Abstract
The growth, survival, and non-structural carbohydrate metabolism in Salix triandroides cuttings, cultivated under no flooding, incomplete submergence, or complete submergence conditions (0, 25, and 50 cm of water above the soil surface, respectively), were measured after 50, 80, 110, and 140 days. Both submergence conditions decreased the biomass of the leaf, stem, and root, plant height, root length, and root number. As the duration of the treatments increased, these growth parameters gradually increased for the control and incompletely submerged conditions, but remained relatively constant for the completely submerged condition. The survival ratio was 100% across the experimental period for plants under the control and incompletely submerged conditions; however for plants under the completely submerged condition, it was 100% after 50 and 80 days but decreased to 91.7 and 33.3% after 110 and 140 days, respectively. Compared with the plants under control and incomplete submergence conditions, the completely submerged plants had significantly lower concentrations of soluble sugars and starch. As the concentration of soluble sugars in the completely submerged plants fell to 19.2%, and that of starch to 23.5% of that in the control plants, their survival ratio decreased to 33.3%. The results indicated that the survival of the S. triandroides cuttings grown in complete submergence conditions was associated with non-structural carbohydrate metabolism.
Introduction
Flooding is a natural environmental factor that adversely affects plant growth and survival (Pan et al. Citation2012; Kurzatkowski et al. Citation2015; Ding et al. Citation2017). Its detrimental effects are mainly attributable to the consequent oxygen and light limitations, and the scale of its impact is closely related to the intensity of the flooding (Ding et al. Citation2017; Loreti et al. Citation2016). During incomplete submergence, plants are able to maintain aerobic respiration and growth, due to their aerial organs, that absorb the light required for plant photosynthesis (Striker Citation2012; Yu et al. Citation2012; Ye et al. Citation2018), and aerenchyma that are formed to transmit oxygen for plant respiration (Ding et al. Citation2017; Loreti et al. Citation2016). However, during complete submergence, less oxygen is acquired due to slow gas diffusion in water (Mohanty et al. Citation2000; Voesenek et al. Citation2006). When exposed to anaerobic conditions, plants shift their metabolism from aerobic respiration to alcoholic fermentation (Peña-Fronteras et al. Citation2009). Moreover, photosynthesis is inhibited due to the low light availability (Loreti et al. Citation2016; van Veen et al. Citation2016) and low stomatal apertures in water (Mishra et al. Citation2008; Rodríguez-Gamir et al. Citation2011). Under such conditions, plants may enter a state of inactivity or dormancy, such as defoliation and atrophy, to reduce energy demands and preserve available energy (Li et al. Citation2007; Voesenek and Bailey-Serres Citation2015). Once photosynthesis is interrupted, the metabolism of stored non-structural carbohydrates in plants becomes crucial for tissue respiration and plant survival (Luo et al. Citation2011; Striker Citation2012; Yu et al. Citation2012).
As non-structural carbohydrates, soluble sugars such as glucose, fructoses, and maltose, are the main substrates for plant respiration, and they are widespread in higher plants (Peña-Fronteras et al. Citation2009; Rodríguez-Calcerrada et al. Citation2011; Striker Citation2012; Béjaoui et al. Citation2016). At low concentrations of soluble sugars, starch, another non-structural carbohydrate, is degraded into soluble sugars (Loreti et al. Citation2003; Lee et al. Citation2008; Gimeno et al. Citation2012; Du et al. Citation2014). As flooding continues, the stored non-structural carbohydrates in plants are gradually degraded (Striker Citation2012; Yu et al. Citation2012; Béjaoui et al. Citation2016), and as the soluble sugar concentrations drop below the threshold for tissue respiration and the stored starches are depleted, plants begin their demise (Das et al. Citation2005; Li et al. Citation2007; Luo et al. Citation2011). Therefore, plant survival in completely submerged conditions, may be associated with the metabolism of non-structural carbohydrates (Jackson et al. Citation2009; Perata et al. Citation2011; Striker Citation2012).
Salix triandroides, an indigenous small tree growing in the Dongting Lake wetlands in China, plays important roles in wave reduction, levee protection, and biodiversity conservation (Li et al. Citation2014). This plant germinates in February and it is submerged from May to October. After the retreat of the flooding waters, S. triandroides plants that grow on higher grounds recover, but those that occupy low-laying areas fail to germinate. A few studies have clarified the metabolic processes of non-structural carbohydrates in plants under flooding stress (Striker Citation2012), and to date, the role of non-structural carbohydrates in plant survival during complete plant submergence remains unclear. In the present paper, a simulated experiment was conducted using three water levels (0 cm, control; 25 cm above soil surface, incomplete submergence; and 50 cm above soil surface, complete submergence) to test the following hypothesis: (1) Growth and survival of plants subjected to complete submergence are significantly lower, compared with plants exposed to no or incomplete submergence; (2) Plant survival in completely submerged conditions is positively associated with non-structural carbohydrate concentrations.
Methods
Plant materials
Cuttings of S. triandroides were collected from the Dongting Lake wetlands. This lake is the second largest freshwater lake in China and covers an area of 2,625 km2 (28°38′–29°45′N, 111°40′–113°10′E). The lake catchment is characterized by a subtropical monsoon climate, with an average annual temperature of 17.0 °C and 268 frost-free days. The mean annual precipitation ranges from 1,200 to 1,415 mm, and the rainy season extends from April to August. The annual average humidity is 80%, and the annual average evaporation is 1,270 mm. The annual fluctuation in the water level is approximately 12.0–14.0 m, with the maxima in August and minima in January–February. The annual mean wind speed is 2.0–3.0 m s−1, and the elevation is 28.0–35.0 m above sea level (Xie et al. Citation2015).
In February 2017, cuttings (20.0 cm long) of one-year-old branches were obtained from two parent trees (29°16′10.1″N, 112°47′29.2″E) in the Dongting Lake wetlands and transported to the meteorological observation station at Hunan Agricultural University (28°11′6.9″N, 113°04′5.8″E), where they were planted in an outdoor nursery for bud germination. On April 15, 2017, the cuttings that had aboveground parts that were 13.0 cm long, were transplanted into PVC tubes (11.0 cm in diameter, 50.0 cm in height), with plastic mesh on the bottom to allow for the draining of water into the tube, and filled with 50.0 cm of soil collected from a paddy field (21.2 g kg−1 organic matter, 141.1 mg kg−1 exchangeable N, and 11.4 mg kg−1 exchangeable P). Tubes were then placed in outdoor cement ponds (150.0 cm in length, 100.0 cm in width, and 100.0 cm in height) for growth. The water level in the cement ponds relative to the soil surface was maintained at 0 cm using tap water (51.1 μg L−1 NH4–N, 176.3 μg L−1 NO3–N, 52.7 μg L−1 PO43+–P, pH = 7.2).
Experimental design
On May 15, 2017, cuttings that had reached 28.5–42.1 cm (35.3 ± 5.6) in height and had 3–6 (4.3 ± 1.2) branches and 48–83 (60.8 ± 13.0) leaves were selected for the submergence experiment. A one-way factorial design was used for this experiment, which consisted of three water levels: 0, 25, and 50 cm above the soil surface. Three cement ponds were prepared per treatment. A total of 126 cuttings were used for the experiment, with 42 cuttings in each cement pond. The water level in each pond was maintained using tap water.
Plant harvest
To imitate the flooding dynamics in the Dongting Lake wetlands, plants were harvested after 50, 80, 110, and 140 days. For each treatment group, there were 10 cuttings for each harvest after 50 and 80 days, and 11 cuttings for each harvest after 110 and 140 days. The intact cuttings, including root tissues, were carefully removed from the soil, and the survival ratio and plant height were recorded. The cuttings were scored as dead when the transverse section of the stem base was completely dark brown, as this indicated lifelessness in the plant (Noodéb and Penney Citation2011; Anderegg et al. Citation2012). The roots were washed with tap water, and the length of the longest root and the length and number of the primary adventitious roots (PAR) were measured. The number of secondary adventitious roots (SAR) was recorded in 10 randomly chosen PAR in each cutting. The plants were then divided into leaves, stems, and roots and dried at 80 °C for 48 h to measure dry biomass.
Measurement of non-structural carbohydrate concentration
The concentration of non-structural carbohydrates was measured following the procedure described by Yoshida et al. (Citation1976). The aboveground and underground parts of the plants were dried and ground to a fine powder and extracted three times using 80% ethanol (v/v). The extract was then used for the soluble sugars analysis after the addition of the anthrone reagent, followed by measurement of its absorbance at 630 nm using a spectrophotometer (723 N, Jinghua Instruments, China). The residue remaining after the soluble sugar extraction was dried and extracted using 30% perchloric acid, and the extract was analyzed for starch (as glucose equivalent) using the anthrone reagent, according to the protocol used for analysis of soluble sugars.
Calculation and statistical analysis
The survival ratio was calculated as the number of living plants divided by the total number of plants in a given treatment. The number of SAR per plant was calculated as the number of PAR multiplied by the average number of SAR in each PAR. To test the effects of the water levels on the growth, survival, and concentrations of the soluble sugars and starch, we conducted repeated-measure ANOVAs using water levels as a dependent factor and time as a repeated-measure factor (Ding et al. Citation2017). All statistical analyses were performed using SPSS V17.0 (IBM Corp., Armonk, NY, USA).
Results
Plant biomass
Compared with the plants grown in the 0 and 25 cm water levels, the plants grown in the 50 cm water level had lower leaf, stem, root, and total biomass values (P< 0.001; ). In the treatments with the 0 and 25 cm water levels, the plant biomass remained relatively constant in the early stage of the experiment, but increased rapidly afterwards, whereas in the treatments with the 50 cm water levels, they remained similar during the entire experimental period. For instance, the total biomass of plants grown in the 0 cm water level was approximately 12.0 g plant−1 after 50 and 80 days of the treatment, and it increased to 18.0 and 35.7 g plant−1 after 110 and 140 days of the treatment, respectively. In treatments with the 50 cm water level, the total biomass was on average was 7.6 g plant−1 during the whole experimental period.
Growth parameters
Water level and duration of the experiment had significant effects on plant height, length of the longest root, length and number of the PAR, and number of the SAR, and both variables had interactive effects on these parameters (P< 0.05; ). These growth parameters were the greatest in treatments with 0 cm water level, and the lowest in treatments with 50 cm water level, after 80, 110, and 140 days. In the 0 and 25 cm water level treatments, the plant height, length of the longest root, length and number of the PAR, and number of the SAR gradually increased as the experiment continued. However, in treatments with the 50 cm water level, these parameters remained relatively constant throughout the experiment.
Figure 2. Survival ratio, plant height, length of the longest root, length and number of the primary adventitious roots (PAR), number of the secondary adventitious roots (SAR) (means ± SE) of Salix triandroides cuttings growing in three water-level regimes (0, 25, and 50 cm above the soil surface) throughout the experimental period.
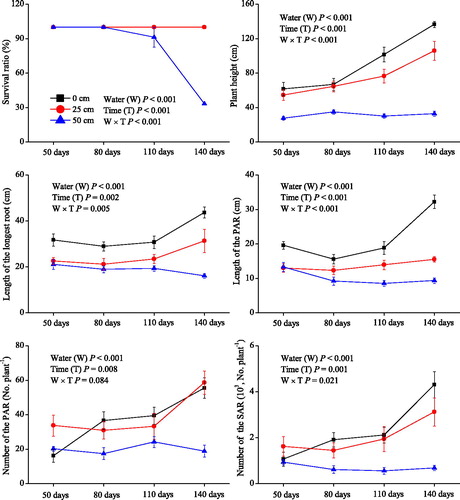
Survival ratio
The survival ratio was significantly affected by both the water level and treatment time (P< 0.001; ). In the treatments with the 0 and 25 cm water levels, the survival ratio was 100% during the entire experimental period; in the treatments with the 50 cm water level, it remained 100% after 50 and 80 days of the experiment, but decreased to 91.7 and 33.3% after 110 and 140 days of the treatment, respectively.
Non-structural carbohydrate concentration
Compared with the plants grown in the 0 and 25 cm water levels, the plants grown in the 50 cm water level had lower soluble sugar and starch concentrations (P< 0.001; ). At the 0 and 25 cm water levels, the soluble sugar and starch concentrations were high for the entire experimental period. For example, as the experiment progressed, in the above ground plant parts for the 0 cm water level treatments, the soluble sugar concentrations were above 37.1 mg g−1 and those of starch were above 46.1 mg g−1. However, in the treatments with the 50 cm water level, both the soluble sugar and starch concentrations gradually decreased as the experiment progressed, and the soluble sugar concentrations were positively associated with the starch concentrations in both the aboveground and underground plant parts.
Discussion
The leaf, stem, root, and total biomass values were high in the control (0 cm water level), intermediate in plants exposed to incomplete submergence (25 cm water level), and low in the completely submerged plants (50 cm water level). These results supported the first hypothesis, which stated that submergence inhibits plant growth, and corroborated the results previously reported for other emergent plants in the Dongting Lake wetlands (Pan et al. Citation2012). When exposed to flooding, plants exhibit two contrasting survival strategies, escape or quiescence. The submerged plants escape flooding by shoot elongation, elevating the shoot above the water surface to capture the aerial light and oxygen; this strategy is advantageous in shallow water environments (Bailey-Serres and Voesenek Citation2008; Perata and Voesenek Citation2007). The second strategy, is that submerged plants decrease their growth rate to maintain low energy demands, and this is beneficial in deep water flooding (Luo et al. Citation2011; Yu et al. Citation2012). Plant height and stem biomass with the incomplete submergence treatments were different from the control during the early stages of submergence (). This suggests that plants increased their investment in stem growth early in the submergence period to escape flooding conditions (Jackson Citation2008; Visser et al. Citation2003). In the complete submergence, plant biomass, including that of the leaf, stem, and root, and growth parameters such as plant height and root length were maintained continuously at low levels during the whole experiment. This indicates that plants inhibited their growth and employed the quiescence strategy under the flooding conditions (Li et al. Citation2007; Voesenek and Bailey-Serres Citation2015).
In the control and incomplete submergence treatments, the plants survival rate was 100% throughout the experiment, whereas the survival ratio of the completely submerged plants decreased to 91.7% after 110 days and 33.3% after 140 days of the treatment. The low survival ratio is attributed to the oxygen and light limitations, that subsequently restrict respiration and photosynthesis in completely submerged plants (Voesenek et al. Citation2006; van Veen et al. Citation2016). With increasing water levels (i.e., complete submergence), the oxygen content decreases rapidly and presents an important limiting factor for plant survival (Sauter Citation2013; Loreti et al. Citation2016). Ding et al. (Citation2017) showed that with increased water levels subsequent decreased effectiveness of oxygen, S. triandroides can form aerenchyma, thus increasing their porosity and increasing their ability to absorb oxygen from the water. The metabolism of stored non-structural carbohydrates may be more crucial for plant survival (Luo et al. Citation2011; Striker Citation2012; Yu et al. Citation2012). In the present study, the control plants and those that were subjected to incomplete submergence maintained high concentrations of soluble sugars and starch in their aboveground and underground parts, but the plants that were exposed to complete submergence exhibited significant decreases of both soluble sugars and starch levels as the submergence persisted (). Under conditions of complete submergence, the low levels of photosynthesis cannot supply enough non-structural carbohydrates (Peña-Fronteras et al. Citation2009; Voesenek and Bailey-Serres Citation2015). As hypothesized, the plant survival ratio decreased with the reducing non-structural carbohydrate concentrations. When the mean concentration of soluble sugars and starch in the completely submerged plants was reduced to 19.2 and 23.5%, respectively, of that found in the control and the plant survival ratio decreased to 33.3%. Therefore, the survival of the completely submerged Salix triandroides cuttings is associated with the metabolism of non-structural carbohydrates (Jackson et al. Citation2009; Peña-Fronteras et al. Citation2009; Yu et al. Citation2012).
The present study showed that submergence had a significant effect on plant growth, plant survival, and the metabolism of non-structural carbohydrates. Plants that were incompletely submerged, successfully adopted the escape strategy of obtaining aerial light and oxygen, that ensured adequate biomass, high survival ratios, and high concentrations of soluble sugars and starch. In contrast, the completely submerged plants adopted the quiescence strategy, as they decreased their energy demand, leading to lower biomasses, reduced survival ratios, and low concentrations of soluble sugars and starch. However, unlike the constant water level in the experimental treatment, the water level in the natural conditions is often variable. Once completely submerged plants emerge from the water in a short period, rapidly accumulated non-structural carbohydrates increase the plants’ tolerance to flooding (Qin et al. Citation2013). In addition, the intensity of the complete submergence, such as water levels 20 and 50 cm above the plant, may also influence the metabolism of non-structural carbohydrates. Further research will be required to explore the links between plant survival and metabolism of nonstructural carbohydrates under these water regimes.
Notes on contributors
Xiaohui Ding is a postgraduate in Hunan Agricultural University, China. Her major is ecology. She is interested in aquatic ecology, wetland plants, and freshwater ecology.
Jia Luo is an associated professor in Hunan Academy of Forestry, China. Her major is ecology. She is interested in plant ecolgy, water quality, wetland restoration, and hydrology.
Youzhi Li is an associated professor in Hunan Agricultural University, China. His major is ecology. He is interested in wetland plants, wetland pollutants, wetland restoration and biodoversity protection.
Bo Ren is an associated professor in Hunan Agricultural University, China. His major is ecology. He is interested in climate change, crop ecology, and wetland plants.
Hualin Bian is a postgraduate in Hunan Agricultural University, China. He major is ecology. He is interested in aquatic ecology, wetland plants, and ecosystem function assessments.
Xin Yao is a postgraduate in Hunan Agricultural University, China. Her major is ecology. She is interested in aquatic ecology, wetland pollutants, and biodiversity protection.
Qiaoqiao Zhou is a postgraduate in Hunan Agricultural University, China. Her major is ecology. She is interested in hydrology, aquatic ecology and biodiversity protection.
Disclosure statement
No potential conflict of interest was reported by the authors.
Additional information
Funding
References
- Anderegg WRL, Berry JA, Field CB. 2012. Linking definitions, mechanisms, and modeling of drought-induced tree death. Trends Plant Sci. 17(12):693–700.
- Bailey-Serres J, Voesenek L. 2008. Flooding stress: acclimations and genetic diversity. Annu Rev Plant Biol. 59(1):313–319.
- Béjaoui Z, Mguis K, Abassi M, Albouchi A, Lamhamedi MS. 2016. Involvement of carbohydrates in response to preconditioning flooding in two clones of Populus deltoides Marsh.×P. nigra L. J Plant Growth Regul. 35(2):492–503.
- Das KK, Sarkar RK, Ismail AM. 2005. Elongation ability and non-structural carbohydrate levels in relation to submergence tolerance in rice. Plant Sci. 168(1):131–136.
- Ding X, Zou J, Li Y, Yao X, Zou D, Zhang C, Yang N, Niu Y, Bian H, Deng J, Ge Z. 2017. Acclimation of Salix triandroides cuttings to incomplete submergence is reduced by low light. Aquat Ecol. 51(2):321–330.
- Du H, Wu N, Cui F, You L, Li X, Xiong L. 2014. A homolog of ETHYLENE OVERPRODUCER, OsETOL1, differentially modulates drought and submergence tolerance in rice. Plant J. 78(5):834–849.
- Gimeno V, Syvertsen JP, Simón I, Nieves M, Díaz-López L, Martínez V, García-Sánchez F. 2012. Physiological and morphological responses to flooding with fresh or saline water in Jatropha curcas. Environ Exp Bot. 78:47–55.
- Jackson MB, Ishizawa K, Ito O. 2009. Evolution and mechanisms of plant tolerance to flooding stress. Ann Bot. 103(2):137–142.
- Jackson MB. 2008. Ethylene-promoted elongation: an adaptation to submergence stress. Ann Bot. 101(2):229–248.
- Kurzatkowski D, Leuschner C, Homeier J. 2015. Effects of flooding on trees in the semi-deciduous transition forests of the Araguaia floodplain, Brazil. Acta Oecol. 69:21–30.
- Lee BR, Jin YL, Jung WJ, Avice JC, Morvan-Bertrand A, Ourry A, Park CW, Kim TH. 2008. Water-deficit accumulates sugars by starch degradation-not by de novo synthesis-in white clover leaves (Trifolium repens). Physiol Plant. 134(3):403–411.
- Li Y, Xie Y, Ren B, Luo W, Huang J. 2007. Oxygen enhances the recovery of Potamogeton maackianus from prolonged exposure to very low irradiance. Aquat Bot. 86(3):295–299.
- Li Y, Li X, Zhang C, Wan X. 2014. Change trend of Salix Ligneous plants in the Dongting Lake wetlands and its reasons. Wetlands Sci. 12:646–649 (in Chinese).
- Loreti E, Yamaguchi J, Alpi A, Perata P. 2003. Sugar modulation of α-amylase genes under anoxia. Ann Bot. 91(2):143–148.
- Loreti E, van Veen H, Perata P. 2016. Plant responses to flooding stress. Curr Opin Plant Biol. 33:64–71.
- Luo FL, Nagel KA, Scharr H, Zeng B, Schurr U, Matsubara S. 2011. Recovery dynamics of growth, photosynthesis and carbohydrate accumulation after de-submergence: a comparison between two wetland plants showing escape and quiescence strategies. Ann Bot. 107(1):49–63.
- Mishra SK, Patro L, Mohapatra PK, Biswal B. 2008. Response of senescing rice leaves to flooding stress. Photosynthetica. 46(2):315–317.
- Mohanty HK, Mallik S, Grover A. 2000. Prospects of improving flooding tolerance in lowland rice varieties by conventional breeding and genetic engineering. Curr Sci. 78:132–140.
- Noodéb LD, Penney JP. 2011. Correlative controls of senescence and plant death in Arabidopsis thaliana (Brassicaceae). J Exp Bot. 52:2151–2159.
- Qin X, Li F, Chen X, Xie Y. 2013. Growth responses and non-structural carbohydrates in three wetland macrophyte species following submergence and de-submergence. Acta Physiol Plant. 35(7):2069–2074.
- Pan Y, Xie Y, Chen X, Li F. 2012. Effects of flooding and sedimentation on the growth and physiology of two emergent macrophytes from Dongting Lake wetlands. Aquat Bot. 100:35–40.
- Peña-Fronteras JT, Villalobos MC, Baltazar AM, Merca FE, Ismail AM, Johnson DE. 2009. Adaptation to flooding in upland and lowland ecotypes of Cyperus rotundus, a troublesome sedge weed of rice: tuber morphology and carbohydrate metabolism. Ann Bot. 103(2):295–302.
- Perata P, Voesenek L. 2007. Submergence tolerance in rice requires Sub1A, an ethylene-response-factor-like gene. Trends Plant Sci. 12(2):43–46.
- Perata P, Armstrong W, Voesenek L. 2011. Plants and flooding stress. New Phytol. 190(2):269–273.
- Rodríguez-Calcerrada J, Shahin O, Rey MC, Rambal S. 2011. Opposite changes in leaf dark respiration and soluble sugars with drought in two Mediterranean oaks. Funct Plant Biol. 38(12):1004–1015.
- Rodríguez-Gamir J, Ancillo G, González-Mas MC, Primo-Millo E, Iglesias DJ, Forner-Giner MA. 2011. Root signalling and modulation of stomatal closure in flooded citrus seedlings. Plant Physiol Biochem. 49(6):636–645.
- Sauter M. 2013. Root responses to flooding. Curr Opin Plant Biol. 16(3):282–286.
- Striker GG. 2012. Time is on our side: the importance of considering a recovery period when assessing flooding tolerance in plants. Ecol Res. 27(5):983–987.
- van Veen H, Vashisht D, Akman M, Girke T, Mustroph A, Reinen E, Hartman S, Kooiker M, van Tienderen P, Schranz ME, et al. 2016. Transcriptomes of eight Arabidopsis thaliana accessions reveal core conserved, genotype- and organ-specific responses to flooding stress. Plant Physiol. 172:668–689.
- Visser EJW, Voesenek L, Vartapetian BB, Jackson MB. 2003. Flooding and plant growth. Ann Bot. 91:107–109.
- Voesenek L, Bailey-Serres J. 2015. Flood adaptive traits and processes: an overview. New Phytol. 206(1):57–73.
- Voesenek L, Colmer TD, Pierik R, Millenaar FF, Peeters A. 2006. How plants cope with complete submergence. New Phytol. 170(2):213–226.
- Xie YH, Tang Y, Chen XS, Li F, Deng ZM. 2015. The impact of three Gorges Dam on the downstream eco-hydrological environment and vegetation distribution of East Dongting Lake. Ecohydrology. 8(4):738–746.
- Ye XQ, Zeng B, Meng JL, Wu M, Zhang XP. 2018. Responses in shoot elongation, carbohydrate utilization and growth recovery of an invasive species to submergence at different water temperatures. Sci Rep. 8(1):306.
- Yoshida S, Forno DA, Cock JH, Gomez KA. 1976. Laboratory manual for physiological studies of rice. Los Baños, Philippines: IRRI.
- Yu X, Luo N, Yan J, Tang J, Liu S, Jiang Y. 2012. Differential growth response and carbohydrate metabolism of global collection of perennial ryegrass accessions to submergence and recovery following de-submergence. J Plant Physiol. 169(11):1040–1049.