N.
2.4. Statistical analyses
First, δ13C and δ15N values of the basal sources and consumers were compared between or within the lake zones using an analysis of variance (ANOVA). Data that did not pass the test of variance homogeneity were analyzed using the Mann-Whitney or Kruskal-Wallis non-parametric tests. A statistical significance level of α = 0.05 was used. Then, bio-plots of δ13C vs δ15N were used to compare the spatial heterogeneity of the food web structure between different lake zones. All statistical analyses were conducted using the software of Origin 8.5 (OriginLab Corporation, Northampton, USA, 2010).
2.5. Food web construction
To construct the food web skeletal structures of the two lake zones, we classified trophic groups by grouping consumers based on similar diets. The common trophic groups in the two lake zones were SPOM, SOM, phytoplankton, zooplankton, shrimp, zoobenthos, filter-feeding fish, omnivorous fish and carnivorous fish. Some specific trophic groups existed in the skeleton food web structures of the two lake zones. For example, the macrophyte-dominated zone had the submerged macrophyte as its specific basal source trophic group and herbivorous fish (i.e., Ctenopharyngodon idella), while the algae-dominated zone had the small carnivorous fish (i.e., Coilia ectenes taihuensis and Protosalanx chinensis) as its unique consumer trophic group. The empirical diet compositions of each trophic group were collected from the gut-content analysis results in Lake Taihu published previously (Institute of Zoology – Chinese Academy of Sciences Citation1979a, Citation1979b, Citation2000; Qi Citation1998; Ni and Zhu Citation2005).
We estimate the trophic levels of species and trophic groups according to Post (Citation2002):
where
is the stable isotope ratio of each species or trophic group, and
is the baseline organisms (zooplankton in our study), 3.4 is the expected
fractionation per trophic level (Post Citation2002), and 2 is the trophic position of baseline organisms. The maximum trophic level value was considered as the food chain length of each lake zone.
The relative contribution of food sources to diets of the consumers was estimated by using the Stable Isotope Analysis in R (SIAR) package in the R language and environment for statistical computing (Parnell et al. 2008). The SIAR is a Bayesian isotopic mixing model that evaluates the distribution probability of a given consumer’s diet composition. The input data included the stable isotope ratio (δ13C and δ15N) of each basal source with its stand error, the stable isotope ratio of consumer and the trophic enrichment factors (TEF) value of each source, with the associated standard error. Trophic enrichment values for C were assumed to be 0.4 ± 1.3‰ for δ13C and 3.4 ± 1.0‰ for δ15N (Post Citation2002). Mean values of dietary proportions of each species were calculated correspondingly.
3. Results
3.1. Spatial heterogeneity of carbon and nitrogen stable isotope ratios of basal sources
The basal sources in the algae-dominated zone had a relatively narrow δ13C ratio range, while that of the basal sources in the macrophyte-dominated zone was wider ( and ). The Kruskal-Wallis test results revealed that basal sources of these two lake zones showed distinct δ13C ratios (algae- and macrophyte-dominated zones: H = 12.865 and 12.699, P = 0.002 and 0.005, respectively). Therefore, they could act as distinct trophic bases in the following analysis. The δ13C ratio of basal sources in the algae-dominated zone ranged from −28.13‰ (SPOM) to −24.06‰ (phytoplankton), and its variation was 4.07‰. In contrast, the δ13C ratio of basal sources in the macrophyte-dominated zone ranged from −24.89‰ (SPOM) to −15.18‰ (submerged macrophyte), and its variation range reached 9.71‰. There were more depleted δ13C ratios of basal sources in the algae-dominated zone than there were in the macrophyte-dominated zone. The mean δ13C ratio of basal sources in the algae-dominated zone was −25.69‰, which was −4.86‰ lower than that in the macrophyte-dominated zone was. The ANOVA of SPOM, phytoplankton and SOM showed significant differences in the δ13C ratios of these three basal sources between the two lake zones ().
Figure 2. Bi-plots of mean δ13C and δ15N values of basal sources and consumers in the (a) algae- and (b) macrophyte-dominated zones in Lake Taihu. Trophic level are given on the right y-axis, assuming a 3.4‰ increase in δ15N per level. Gray diamond: Basal food sources (Phy: Phytoplankton; SM: Submerged macrophyte); downtriangle: Zooplankton; circle: Zoobenthos (Cfl: Corbicula fluminea; Bae: Bellamya aeruginosa; Bpu: Bellamya purificata; Rws: Radix swinhoei); righttriangle: Shrimp; black square: Fish (1: Hypophthalmichthys molitrix; 2: Aristichthys nobilis; 3: Ctenopharyngodon idella; 4: Carassius auratus; 5: Cyprinus carpio; 6: Mylopharyngodon piceus; 7: Coilia ectenes taihuensis; 8: Protosalanx chineniss; 9: Erythroculter ilishaeformis; 10: Pelteobagrus fulvidraco; 11: Parasilurus asotus; 12: Channa argus Cantor; 13: Siniperca chuatsi).
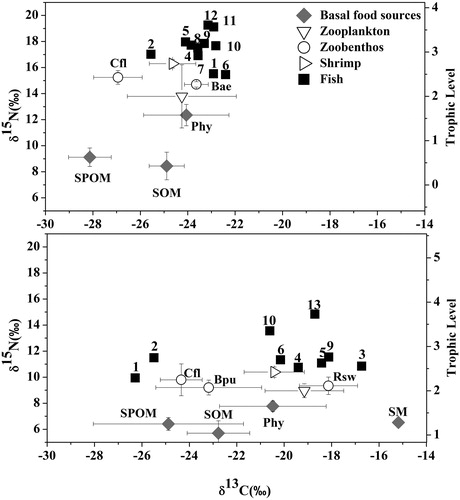
Table 2. Carbon and nitrogen stable isotope ratios (mean, with SD followed in parentheses) of the basal sources and consumers in the algae- and macrophyte-dominated zones in Lake Taihu.
Table 3. ANOVA results comparing differences of δ13C and δ15N in trophic groups between the algae- and macrophyte-dominated lake zones in Lake Taihu.
The δ15N ratios of basal sources in the two lake zones showed opposite distribution patterns compared to those of the δ13C ratios ( and ). The basal sources in the algae-dominated zone had δ15N ratios between 8.44‰ (SOM) and 12.35‰ (phytoplankton), and the changing magnitude reached 3.91‰. The basal sources in the macrophyte-dominated zone had relatively lower δ15N ratios than those in the other zone did. Their δ15N ratios ranged from 5.70‰ (SOM) to 7.77‰ (phytoplankton), and the changing magnitude was 2.07%. In addition, the basal sources in the algae-dominated zone had more enriched δ15N ratios than those in the macrophyte-dominated zone. The average δ15N ratio of basal sources in the algae-dominated zone was 3.37‰ higher than that in the macrophyte-dominated zone. The ANOVA showed that there were significant differences in the δ15N ratios in basal sources (SPOM, phytoplankton and SOM) between the two lake zones ().
3.2. Spatial heterogeneity of carbon and nitrogen stable isotope ratios of consumers
The δ13C ratios of consumers in the algae-dominated zone were narrower than those in the other zone ( and ). The δ13C range of consumers was from −26.95‰ (Corbicula fluminea) to −22.40‰ (Mylopharyngodon piceus) in the algae-dominated zone, but it was from −26.28‰ (Hypophthalmichthys molitrix) to −16.73‰ (Ctenopharyngodon idella) in the macrophyte-dominated zone. All consumers in the algae-dominated zone had significant lower mean δ13C values than those in the macrophyte-dominated zone did except for the filter-feeding fishes ( and ).
Figure 3. Comparisons of mean (±1 S.D.) carbon and nitrogen stable isotope ratios of consumers in the two lake zones (zoo. = zooplankton, zooben. = zoobenthos, Fil fish = filter-feeding fish, Omn fish = omnivorous fish, Carn fish = carnivorous fish).
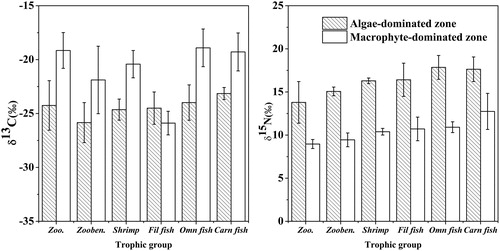
The mean δ15N values of consumers in the algae-dominated zone varied from 13.79‰ (zooplankton) to 19.25‰ (Channa argus Cantor), and from 8.96‰ (zooplankton) to 14.83‰ (Siniperca chuatsi) in the macrophyte-dominated zone. The average δ15N values of consumers were significantly higher in the algae-dominated zone than those in the macrophyte-dominated zone ( and ). The changing magnitude of the δ15N values in consumers was 5.46‰ and 5.87‰ in the algae- and macrophyte-dominated zones, respectively.
3.3. Spatial heterogeneity of food chain length and key trophic linkages
Although the consumers in the algae-dominant zone had higher average δ15N values, the results of trophic level calculations showed the macrophyte-dominated zone was longer food chain length than algae-dominated zone. Using the assumption of δ15N fractionation per trophic level of 3.4‰, and the zooplankton as the baseline trophic level, the maximum trophic level in the algae-dominated zone was Channa argus Cantor with 3.56, while the maximum trophic level in the marophyte-dominated zone was Siniperca chuatsi with 3.73.
The relative contributions of food sources to consumers’ diets in the two lake zones are presented in and . The zooplankton in the macrophyte-dominated zone appeared to depend more on SPOM than on phytoplankton than the algae-dominated zone. The zoobenthos in the algae-dominated zone mainly fed on planktons (mean distribution, 32.29% for phytoplankton and 26.92% for zooplankton), whereas the carbon source from sediment was the major component (mean distribution was 44.99%) for the zoobenthos in the macrophyte-dominated zone. Zooplankton was the largest food source for the shrimps (mean distribution was 32.62%) followed by phytoplankton, SPOM and SOM in the algae-dominated zone. In contrast, SOM became the largest food source for the shrimps (mean distribution, 26.42%) followed by phytoplankton, zooplankton and SPOM in the macrophyte-dominated zone. The filter-feeding fishes (Hypophthalmichthys molitrix and Aristichthys nobilis) in Lake Taihu were artificially stocked and fed other food sources before they were released into the lake, so their diet distributions calculated by SIAR model may reflect previous dietary contributions. Omnivorous fish had the most diversified food sources in both lake zones. Among the various choices, the submerged macrophyte was an important food source for omnivorous fishes in the macrophyte-dominated zone where its proportion possessed the first place in two species (Carassius auratus, Cyprinus carpio). The omnivorous fish in the algae-dominated zone showed no preference on food source choice. Small carnivorous fish (Coilia ectenes taihuensis and Protosalanx chinensis) was a special trophic group in the algae-dominated zone food web. Mixing model outcomes showed that zooplankton was the most dependent food source for small carnivorous fishes: its contribution exceeded 50% for Coilia ectenes taihuensis and Protosalanx chinensis. The big carnivorous fish in the two lake zones had relatively equal food source choices. The only exception was Erythroculter ilishaeformis in the macrophyte-dominated zone where its diet was dominated by zooplankton (mean distribution was 63.51%).
Figure 4. Mean relative contributions of food sources to consumer diets in (a) algae- and (b) macrophyte-dominated zones in Lake Taihu. SPOM = suspended particulate organic matter, PHY = phytoplankton, SOM = sediment organic matter, Small Zoo-prefer Carn Fish = small carnivorous fishes (individual length <10 cm) which prefer to eat zooplankton, Big Zoo-prefer Carn Fish = big carnivorous fishes (individual length >10 cm) which prefer to eat zooplankton, Zoob-prefer Carn Fish = carnivorous fishes which prefer to eat zoobenthos, Fish-prefer Carn Fish = carnivorous fishes which prefer to eat fishes.
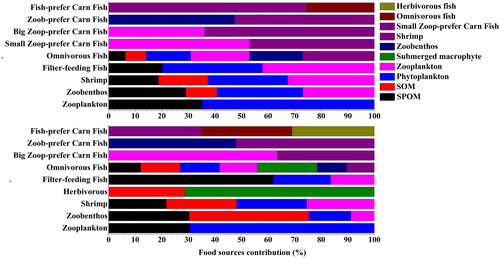
Figure 5. Food webs of (a) algae- and (b) macrophyte-dominated zones in Lake Taihu, based on stable isotope analysis. The line width represents proportional contribution of food source. SPOM = suspended particulate organic matter, PHY = phytoplankton, SOM = sediment organic matter, Zoo = zooplankton, Zoob = zoobenthos, Shr = shrimp, FFF = filter-feeding fish, HerF = herbivorous fish, OmnF = Omnivorous Fish, SZPCF = small carnivorous fishes (total length <10 cm) which prefer to eat zooplankton, BZPCF = big carnivorous fishes (total length >10 cm) which prefer to eat zooplanktion, ZPCF = carnivorous fishes which prefer to eat zoobenthos, FPCF = carnivorous fishes which prefer to eat fishes.
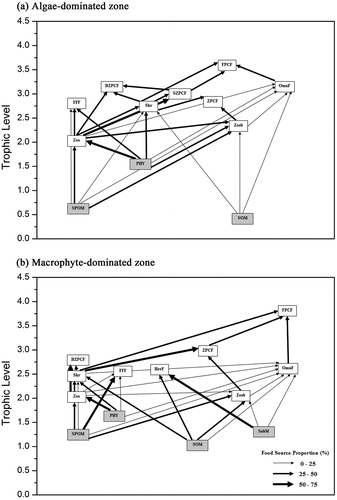
By analyzing the complex feeding relationships in the skeleton food webs, we identified the related key trophic linkages (the mean relative contribution of each food source to its consumer diet exceeded 50%) of the two food webs (). The key trophic linkages in the algae-dominated zone started from phytoplankton to zooplankton, and then to small zooplankton-preferred carnivorous fish (e.g., the Coilia ectenes taihuensis and Protosalanx chinensis). Moreover, three key trophic linkages existed in the food web of the macrophyte-dominated zone: (1) from SPOM to filter-feeding fish; (2) from phytoplankton to zooplankton, and then to big zooplankton-preferred fish (e.g., Erythroculter ilishaeformis); and (3) from submerged macrophyte to herbivorous fish (e.g., Ctenopharyngodon idella).
4. Discussion
Results based on stable isotope analyses showed large spatial heterogeneity in food web structure between the two stable states lake zones in Lake Taihu. The carbon and nitrogen stable isotopes of most trophic groups reflected significant differences in the two lake zones, and showed the trends of lower δ13C ratio and higher δ15N ratio in the algae-dominated than in the macrophyte-dominated zone. However, the food web in the macrophyte-dominated zone showed longer food chain length and more diver diverse key trophic linkages for transfer matter and energy. This similar research results can also be found in some shallow lake ecosystems (Rawcliffe et al. Citation2010; Ziegler et al. Citation2015; Iglesias et al. Citation2017), and river ecosystems (Roach Citation2013; Kaymak et al. Citation2018; Zheng et al. Citation2018).
The basal sources in the algae-dominated lake zone showed more narrow carbon range than that in the marcophyte-dominated lake zone. The submerged marcophyte expanded the carbon range of basal source in the macrophyte-dominated lake zone. Increased δ13C range of basal sources in food webs would be expected there are more abundant basal resources choice for consumers, and more diverse niche at the basal of food webs (Layman et al. Citation2007). The δ13C ratios of consumers also showed lighter and more concentrated trends in the algae-dominated than in the macrophyte-dominated zone. The narrow carbon range of the basal sources seems to limit the carbon source acquisition scope of consumers, and simplify the pathways for the transfer of energy and matter (Layman et al. Citation2012). These may have relationships with the shorter food chain length in the algae-dominated lake zone.
Different anthropogenic nutrient inputs around the sub-basins were likely driving the significant spatial δ13C and δ15N baseline differences in food webs of these two lake zones. Lake ecosystems which received more allochthonous loading showed lower δ13C and higher δ15N than those that had lower terrestrial contributions (Liu et al. Citation2012). The northwest area of Lake Taihu had higher allochthonous loading than the southeast area (Townsend-Small et al. Citation2007). In addition, the macrophyte-dominated zone is located in the outflow region of the Lake Taihu, while the algae-dominated zone is located in the inflow region where more terrestrial organic matter is received (Ngochera and Bootsma Citation2011). Excessive nutrient concentrations leaded to cyanobacteria become the important components of basal sources in algae-dominated zone, which has less negative δ13C ratios than other algae. Previous studies showed that the northwest area of Lake Taihu had more sewage-derived N than the southeast area (Mao et al. Citation2012, Citation2014). This may result in basal sources incorporating δ15N enriched NH4+, NO3− or both that originated from waste water (Carlisle et al. Citation2012). Therefore, the high δ15N ratios of basal sources and consumers in the algae-dominated zone may be affected by the inputs of human-related pollutants around this zone. Similar effects can also be found in stream ecosystems (Coat et al. Citation2009).
Although the δ15N ratios of consumers were significantly higher, the algae-dominated zone did not exhibit a longer food chain than that of the macrophyte-dominated zone. This has relationship with the higher baseline δ15N of the food web in the algae-dominated zone, and the δ15N ratios range among consumers in the macrophyte-dominated zone was larger than that in the algae-dominated zone. A larger range in δ15N ratios generally mean more trophic levels and a greater degree of trophic diversity (Layman et al. Citation2012). The δ15N ratios of primary consumers are used as indicators of the catchment anthropogenic impact level when compared with reference sites, and could facilitate weighing of the nitrogen stable isotope baseline of the food web (Turschak et al. Citation2014). Our findings are in agreement with those of previous studies where negative relationships between anthropogenic pollution inputs and food chain length in aquatic ecosystems were reported (Winemiller et al. Citation2011; Sensula Citation2015).
The spatial distinction of water depth is also a non-negligible factor that influenced the food web structure in these two contrasting and stable lake zones. As showed in , the mean water depth was 2.46 ± 0.39 m in the algae-dominated zone, and 1.68 ± 0.38 m in the macrophyte-dominated zone. Larger water depth will result in lower light intensity absorbed and utilized by submerged plants in water body, and make against the germination, growth and distribution of submerged plants (Le Fur et al. Citation2018; Heidbuchel and Hussner Citation2019). Furthermore, water depth also affects the underwater temperature, nutrients and dissolved oxygen (Jager et al. Citation2008). The difference of physical conditions within Lake Taihu decided the key component of basal source in the algae-dominated zone is algae, not submerged macrophyte. Previous studies have pointed out that the macrophyte-dominated zone also have received large amount of nutrient inputs (Liang et al. Citation2017). For instance, enclosure culture in East Taihu Bay (the region Site 5 located) reached its peak from 1998 to 2008 (Wang et al. Citation2019). Although suffered a mass of anthropogenic organic matter inputs, the water quality of East Taihu Bay was better than that of algae-dominated zone (Kong et al. Citation2018). It seems that the large area of submerged macrophytes and floating-leaf macrophytes play important role in maintain water quality and favorable food web structure.
The stable isotope results indirectly demonstrated that the exchange of organisms between these two lake zones was infrequent. There are few data available on the movement of organisms in the entire Lake Taihu, and most have focused on the movement of algae bloom with special wind-field conditions (Chen et al. Citation2012). Stable isotope ratios reflect diets integrated over time and space, and could be a useful tool to trace the movement of organisms (Solomon et al. Citation2015). The carbon and nitrogen stable isotopes of most trophic groups reflected significant differences in the two lake zones, and showed lower δ13C baseline and higher δ15N baseline in the algae-dominated than in the macrophyte-dominated zone. These results imply that the species in both lake zones might live in relatively stable habits. The only exception was the filter-feeding fishes (Hypophthalmichthys molitrix and Aristichthys nobilis). These filter-feeding fishes were artificially stocked into the whole lake as a biomanipulation strategy to control cyanobacterial blooms (Li et al. Citation2017). So their δ13C ratios may be affected by uncertain food sources before they were stocked. Further studies combining stable isotope analysis and electronic tagging may provide more accurate evidence from studying the migratory behaviors of organisms in the whole lake (Carlisle et al. Citation2012).
The food webs of the two lake zones depended on various food sources with a series of trophic linkages. In considering the spatial heterogeneity, our study provided a more targeted and quantitative food web for the different lake zones of Lake Taihu than previous studies (Mao et al. Citation2014; Li et al. Citation2018). In the algae-dominated zone, the small zooplankton-preferring carnivorous fishes appeared to play an important trophic role in the food web. Their proportion in the total catches of Lake Taihu have especially increased remarkably since 1980s (Xu et al. Citation2015). Considering their abundance and diet, small zooplankton-preferring carnivorous fishes exhibited strong predation pressure on zooplankton, and then had a trophic cascade effect on phytoplankton. The food web in the macrophyte-dominated zone had diverse key trophic links, and relatively dispersed predation pressures. It might be related to abundant food sources contributing to consumers, and consumers then have more choices to balance their diets. Predators need to adjust the diet choices to accommodate the variations of species composition and dominance in different habitats (Baudrot et al. Citation2016). Because of the spatial heterogeneity of ecological conditions and pollution loads, the food webs of the two studied zones showed distinct characteristics and processes. Spatial complexity of trophic structure seems to be a characteristic of complex ecosystems with heterogeneous environments in aquatic ecosystems (Nelson et al. Citation2015; Hetherington et al. Citation2017). Lake managers may consider this spatial heterogeneity of the food web in developing lake protection strategies.
Our results should be interpreted with caution because of the possible limitations of the sampling methods (for instance, we did not catch the small size fish species in the marcrophyte-dominated zone due to the big mesh size of traps) or the applied methodology (such as the assumption of the fractionation rate of δ15N ratio). Nevertheless, they provide an empirical evidence for the hypotheses that the food web with higher resources availability and lower environmental stress, has longer food chain length and more complex key trophic linkages for the transfer of matter and energy. Previous studies have reported that these two stable states can be transfer between each other by the oscillations of ecosystem process or external fluctuations (Ibelings et al. Citation2007; Terborgh et al. Citation2018). The macrophyte-dominant zone also receives external nutrients due to agricultural practices in this zone (Cai et al. Citation2012), and this stable state is susceptible and could be easily transformed into the algae-dominated stable state if the water level rises suddenly (Zhao et al. Citation2012), the water quality decline sharply (Janssen et al. Citation2017) or some other interruptions. Ecological engineering strategies, such as constructing enclosures or lowering the water level, may facilitate the partial restoration of the algae-dominated stable state to the macrophyte-dominated stable state, or prevent the transformation of the latter to the former (Zhang et al. Citation2016).
5. Conclusions
The food web of the Lake Taihu had distinct spatial heterogeneity in two contrasting lake zones. Resources availability and environmental stress in the systems may have contributed to this distinction. This spatially trophic heterogeneity needs to be considered by lake mangers in developing strategies for the restoration of this eutrophic shallow lake ecosystem.
Disclosure statement
No potential conflict of interest was reported by the authors.
Additional information
Funding
Notes on contributors
Delin Xu
Delin Xu is an associate research fellow of Nanjing Institute of Environmental Science, MEE, who works in food webs of freshwater ecosystem.
Yan Wang
Yan Wang is an assistant research fellow of Nanjing Institute of Environmental Science, MEE, who works in ecosystem health assessment.
Dong Liu
Dong Liu, Dan Wu are associate research fellows of Nanjing Institute of Environmental Science, MEE, who work in wetland management, particularly in shallow lakes.
Dan Wu
Dong Liu, Dan Wu are associate research fellows of Nanjing Institute of Environmental Science, MEE, who work in wetland management, particularly in shallow lakes.
Changxin Zou
Changxin Zou is an research fellow at Nanjing Institute of Environmental Science, MEE, where he has worked for many years as an ecologist, particularly in ecological space protection.
Yushun Chen
Yushun Chen is a professor of Institute of Hydrobiology, Chinese Academy of Sciences, where he conducts research on freshwater ecology and ecosystem health.
Ying Cai
Ying Cai got her doctor degree from School of Life Sciences, Nanjing University, and continued to do freshwater food web researches at Jiangsu Provincial Academy of Environmental Science.
Xin Leng
Xin Leng is an associate professor of School of Life Sciences, Nanjing University, and her research field is wetland management.
Shuqing An
Shuqing An is a professor of School of Life Sciences, Nanjing University, where he worked for many years as a teacher and ecologist, particularly in freshwater ecology.
References
- Anderson C, Cabana G. 2009. Anthropogenic alterations of lotic food web structure: evidence from the use of nitrogen isotopes. Oikos. 118(12):1929–1939.
- Baudrot V, Perasso A, Fritsch C, Giraudoux P, Raoul F. 2016. The adaptation of generalist predators' diet in a multi-prey context: insights from new functional responses. Ecology. 97(7):1832–1841.
- Cai CF, Gu XH, Huang HZ, Dai XY, Ye YT, Shi CJ. 2012. Water quality, nutrient budget, and pollutant loads in Chinese mitten crab (Eriocheir sinensis) farms around East Taihu Lake. Chin J Ocean Limnol. 30(1):29–36.
- Carlisle AB, Kim SL, Semmens BX, Madigan DJ, Jorgensen SJ, Perle CR, Anderson SD, Chapple TK, Kanive PE, Block BA. 2012. Using stable isotope analysis to understand the migration and trophic ecology of northeastern Pacific white sharks (Carcharodon carcharias). Plos One. 7(2):e30492.
- Chen LM, Wang CL, Li TL. 2012. Study on movement of cyanobacteria bloom with special wind-field condition in Taihu Lake. Admin Tech Environ Monit. 24(3):29–34.
- Coat S, Monti D, Bouchon C, Lepoint G. 2009. Trophic relationships in a tropical stream food web assessed by stable isotope analysis. Freshw Biol. 54(5):1028–1041.
- Cornelissen IJM, Vijverberg J, van den Beld AM, Helmsing NR, Verreth JAJ, Nagelkerke LAJ. 2018. Heterogeneity in food-web interactions of fish in the Mwanza Gulf, Lake Victoria: a quantitative stable isotope study. Hydrobiologia. 805(1):113–130.
- France RL. 1995. Differentiation between littoral and pelagic food webs in lakes using stable carbon isotopes. Limnol Oceanogr. 40(7):1310–1313.
- Guo L. 2007. Ecology. Doing battle with the green monster of Taihu Lake. Science. 317(5842):1166
- Hamilton SK, Sippel SJ, Bunn SE. 2005. Separation of algae from detritus for stable isotope or ecological stoichiometry studies using density fractionation in colloidal silica. Limnol Oceanogr Methods. 3(3):149–157.
- Heidbuchel P, Hussner A. 2019. Fragment type and water depth determine the regeneration and colonization success of submerged aquatic macrophytes. Aquat Sci. 81(1) https://doi.org/10.1007/s00027-018-0603-2.
- Hetherington ED, Olson RJ, Drazen JC, Lennert-Cody CE, Ballance LT, Kaufmann RS, Popp BN. 2017. Spatial food-web structure in the eastern tropical Pacific Ocean based on compound- specific nitrogen isotope analysis of amino acids. Limnol Oceanogr. 62(2):541–560.
- Hobbs WO, Theissen KM, Hagen SM, Bruchu CW, Czeck BC, Hobbs JMR, Zimmer KD. 2014. Persistence of clear-water, shallow-lake ecosystems: the role of protected areas and stable aquatic food webs. J Paleolimnol. 51(3):405–420.
- Hu LM, Hu WP, Deng JC, Li QQ, Gao F, Zhu JG, Han T. 2010. Nutrient removal in wetlands with different macrophyte structures in eastern Lake Taihu, China. Ecol Eng. 36(12):1725–1732.
- Ibelings BW, Portielje R, Lammens E, Noordhuis R, van den Berg MS, Joosse W, Meijer ML. 2007. Resilience of alternative stable states during the recovery of shallow lakes from eutrophication: Lake Veluwe as a case study. Ecosystems. 10(1):4–16.
- Iglesias C, Meerhoff M, Johansson LS, Gonzalez-Bergonzoni I, Mazzeo N, Pablo Pacheco J, Teixeira-de Mello F, Goyenola G, Lauridsen TL, Sondergaard M, et al. 2017. Stable isotope analysis confirms substantial differences between subtropical and temperate shallow lake food webs. Hydrobiologia. 784(1):111–123.
- Institute of Zoology – Chinese Academy of Sciences 1979a. Fauna of China (Arthropoda, Crustacea, Freshwater cladocera). Beijing: Science Press.
- Institute of Zoology – Chinese Academy of Sciences 1979b. Fauna of China (Arthropoda, Crustacea, Freshwater copepods). Beijing: Science Press.
- Institute of Zoology – Chinese Academy of Sciences 2000. Fauna of China (Arthropoda, Crustacea, mysidacea). Beijing: Science Press.
- Jager CG, Diehl S, Schmidt GM. 2008. Influence of water-column depth and mixing on phytoplankton biomass, community composition, and nutrients. Limnol Oceanogr. 53(6):2361–2373.
- Janssen ABG, de Jager VCL, Janse JH, Kong XZ, Liu S, Ye QH, Mooij WM. 2017. Spatial identification of critical nutrient loads of large shallow lakes: Implications for Lake Taihu (China). Water Res. 119:276–287.
- Janssen ABG, Teurlincx S, An SQ, Janse JH, Paerl HW, Mooij WM. 2014. Alternative stable states in large shallow lakes? J Great Lakes Res. 40(4):813–826.
- Kaymak N, Winemiller KO, Akin S, Altuner Z, Polat F, Dal T. 2018. Spatial and temporal variation in food web structure of an impounded river in Anatolia. Mar Freshwater Res. 69(9):1453–1471.
- Kong M, Chao JY, Zhuang W, Wang PF, Wang C, Hou J, Wu ZS, Wang LM, Gao GG, Wang Y. 2018. Spatial and temporal distribution of particulate phosphorus and their correlation with environmental factors in a shallow eutrophic Chinese lake (Lake Taihu). IJERPH. 15(11) https://doi.org/10.3390/ijerph15112355.
- Kong XZ, He W, Qin N, Liu WX, Yang B, Yang C, Xu FL, Mooij WM, Koelmans AA. 2017. Integrated ecological and chemical food web accumulation modeling explains PAH temporal trends during regime shifts in a shallow lake. Water Res. 119:73–82.
- Kopec AD, Kidd KA, Fisher NS, Bowen M, Francis C, Payne K, Bodaly RA. 2019. Spatial and temporal trends of mercury in the aquatic food web of the lower Penobscot River, Maine, USA, affected by a chlor-alkali plant. Sci Total Environ. 649:770–791.
- Kuiper JJ, Verhofstad M, Louwers ELM, Bakker ES, Brederveld RJ, van Gerven LPA, Janssen ABG, de Klein JJM, Mooij WM. 2017. Mowing submerged macrophytes in shallow lakes with alternative stable states: battling the good guys? Environ Manage. 59(4):619–634.
- Layman CA, Araujo MS, Boucek R, Hammerschlag-Peyer CM, Harrison E, Jud ZR, Matich P, Rosenblatt AE, Vaudo JJ, Yeager LA, et al. 2012. Applying stable isotopes to examine food-web structure: an overview of analytical tools. Biol Rev Camb Philos Soc. 87(3):545–562.
- Layman CA, Arrington DA, Montana CG, Post DM. 2007. Can stable isotope ratios provide for community-wide measures of trophic structure? Ecology. 88(1):42–48.
- Le Fur I, De Wit R, Plus M, Oheix J, Simier M, Ouisse V. 2018. Submerged benthic macrophytes in Mediterranean lagoons: distribution patterns in relation to water chemistry and depth. Hydrobiologia. 808(1):175–200.
- Li Y, Xie P, Zhang J, Tao M, Deng X. 2017. Effects of filter-feeding planktivorous fish and cyanobacteria on structuring the zooplankton community in the eastern plain lakes of China. Ecol Eng. 99:238–245.
- Li YK, Song B, Chen Y, Chen LQ, Yu N, Olson D. 2010. Changes in the trophic interactions and the community structure of Lake Taihu (China) ecosystem from the 1960s to 1990s. Aquat Ecol. 44(2):337–348.
- Li YK, Zhang YY, Xu J, Zhang S. 2018. Spatial variations in food web structures with alternative stable states: evidence from stable isotope analysis in a large eutrophic lake. J Ocean Limnol. 36(2):385–394.
- Liang QC, Zhang YC, Ma RH, Loiselle S, Li J, Hu MQ. 2017. A MODIS-based novel method to distinguish surface cyanobacterial scums and aquatic macrophytes in Lake Taihu. Remote Sens. 9(2)
- Liu DY, Chen G, Li YC, Gu BH. 2012. Global pattern of carbon stable isotopes of suspended particulate organic matter in lakes. Limnology. 13(2):253–260.
- Lopez Moreira Mazacotte GA, Hinegk L, Salvadore A, Zolezzi G, Holker F, Monte Domecq Serrati RA, Bocci M, Carrer S, D, Nat L, Escriba J, Escriba C, et al. 2018. Eutrophication, research and management history of the shallow Ypacarai Lake (Paraguay). Sustainability. 10(7)
- Mao ZG, Gu XH, Zeng QF, Chen HH. 2016. Carbon sources and trophic structure in a macrophyte-dominated polyculture pond assessed by stable-isotope analysis. Freshw Biol. 61(11):1862–1873.
- Mao ZG, Gu XH, Zeng QF, Pan G. 2014. Seasonal and spatial variations of the food web structure in a shallow eutrophic lake assessed by stable isotope analysis. Fish Sci. 80(5):1045–1056.
- Mao ZG, Gu XH, Zeng QF, Zhou LH, Sun MB. 2012. Food web structure of a shallow eutrophic lake (Lake Taihu, China) assessed by stable isotope analysis. Hydrobiologia. 683(1):173–183.
- Nelson JA, Deegan L, Garritt R. 2015. Drivers of spatial and temporal variability in estuarine food webs. Mar Ecol Prog Ser. 533:67–77.
- Ngochera MJ, Bootsma HA. 2011. Temporal trends of phytoplankton and zooplankton stable isotope composition in tropical Lake Malawi. J Great Lakes Res. 37:45–53.
- Ni Y, Zhu CD. 2005. Ichthyography of Taihu Lake. Shanghai: Shanghai Technology Publisher.
- Parnell AC, Inger R, Bearhop S, Jackson AL. 2008. SIAR: stable isotope analysis in R. http://cran.r-project.org/web/packages/siar/index.html.
- Pinnegar JK, Polunin NVC. 1999. Differential fractionation of delta C-13 and delta N-15 among fish tissues: implications for the study of trophic interactions. Funct Ecol. 13(2):225–231.
- Post DM. 2002. Using stable isotopes to estimate trophic position: models, methods, and assumptions. Ecology. 83(3):703–718.
- Qi ZY. 1998. Economic mollusca of China. Beijing: Chinese Agriculture Press.
- Qin BQ, Hu WP, Chen WM. 2004. Process and mechanism of environmental changes of the Taihu Lake. Beijing: Science Press.
- Qin BQ, Xu PZ, Wu QL, Luo LC, Zhang YL. 2007. Environmental issues of Lake Taihu, China. Hydrobiologia. 581(1):3–14.
- Qu CS, Chen W, Bi J, Huang L, Li FY. 2011. Ecological risk assessment of pesticide residues in Taihu Lake wetland, China. Ecol Model. 222(2):287–292.
- Rawcliffe R, Sayer CD, Woodward G, Grey J, Davidson TA, Jones JI. 2010. Back to the future: using palaeolimnology to infer long-term changes in shallow lake food webs. Freshw Biol. 55(3):600–613.
- Roach KA. 2013. Environmental factors affecting incorporation of terrestrial material into large river food webs. Freshw Sci. 32(1):283–298.
- Scharroba A, Kramer S, Kandeler E, Ruess L. 2016. Spatial and temporal variation of resource allocation in an arable soil drives community structure and biomass of nematodes and their role in the micro-food web. Pedobiologia. 59(3):111–120.
- Scheffer M, Jeppesen E. 2007. Regime shifts in shallow lakes. Ecosystems. 10(1):1–3.
- Sensula BM. 2015. Spatial and short-temporal variability of delta C-13 and delta N-15 and water-use efficiency in pine needles of the three forests along the most industrialized part of Poland. Water Air Soil Pollut. 226(11)
- Solomon CT, Carpenter SR, Clayton MK, Cole JJ, Coloso JJ, Pace ML, Vander Zanden MJ, Weidel BC. 2011. Terrestrial, benthic, and pelagic resource use in lakes: results from a three-isotope bayesian mixing model. Ecology. 92(5):1115–1125.
- Solomon CT, Jones SE, Weidel BC, Buffam I, Fork ML, Karlsson J, Larsen S, Lennon JT, Read JS, Sadro S, Saros JE. 2015. Ecosystem consequences of changing inputs of terrestrial dissolved organic matter to lakes: current knowledge and future challenges. Ecosystems. 18(3):376–389.
- Syvaranta J, Hogmander P, Keskinen T, Karjalainen J, Jones RI. 2011. Altered energy flow pathways in a lake ecosystem following manipulation of fish community structure. Aquat Sci. 73(1):79–89.
- Terborgh JW, Davenport LC, Belcon AU, Katul G, Swenson JJ, Fritz SC, Baker PA. 2018. Twenty-three-year timeline of ecological stable states and regime shifts in upper Amazon oxbow lakes. Hydrobiologia. 807(1):99–111.
- Thompson RM, Brose U, Dunne JA, Hall RO, Hladyz S, Kitching RL, Martinez ND, Rantala H, Romanuk TN, Stouffer DB, Tylianakis JM. 2012. Food webs: reconciling the structure and function of biodiversity. Trends Ecol Evol. 27(12):689–697.
- Townsend-Small A, McCarthy MJ, Brandes JA, Yang LY, Zhang L, Gardner WS. 2007. Stable isotopic composition of nitrate in Lake Taihu, China, and major inflow rivers. Hydrobiologia. 581:135–140.
- Turschak BA, Bunnell D, Czesny S, Hook TO, Janssen J, Warner D, Bootsma HA. 2014. Nearshore energy subsidies support Lake Michigan fishes and invertebrates following major changes in food web structure. Ecology. 95(5):1243–1252.
- van Nes EH, Rip WJ, Scheffer M. 2007. A theory for cyclic shifts between alternative states in shallow lakes. Ecosystems. 10(1):17–27.
- Vander Zanden MJ, Shuter BJ, Lester N, Rasmussen JB. 1999. Patterns of food chain length in lakes: a stable isotope study. Am Nat. 154(4):406–416.
- Vizzini S, Mazzola A. 2006. Sources and transfer of organic matter in food webs of a Mediterranean coastal environment: Evidence for spatial variability. Estuar Coast Shelf Sci. 66(3–4):459–467.
- Wang SP, Brose U. 2018. Biodiversity and ecosystem functioning in food webs: the vertical diversity hypothesis. Ecol Lett. 21(1):9–20.
- Wang SS, Gao YN, Li Q, Gao JF, Zhai SH, Zhou Y, Cheng YH. 2019. Long-term and inter-monthly dynamics of aquatic vegetation and its relation with environmental factors in Taihu Lake, China. Sci Total Environ. 651:367–380.
- Wang YY, Yu XB, Zhang L, Lei GC. 2013. Seasonal variability in baseline δ15N and usage as a nutrient indicator in Lake Poyang, China. J Freshw Ecol. 28(3):365–373.
- Winemiller KO, Hoeinghaus DJ, Pease AA, Esselman PC, Honeycutt RL, Gbanaador D, Carrera E, Payne J. 2011. Stable isotope analysis reveals food web structure and watershed impacts along the fluvial gradient of a Mesoamerican coastal river. River Res Applic. 27(6):791–803.
- Woodward G. 2009. Biodiversity, ecosystem functioning and food webs in fresh waters: assembling the jigsaw puzzle. Freshw Biol. 54(10):2171–2187.
- Xu DL, Cai Y, Wu XQ, Jiang H, Leng X, An SQ. 2015. Regime shifts and resilience of the Lake Taihu social-ecological system under long-term external disturbance (1960s–2000s). Clean Soil Air Water. 43(4):561–568.
- Xu X, Yang G, Tan Y, Zhuang Q, Li H, Wan R, Su W, Zhang J. 2016. Ecological risk assessment of ecosystem services in the Taihu Lake Basin of China from 1985 to 2020. Sci Total Environ. 554:7–16.
- Zeng QF, Kong FX, Zhang EL, Wu XD, Tan X. 2007. Stable isotope analyses of size-based microplankton trophic structure in Taihu Lake during a cyanobacterial bloom. J Freshw Ecol. 22(4):553–559.
- Zhang YL, Liu XH, Qin BQ, Shi K, Deng JM, Zhou YQ. 2016. Aquatic vegetation in response to increased eutrophication and degraded light climate in Eastern Lake Taihu: implications for lake ecological restoration. Sci Rep. 6:2426.
- Zhao DH, Jiang H, Cai Y, An SQ. 2012. Artificial regulation of water level and its effect on aquatic macrophyte distribution in Taihu Lake. PLoS One. 7(9):e44836. doi:44810.41371/journal.pone.0044836.
- Zheng YY, Niu JG, Zhou Q, Xie CX, Ke ZX, Li DP, Gao YW. 2018. Effects of resource availability and hydrological regime on autochthonous and allochthonous carbon in the food web of a large cross-border river (China). Sci Total Environ. 612:501–512.
- Ziegler JP, Solomon CT, Finney BP, Gregory-Eaves I. 2015. Macrophyte biomass predicts food chain length in shallow lakes. Ecosphere. 6(1)