Abstract
Understanding patterns in trace element concentrations and water quality within river systems provides a foundation to evaluate retrospective movements and habitat use of fish. Because trace elements are incorporated into calcified structures of fishes relative to water concentrations, baseline maps have application for fisheries management, including for rare species such as sturgeons. Therefore, trace elements [strontium (Sr), barium (Ba), manganese (Mn), zinc (Zn), and magnesium (Mg)], strontium isotopes (87Sr/86Sr), dissolved oxygen and salinity were measured in the Pearl River and Lake Pontchartrain watersheds, within Mississippi and Louisiana, USA for application in assessing riverine use by Gulf Sturgeon Acipenser oxyrinchus desotoi. Water samples were collected during the summer-fall period when juvenile Gulf Sturgeon were anticipated to be present and analyzed by solution and multi-collector inductively coupled plasma mass spectrometry. Trace element to calcium (Ca) ratios in Sr, Ba, Zn, and Mg were distinguishable between the upper Pearl River and the Bogue Chitto River tributary, and the upper Pearl River and lower Pearl River. For most trace elements and 87Sr/86Sr, there was a gradual increase from upper river regions to lower river regions, and large differences between freshwater and saline areas. Lake Pontchartrain watershed rivers were not easily differentiated, but regions of low dissolved oxygen concentrations in the lower reaches suggest available Gulf Sturgeon habitat may be seasonally limited. Therefore, maps of water microchemistry in the Pearl River watershed allow for retrospective analyses of fish watershed use.
1. Introduction
Within a watershed, the chemistry of surface water is influenced by bedrock composition, soil, land use and atmospheric inputs. Weathering and land use result in inputs of elements and isotopes to surface waters relative to their abundance in surrounding substrates. Concentrations of elements in surface waters may provide a unique signature for specific watersheds and locations within a watershed, particularly when elements found at trace concentrations are evaluated (Asante et al. Citation2008; Dkhar et al. Citation2014). Trace elements vary greatly with changes in geological and geographical factors and are defined as any element having an average concentration of less than 100 parts per million (ppm) (McNaught and Wilkinson Citation1997; Richir and Gobert Citation2016). Trace elements have been shown to accumulate in the liver, gills, otoliths, and calcified structures of many fish species, and incorporation can vary based on individual physiology as well as environmental factors (Gilanders Citation2001; Gilbert et al. Citation2017; Pracheil et al. Citation2019).
Analysis of incorporated trace elements can be applied in a variety of ways, such as stock identification and bioindication of toxins (Gilanders Citation2001; Pracheil et al. Citation2014; Gilbert et al. Citation2017). Additionally, trace element analyses of calcified structures are used to retrace life history changes in anadromous fish species, and are particularly useful for understanding movements and life history characteristics of rare or endangered species when validated by reference samples (Secor et al. Citation1995; Elsdon and Gillanders Citation2003; Allen et al. Citation2009).
Strontium (Sr), calcium (Ca), and barium (Ba) are common trace elements analyzed in aquatic systems because of their abundance and variability between fresh water and seawater (Capo et al. Citation1998; Secor and Rooker Citation2000; Allen et al. Citation2009). Strontium is a relatively high mass element that is found ubiquitously in nature in four naturally occurring isotopes: 84Sr, 86Sr, 87Sr and 88Sr (Capo et al. Citation1998). Concentrations of 86Sr do not change by radioactive decay, unlike 87Sr, which is produced by the decay of rubidium (87Rb) (half-life ∼ 48.8 × 109 years; Capo et al. Citation1998). Consequently, quantifying the ratio of 87Sr/86Sr accurately identifies the age of geological (Graustein Citation1989) and biological structures (Kennedy et al. Citation2000; Hobbs et al. Citation2007). Strontium in bedrock is released naturally due to weathering, where it accumulates proportionately in local flora and fauna (Capo et al. Citation1998; Price et al. Citation2002). As a result, ratios of Sr in conjunction with Ca and Ba are useful for linking organisms with site-specific regions (Nakano Citation2016). Ecological studies have utilized this technique to describe migratory (Price et al. Citation2002) and seasonal ontogenetic patterns (Secor et al. Citation1995; Hobbs et al. Citation2010), and scalar life cycles (Secor and Rooker Citation2000). To facilitate such studies, it is imperative to develop a representative data set of environmental levels of Sr and other trace elements in aquatic systems.
Sturgeons belong to the Division Chondrostei, Order Acipenseriformes, and Family Acipenseridae (Helfman et al. Citation1997). They are long-lived species, and some of the largest fishes occupying river systems (Helfman et al. Citation1997). In the past, sturgeons were the focus of many commercial fisheries which resulted in the near extirpation of several species (Pikitch et al. Citation2005). As a result, their conservation and management have become a primary focus of regulatory entities (Chebanov and Savelyeva Citation1999; Billard and Lecointre Citation2000). A critical component to conservation of threatened and endangered species, such as sturgeons, is understanding life history movements (Woolnough et al. Citation2009; Claudet et al. Citation2010). Life history movements are often identified with telemetry studies (Cooke et al. Citation2008), but cost, rarity of study organisms and logistical considerations may preclude use of this technology. Similarly, telemetry is often an inviable option when working with endangered species, as capture, surgery, and transmitter application may be harmful (Nielsen Citation1998; Godfrey et al. Citation2003). Trace elements have been demonstrated to be a useful technique for identifying life history traits in sturgeons (Allen et al. Citation2009, Citation2018a).
The Gulf Sturgeon Acipenser oxyrhynchus desotoi is an endemic species to the northern Gulf of Mexico, extending from the Suwanee River in Florida to the Pearl River in eastern Louisiana (Havrylkoff et al. Citation2012). This species is listed as threatened under the U.S. Endangered Species Act, primarily due to habitat loss through damming and river fragmentation, and overfishing (USFWS and GSMFC Citation1995; Heise et al. Citation2005). In the spring, Gulf Sturgeon migrate to coastal rivers to spawn (Fox et al. Citation2000, Citation2002; Craft et al. Citation2001; Harris et al. Citation2005; Duncan et al. Citation2011), where they remain for the duration of the summer (Duncan et al. Citation2011). Young-of-the-year spend 9–10 months feeding in these rivers before they appear in estuaries between December and February (Sulak and Clugston Citation1998; Havrylkoff et al. Citation2012). Juveniles are believed to overwinter in estuaries (Sulak and Clugston Citation1998, Citation1999; Sulak and Randall Citation2002; Sulak et al. Citation2009; Havrylkoff et al. Citation2012) before returning to freshwater habitats in the summer months to seek thermal refuge from warm saline habitats (Hightower et al. Citation2002). Past research on Gulf Sturgeon has primarily focused on broad-scale patterns of life history (Havrylkoff et al. Citation2012). Less is known of the early life history stages, particularly in terms of habitat use within freshwater and the timing of emigration to saline habitats.
Trace element analyses of pectoral fin rays, which can be sampled non-lethally (Collins and Smith Citation1996; Allen et al. Citation2018b), are used to determine the timing of seawater entry in A. medirostris (Green Sturgeon) (Allen et al. Citation2009), A. gueldenstaedti (Russian Sturgeon) (Arai et al. Citation2002), and A. transmontanus (White Sturgeon) (Veinott et al. Citation1999), and predict natal origin within freshwater in Scaphirhynchus platorynchus (Shovelnose Sturgeon; Phelps et al. Citation2012; Pracheil et al. Citation2018), S. albus (Pallid Sturgeon; Phelps et al. Citation2012), and A. fulvescens (Lake Sturgeon) (Phelps et al. Citation2017). These techniques were found to be useful when identifying the spawning location, age determination, and growth of A. oxyrinchus oxyrinchus (Atlantic Sturgeon) (Stevenson and Secor Citation2000; Balazik et al. Citation2012) and in A. brevirostrum (Shortnose Sturgeon) (Altenritter et al. Citation2015). Strontium (Sr) isotopes, are also used to identify natal spawning areas of freshwater fishes (Hobbs et al. Citation2007; Hobbs et al. Citation2010), and contemporary studies have used them to distinguish freshwater movements of juvenile Gulf Sturgeon in the Choctawhatchee River System (Allen et al. Citation2018a). Trace element and Sr isotopes analysis are potentially useful for other river systems occupied by Gulf Sturgeon, such as the Pearl River System in Louisiana and Mississippi where little is known of movements and habitat use of juveniles.
Gulf Sturgeon have historically been collected in the Pearl and Bogue Chitto Rivers and several tributaries of Lake Pontchartrain (Rogillio Citation1992; Morrow et al. Citation1996, Citation1998; Rogillio et al. Citation2001; Rogillio et al. Citation2007). A study conducted by Rogillio et al. (Citation2007) found summer habitats in the Pearl and Bogue Chitto Rivers from April through November, with adults concentrating around the Bogue Chitto River and subadults around the Pearl River. Adults were observed to congregate in the Mississippi Sound between November and March (Rogillio et al. Citation2007). Spawning locations are thought to be in the Pearl or Bogue Chitto Rivers but have not been conclusively identified (Rogillio et al. Citation2007). Adult Gulf Sturgeon have been found in Lake Pontchartrain and the Amite River, and there is speculation the lake and surrounding rivers are important habitats (Rogillio et al. Citation2007).
Identifying trace elements and strontium isotope gradients has the potential to provide useful baseline information for application to fisheries conservation within the Pearl River Watershed. Based on a contemporary study on the Choctawhatchee River System (Allen et al. Citation2018a), it is anticipated that trace element and 87Sr/86Sr isotope ratios in calcified structures of fishes will be beneficial for life history reconstruction within the Pearl River System. This information is beneficial for resource managers for use in assessing changes within watersheds and identifying ontogenetic patterns, anadromous movements, and life history characteristics of Gulf Sturgeon and other fishes. Therefore, the objectives of this study are to analyze water in the Pearl and Bogue Chitto Rivers, and drainages supplying Lake Pontchartrain for: (1) concentrations of trace elements and (2) 87Sr/86Sr, and (3) to make baseline maps of patterns within watersheds using these data for application to fisheries management.
2. Methods
2.1. Study area
The Pearl River Basin covers southeast Louisiana and southwest Mississippi (). The Pearl River originates in the east-central area of Mississippi, flows for a total of 790 km in a 22,690 km2 drainage basin, and discharges into the Gulf of Mexico via the Mississippi Sound (Cai et al. Citation2016). The basin area is dominated by forest, but also includes agricultural land and wetlands (Mississippi Department of Environmental Quality Citation2007; Cai et al. Citation2016). It is joined by the Bogue Chitto River, a 102 km river originating in Southern Mississippi, 16 km downstream from a navigation sill purportedly blocking most sturgeon passage in the Pearl River (Rogillio et al. Citation2007). The Pearl River has a braided delta region, which discharges near the outflow of Lake Pontchartrain.
Figure 1. Map of study area showing the (1) Pearl River, (2) Bogue Chitto River, (3) Tchefuncte River, (4) Tangipahoa River, (5) Tickfaw River, (6) Amite River, (7) Lake Pontchartrain and (8) Lake Maurepas. Light gray lines show United States Department of Agriculture Major Land Use Resource Area boundaries.
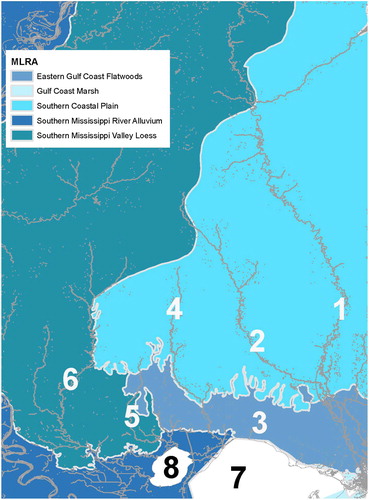
The Lake Pontchartrain Basin covers 24,080 km2 and drains from 11 major counties in Louisiana and five in Mississippi (Alford Citation2014; Liu et al. Citation2014). The major water body of the basin is Lake Pontchartrain, located in southeastern Louisiana (Chao et al. Citation2012; Liu et al. Citation2014). The lake is 1600 km2, a shallow water body with low salinity ranging from 0 to 2 ppt (Chao et al. Citation2012; Liu et al. Citation2014). Lake Pontchartrain is supplied by spring creeks along the northern and western edges and connects to the Gulf of Mexico along the eastern edge through three narrow tidal channels (Liu et al. Citation2014). The major drainages feeding into Lake Pontchartrain, from west to east, include the Amite, Tickfaw, Tangipahoa, and Tchefuncte Rivers (Alford Citation2014).
2.2. Water sample collection
Water sampling locations spanned the region of potential Gulf Sturgeon spawning and juvenile habitat use on the Pearl and Bogue Chitto Rivers and associated drainages connected to Lake Pontchartrain, notably the Tchefuncte, Tangipahoa, Tickfaw and Amite Rivers in Louisiana and Mississippi (). Water samples were collected during the late summer and early fall period when juvenile Gulf Sturgeon were anticipated to be in the watershed. High river runoff following precipitation events were avoided to assess typical conditions. Over 30 samples were collected from both the Pearl and Bogue Chitto Rivers, and ≥5 samples from each of the four associated drainages. At each sampling location, dissolved oxygen and salinity were measured using a YSI85 (YSI, Inc., Yellow Springs, OH), and global positioning satellite (GPS) coordinates were recorded and a digital picture of the site was taken using a GPS instrument (GPSMAP 62sc, Garmin International, Inc., Olathe, KS). Water was collected approximately 30 cm below the surface using an extendable pole with a 1-L plastic container, which was rinsed twice at each site before collecting a water sample. Water was placed into 125-mL sample containers previously triple rinsed with ultrapure water and air dried. Water samples were acidified to pH <2 using 16 N trace element grade nitric acid (Fluka TraceSelect, Sigma Aldrich, St Louis., MO), filtered (0.45 µm, VWR, Radnor, PA) and stored at 4 °C prior to analyses.
2.3. Water sample analysis
Water samples were evaluated for trace elements using solution inductively coupled plasma mass spectrometry (ICPMS) to determine the elements with the greatest changes between freshwater, estuarine and oceanic areas. Trace element analyses included 44Ca, 26 Mg, 86Sr, 137Ba, 55Mn, 66Zn and 208Pb. A new wave UP-213 laser ablation system with a quadrupole mass spectrometer (Agilent technologies, Santa Clara, CA) was used, with wash time of 60 s, take-up time of 120 s, argon as the sample, cooling, auxiliary gas and 4 runs/10 passes for low resolution elements and 3 runs/10 passes for medium resolution elements.
Water samples were also analyzed for Sr isotopic (87Sr/86Sr) changes using a multi-collector (MC) ICPMS (Nu Plasma HR, Nu Instruments Ltd., Wrexham, UK) following methods by Willmes et al. (Citation2016). Samples were introduced with a desolvating nebulizer system (DSN-100), and computer software (Nu Plasma v.1.4.2011) calculated twice the standard error from the baseline as the detection limit and also identified outliers. Detectors (sapphire-cased graphite to gold contact faraday cups amplified by 1011 Ohm feedback resistors) were maintained in a pressure and temperature-controlled environment and calibrated every 24 h. 87Sr/86Sr data were internally normalized by the measured 86Sr/88Sr ratio relative to the ratio of natural abundances of 86Sr and 88Sr: 0.1194. 85Rb was measured to correct for the potential of small amounts of 87Rb present following Willmes et al. (Citation2016). Analyses were conducted at the University of California Davis Interdisciplinary Center for Inductively-Couple Plasma Mass Spectrometry based on methods developed at the facility.
2.4. Baseline map development
Mapping included use of National Hydrography Data set Plus v. 2 to map aquatic features of water chemistry maps and Major Land Use Resource Areas (MLRAs; USDA NRCS Citation2006) were used to map terrestrial areas. Each MLRA integrates land use, geologic, and other features that can confer an initial impression of differences in water chemistry. To determine spatial patterns of water chemistry measurements, water chemistry points were overlaid onto the map containing aquatic features and MLRAs. The MLRA layers were then used to identify soil type and land use in each area of the watersheds.
2.5. Statistical analysis
Water in the freshwater portion of rivers were compared for trace elements and strontium isotope concentrations using one-way analysis of variance (ANOVA). In the Pearl River, the Upper Pearl River, the Bogue Chitto River (the main tributary) and Lower Pearl River were compared. In the Lake Pontchartrain watershed, the Tchefuncte, Tangipahoa, Tickfaw and Amite Rivers were compared. Data were tested for outliers using a Dixon’s Q-test (Rorabacher Citation1991) and one outlier was removed from the Lower Pearl River for Mg, from the Bogue Chitto River for Zn, and the Amite River for Ba and Zn. ANOVA assumptions of normality and homogeneity of variance were examined on residuals using Shapiro–Wilk tests and Levene’s tests, respectively. Data were transformed using Log10 transformations to meet ANOVA assumptions where possible, and means were compared using Tukey’s post hoc tests. If data could not be transformed to meet ANOVA assumptions, non-parametric Kruskal–Wallis tests were used, with means compared by Dunn’s post-hoc tests. In all cases, differences among means were considered significant at alpha = 0.05 using JMP statistical software (v.12.2.0).
3. Results
The Pearl and Bogue Chitto Rivers, and four smaller rivers draining into Lake Pontchartrain, the Tchefuncte, Tangipahoa, Tickfaw, and Amite Rivers, were analyzed for Sr/Ca, Ba/Ca, Mn/Ca, Zn/Ca, Mg/Ca and 87Sr/86Sr.
3.1. Pearl and Bogue Chitto Rivers
The geology of the upriver areas of the Pearl and Bogue Chitto Rivers is made up primarily of Citronelle formation, and Pascagoula and Hattiesburg formation (Thompson Citation2011). The Citronelle formation is red sand and gravel with white clay, whereas the Pascagoula and Hattiesburg formation are primarily green and blue-green, sandy clay, sand, and siltstone. The minerals in the upriver areas of both of these rivers tend to be primarily sand and clay with some areas of gravel-bearing strata incorporated (Thompson Citation2009). The land area around the upriver portions of the Pearl and Bogue Chitto Rivers consists of Southern Mississippi Valley Loess and Southern Coastal Plain (). Both contain Entisols, Inceptisols, and Ultisols soils, with Alfisols also found in the Loess region (USDA NRCS Citation2006).
The mineral makeup of the lower Pearl River is in an area of heavy mineral occurrence and coastal deposits, which are made up of loam, sand, gravel, and clay (Thompson Citation2009, Citation2011). The downriver section of the Pearl River below the sill dam at Bogalusa, LA, near the confluence with the Bogue Chitto River, or the lower Pearl River, is in an area of Eastern Gulf Coast Flatwoods, which consists of Alfisols, Ultisols, Entisols, Spodosols, and Histosols (USDA NRCS Citation2006).
The upriver portion of the Pearl River, or the upper Pearl River, from the outflow of Ross Barnett Reservoir to the sill dam near Bogalusa, LA, had dissolved oxygen saturation (%) and concentration (mg/L) similar to the Bogue Chitto River, which is actually a tributary to the Pearl River (). The lower Pearl River had lower dissolved oxygen saturation and concentration, and higher salinity than either the upper Pearl River or the Bogue Chitto River (). Dissolved oxygen was comparable in upper river areas to upper river areas of the Lake Ponchartrain watershed rivers ().
Table 1. Mean ± standard error water quality data for upriver (UR) and downriver (DR) sections of the Pearl and Bogue Chitto rivers.
Table 2. Mean ± standard error water quality data for upriver (UR) and downriver (DR) sections of Lake Pontchartrain rivers: the Tchefuncte, Tangipahoa, Tickfaw, and Amite.
In terms of patterns observed in trace elements, in the Pearl River, Sr/Ca was low in upriver regions and gradually increased downriver reaching highest concentrations in lower river regions of salinity intrusion (). The Bogue Chitto River had a similar pattern of low Sr/Ca in the upriver region, increasing downriver until its confluence with the Pearl River. Values of Sr/Ca were higher in the Bogue Chitto River (8.606–10.564 mol Sr/mmol Ca) than the upper Pearl River (5.034–6.575 mol Sr/mmol Ca) and most of the lower Pearl River (6.576–8.605 mol Sr/mmol Ca) as well. Near the mouth of the Pearl River, Sr/Ca increased (14.657–20.660 mol Sr/mmol Ca) coincident with increases in salinity.
Figure 2. Sr/Ca (left panel) and 87Sr/86Sr (right panel) maps of the Pearl River and Lake Pontchartrain Watershed areas.
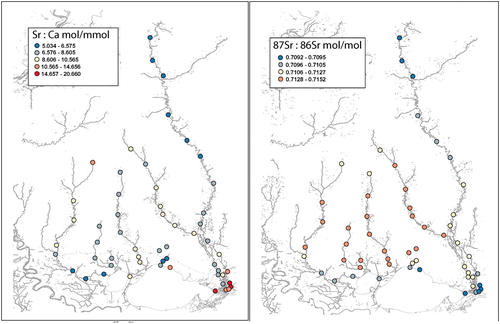
In the Pearl River, 87Sr/86Sr had a clear pattern of gradual increase from upriver to downriver, with low values found at the river mouth in the area of saline intrusion (). In the Bogue Chitto River, 87Sr/86Sr increased gradually downriver, and was higher (0.711621-0.712768 mol 87Sr/mol 86Sr) than values in most of the upper and lower Pearl River (generally <0.711620).
In the Pearl River, Ba/Ca was low (2.553–7.535 mol Ba/mmol Ca) in upriver regions, increased slightly (7.536–12.520 mol Ba/mmol Ca) near the northeast corner of Louisiana to the lower river, and decreased (0.025–2.552 mol Ba/mmol Ca) near the river mouth where it mixed with salinity (). The Bogue Chitto River had higher Ba/Ca (12.521–19.717 mol Ba/mmol Ca) than the upper or lower Pearl River.
Figure 3. Ba/Ca (left panel) and Zn/Ca (right panel) maps of the Pearl River and Lake Pontchartrain Watershed areas.
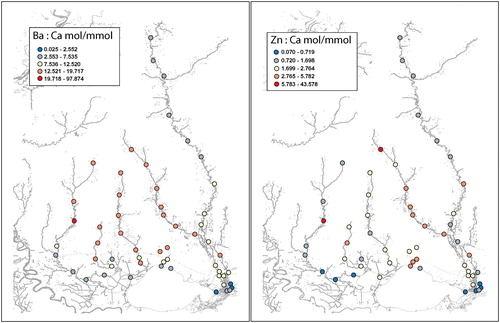
The pattern of Zn/Ca in the Pearl River was low upriver (0.720–1.698 mol Zn/mmol Ca), increasing gradually downriver, except near the mouth where values were very low (generally < 0.719 mol Zn/mmol Ca) (). In the Bogue Chitto River, a similar pattern of decreasing Zn/Ca as the river moved downstream was present, albeit values were higher than most of those in the Pearl River.
In the Pearl River, Mn/Ca was generally low in upriver areas, gradually increased downriver until it met with saline waters where values were very low (). The Bogue Chitto River had a different pattern, with generally higher Mn/Ca upriver and lower values downriver. Mn/Ca in the middle Pearl River region were generally similar to the Bogue Chitto River (2.765–5.782 mol Mn/mmol Ca), but upper Pearl River values were lower (11.823–28.391 mol Mn/mmol Ca).
Figure 4. Mn/Ca (left panel) and Mg/Ca (right panel) maps of the Pearl River and Lake Pontchartrain Watershed areas.
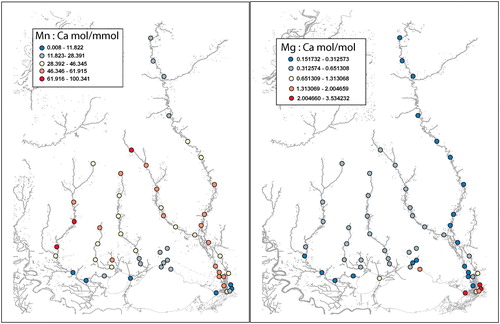
The pattern of Mg/Ca was low (0.151732–0.312573 mol Mg/mol Ca) throughout most of the upper and lower Pearl River, but increased sharply (2.004660–3.534232 mol Mg/mol Ca) near the mouth of the river in the area of saline intrusion (). The Bogue Chitto River had higher (0.312574–0.651308 mol Mg/mol Ca) Mg/Ca than the upper Pearl River (0.151732–0.312573 mol Mg/mol Ca).
In terms of statistical comparisons among freshwater portions of rivers, trace element and strontium isotope ratios differed among the Upper Pearl River, the Bogue Chitto River and the Lower Pearl River (). The Bogue Chitto River had greater values than the Upper and Lower Pearl River in Sr isotopes (K-W[2, 24], Chi-square: 18.2054, p < .001), Sr (F[2, 21] = 19.00, p < .001), Ba/Ca (K-W[2,24], Chi-square: 19.4342, p < .001), and Zn/Ca (F[2, 20] = 13.10, p < .001). Magnesium (Mg/Ca) differed among each river area (F[2, 20] = 56.87, p < .001) with highest values in the Bogue Chitto River, moderate values in the Lower Pearl River, and lowest values in the Upper Pearl River.
Table 3. Mean ± standard error strontium isotope and trace element data for freshwater portions of Pearl River and Lake Pontchartrain watershed rivers.
3.2. Lake Pontchartrain watershed rivers
The Tchefuncte, Tangipahoa, and Tickfaw Rivers are all in areas of gravel with a sandy matrix, surrounded by gravel in clayey or iron oxide matrix (Heinrich and McCulloh Citation1999). The mineral makeup of the upper Amite River is primarily sand and clay (Thompson Citation2009).
The Tchefuncte River, the downriver portion of the Tangipahoa River, and the edge of the Tickfaw River are in Eastern Gulf Coast Flatwoods (). The upper Tangipahoa and upper Tickfaw Rivers are in areas of Southern Coastal Plain, and the majority of the Amite River along with the mid-drainage portion of the Tickfaw River are in Southern Mississippi Valley Loess.
The Amite River begins in Pascagoula and Hattiesburg formation area, whereas the Tchefuncte, Tangipahoa, and Tickfaw Rivers begin in High Terraces formations (Snead and McCulloh Citation1984). Each then moves into Prairie Terraces, and end in Alluvium areas (Snead and McCulloh Citation1984).
The upper Tchefuncte, Tangipahoa, Tickfaw, and upper Amite rivers all had similar patterns in water quality, with slightly higher dissolved oxygen (% saturation and concentration [mg/L]) in the upstream portions of the rivers. Salinity, conductivity (µS) and specific conductivity (µS) were low in all river areas, typical of freshwater, although there was some salinity intrusion at downriver portions of the Tickfaw and Amite Rivers, and low salinity present in the upper Amite River ().
In terms of patterns observed in trace elements, in the Amite and Tchefuncte Rivers, Sr/Ca generally decreased from upriver to downriver, whereas in the Tangipahoa and Tickfaw Rivers, Sr/Ca changed very gradually or increased slightly downriver (). The Tangipahoa and Amite Rivers are larger than the Tickfaw and Tchefuncte Rivers and changes within these rivers were clearer than the shorter rivers.
For all four rivers, 87Sr/86Sr was generally higher upriver and decreased downriver with lowest values near areas of saline intrusion (). 87Sr/86Sr was similar among upriver regions of all four rivers (0.710542–0.712768 mol 87Sr/mol 86Sr). The Amite River appeared to have greater intrusion of water from Lake Maurepas (drains into Lake Pontchartrain), based on lower 87Sr/86Sr in downriver areas than the other rivers.
In the Tchefuncte, Tangipahoa, Tickfaw, and Amite Rivers, Ba/Ca was similar among rivers with higher values in upriver areas (12.521–19.717 mol Ba/mmol Ca) decreasing downriver to low values (2.553–7.535 mol Ba/mmol Ca) near river mouths ().
Zn/Ca and Mn/Ca were generally higher in upriver areas decreasing to lower values near river mouths, although among river variability was relatively high ( and ). Some of the variability may be due to different soil layers and land uses (Amite River flows through urbanized region of Baton Rouge, LA) among rivers. All four tributary rivers were very similar in Mg/Ca (0.312574–0.651308 mol Mg/mol Ca), with the Tchefuncte and Amite Rivers decreasing (0.151732–0.312573 mol Mg/mol Ca) in lower river areas ().
In terms of statistical comparisons among freshwater portions of rivers, trace element and strontium isotope ratios differed among the rivers of the Lake Pontchartrain watershed (). The Tickfaw River was greater than the Amite River in Sr isotopes (K-W[3, 24], Chi-square: 11.4704, p = .009) and Mg/Ca (K-W[3, 24], Chi-square: 10.5389, p = .015). The Tchefuncte, Tangipahoa and Tickfaw Rivers were greater than the Amite River in Zn/Ca (F[3,19] = 12.4277, p < .001).
4. Discussion
4.1. Water chemistry
The upper Pearl River (above the Bogalusa sill dam) and the Bogue Chitto River had distinct trace element patterns, presumably facilitating the ability to differentiate fish derived from either drainage based on analysis of calcified structures. In the upper Pearl River, Sr/Ca, Ba/Ca, Zn/Ca, Mg/Ca and 87Sr/86Sr were all lower than values in the Bogue Chitto River. In addition, there were gradual decreasing gradients from upper to lower watershed for trace elements and Sr isotopes within each drainage, potentially allowing for detection of movements of fish. Differences in trace elements and Sr isotopes may be due to differing composition of geologic and mineral differences in underlying soil types (Thompson Citation2009, Citation2011). As the upper Pearl and Bogue Chitto Rivers move into the Southern Coastal Plain and Eastern Gulf Flatwoods with harder water and more dissolved solids, trace elements and 87Sr/86Sr increase. Dissolved oxygen was high and salinity was not detectable in either of these portions of the drainages, which would be beneficial for many species of fish.
In the lower Pearl River, trace element and Sr isotope patterns were more complex than in either the upper Pearl River or the Bogue Chitto River. The lower Pearl River is a braided channel with hydrology impacted by sill dams near Bogalusa, LA on the Pearl River and a sill dam on the Bogue Chitto River near its confluence with the Pearl River. In addition, there are a number of channel modifications that influence hydrology in the lower Pearl River. Trace element and Sr isotopes generally followed a decreasing gradient. In the lower Pearl River, trace element and Sr isotope ratios were influenced more strongly by the upper Pearl River than the Bogue Chitto River, with values often continuing the declining gradient observed in the upper Pearl River.
Unlike the upper Pearl and Bogue Chitto Rivers, the lower Pearl River is influenced by tidal cycles and salinity near the braided mouth of the river. Salinity rises and dissolved oxygen decreases to hypoxic levels (51% saturation) in areas of saltwater intrusion. The lower Pearl River is also characterized by a broader floodplain than the upper Pearl River, and a number of slow-moving, hypoxic, anastomosing channels through dense vegetation. Where saltwater intrusion occurs, Sr/Ca and Mg/Ca increase dramatically, while Zn/Ca, Mn/Ca, and Ba/Ca decrease to very low ratios. The Eastern Gulf Coast Flatwood areas generally have low dissolved solids and higher salinity than the other areas within the study (USDA NRCS Citation2006).
In the Lake Pontchartrain Watershed, the Tchefuncte, Tangipahoa, Tickfaw and Amite Rivers were generally similar in their trace element and Sr isotope composition. These rivers have much smaller watersheds than the Pearl River, and much lower discharge. The Amite River was the most different in trace element and Sr isotope patterns, compared to the other rivers. Differentiating trace element and Sr isotope patterns within these rivers would be more difficult, although for the Amite River, Sr/Ca, Mn/Ca, and Zn/Ca could potentially be used to differentiate water chemistry from the other three rivers. The Tchefuncte, Tangipahoa, and Tickfaw Rivers had few differences in trace element composition and therefore differentiation of fish habitat use within these rivers based on water chemistry would be difficult. Notably, the downriver areas of several of these rivers, particularly the Amite and Tchefuncte, were hypoxic. Hypoxia can impair development, respiratory metabolism, foraging activity, and growth rates in sturgeons and other fishes (Jobling Citation1995; Crocker and Cech Citation1996; Blevins Citation2011). It is possible that there is seasonal variability in dissolved oxygen in the lower portions of these rivers, although topographically, these areas are characterized by a low gradient and slow flow.
Use of trace element and Sr isotope watershed data has the potential to benefit management of species within the river systems studied, including Gulf Sturgeon. Strontium isotopes are incorporated into calcified tissues based on ratios present in the surrounding aqueous environment, with differentiation between habitat use based on otoliths and fin rays using 87Sr/86Sr ratios with differences as little as 0.0001 (Kennedy et al. Citation2000; Kennedy et al. Citation2002; Allen et al. Citation2018a). The ability to use trace element and Sr isotope ratios to retrospectively determine movements of reproductive adults as well as identify natal habitats of juveniles, would help to guide management actions. In the Choctawhatchee River system, trace element and 87Sr/86Sr analysis were useful in identifying spawning locations and within river habitat use (Allen et al. Citation2018b). Similarly, in the Pearl River Watershed, trace element analysis of fin rays (e.g. Allen et al. Citation2009; Balazik et al. Citation2012; Phelps et al. Citation2012; Altenritter et al. Citation2015; Loewen et al. Citation2016) could provide insight into areas now being utilized by these fish. Processes affecting recovery are important and their effects on movement and spawning need to be understood. For Gulf Sturgeon, spawning reaches within these river systems are relatively unknown, but may be in very discrete locations (Heise et al. Citation2005).
4.2. Synthesis
This study indicates trace element and Sr isotope patterns within the upper Pearl River and Bogue Chitto Rivers are distinct and would likely allow for retrospective analyses of fish habitat use from calcified structures, such as Gulf sturgeon fin rays. The gradual longitudinal gradients in trace elements and Sr isotopes may facilitate differentiating within-river habitat use. Large differences in trace element and Sr isotopes between freshwater and saline habitats would allow for clear identification of movements between these two habitat types, as has been demonstrated in green sturgeon (Allen et al. Citation2009) and other diadromous fish species (e.g. Kennedy et al. Citation2002; Hogan et al. Citation2014). Distinguishing retrospective habitat use of fish in the Lake Maurepas and Lake Pontchartrain drainages would be more difficult based on the similarity of trace element and Sr isotope ratios among rivers. Discharge in these rivers is much lower than in the Pearl River Watershed, and habitats in many of the lower rivers were typified by slow flow and hypoxia.
Acknowledgments
We thank J. Rueter, National Marine Fisheries Service and the Northern Gulf Institute for project funding and the US Department of Agriculture National Institute of Food and Agriculture and the Mississippi Agricultural and Forestry Experiment Station for funding for P.J.A.; M. Pulliam, B. Akers, and N. Young for administrative help; K. Kimmel and C. Doolittle, US Fish and Wildlife Service, Baton Rouge, LA, for assistance in collecting water samples from the lower Pearl River; J. Glessner at the University of California Davis Interdisciplinary Center for Plasma Mass Spectrometry for expertise and sample analysis; and C. Shirley and B. Chesser for assistance with sample preparation.
Disclosure statement
No potential conflict of interest was reported by the authors.
Data availability statement
The data supporting the findings of this study are available from the corresponding author upon reasonable request.
Additional information
Funding
References
- Alford BJ. 2014. Multi-scale assessment of habitats and stressors influencing stream fish assemblafes in the Lake Pontchartrain Basin, USA. Hydrobiologists. 738(1):129–146.
- Allen PJ, Baumgartner W, Brinkman E, Devries RJ, Stewart HA, Aboagye DL, Ramee SW, Ciaramella MA, Culpepper CM III, Petrie-Hanson L. 2018a. Fin healing and regeneration in sturgeon. J Fish Biol. 93(5):917–930.
- Allen PJ, DeVries RJ, Fox DA, Gabitov RI, Anderson WG. 2018b. Trace element and strontium isotopic analysis of Gulf Sturgeon fin rays to assess habitat use. Environ Biol Fish. 101(3):469–488.
- Allen PJ, Hobbs JA, Cech JJ Jr., Van Eenennaam JP, Doroshov SI. 2009. Using trace elements in pectoral fin rays to assess life history movements in sturgeon: estimating age at initial seawater entry in Klamath River green sturgeon. Trans Am Fish Soc. 138(2):240–250.
- Altenritter ME, Kinnison MT, Zydlewski GB, Secor DH, Zydlewski JD. 2015. Assessing dorsal scute microchemistry of shortnose sturgeon life histories. Environ Biol Fish. 98(12):2321–2335.
- Arai T, Levin AV, Boltunov AN, Miyazaki N. 2002. Migratory history of the Russian sturgeon Acipenser guldenstadti in the Caspian Sea, as revealed by pectoral fin spine Sr:Ca ratios. Marine Biol. 141:315–319.
- Asante KA, Agusa T, Mochizuki H, Ramu K, Inoue S, Kubodera T, Takahashi S, Subramanian A, Tanabe S. 2008. Trace elements and stable isotopes (δ13C and δ15N) in shallow and deep-water organisms from the East China Sea. Environ Pollut. 156(3):862–873. doi:10.1016/j.envpol.2008.05.020
- Balazik MT, Garman GC, Van Eenennaam JP, Mohler J, Curry Woods L III. 2012. Empirical evidence of fall spawning by Atlantic sturgeon in James River, Virginia. Trans Am Fish Soc.141(6):1465–1471.
- Billard R, Lecointre G. 2000. Biology and conservation of sturgeon and paddlefish. Rev Fish Biol Fish. 10(4):355–392.
- Blevins DW. 2011. Water-quality requirements, tolerances, and preferences of Pallid Sturgeons (Scaphirhynchus albus) in the Lower Missouri River. U.S. Geological Survey Scientific Investigations Report. U.S. Geological Survey, Reston, Virginia. 2011-5186, p. 20.
- Cai Y, Shim MJ, Guo L, Shiller A. 2016. Floodplain influence on carbon speciation and fluxes from the lower Pearl River, Mississippi. Geochim Cosmochim Acta. 186:189–206.
- Capo RC, Stewart BW, Chadwick OA. 1998. Strontium isotopes as tracers of ecosystem processes: theory and methods. Geoderma. 82(1–3):197–225. doi:10.1016/s0016-7061(97)00102-x
- Chao X, Jia Y, Wang SSY, Azad Hossain AKM. 2012. Numerical modeling of surface flow and transport phenomena with applications to Lake Pontchartrain. Lake Reservoir Manage. 28(1):31–45.
- Chebanov MS, Savelyeva EA. 1999. New strategies for brood stock management of sturgeon in the sea of Azov basin in response to changes in patterns of spawning migration. J Appl Ichthyol. 15(4–5):183–190.
- Claudet J, Osenberg CW, Domenici P, Badalamenti F, Milazzo M, Falcón JM, Bertocci I, Benedetti-Cecchi L, García-Charton J-A, Goñi R, et al. 2010. Marine reserves: fish life history and ecological traits matter. Ecol Appl. 20(3):830–839.
- Collins MR, Smith TIJ. 1996. Sturgeon spine removal is nondeleterious. N Am J Fish Manage. 16(4):939–941. doi:10.1577/1548-8675(1996)016<0939:SFRRIN>2.3.CO;2
- Cooke SJ, Hinc SG, Farrell AP, Patterso DA, Miller-Saunders K, Welch DW, Donaldson MR, Hanson KC, Crossin GT, Mathes MT, et al. 2008. Developing a mechanistic understanding of fish migrations by linking telemetry with physiology, behavior, genomics and experimental biology: an interdisciplinary case study on adult Fraser River Sockeye Salmon. Fisheries. 33(7):321–339.
- Craft NM, Russell B, Travis S. 2001. Identification of Gulf sturgeon spawning habitats and migratory patterns in the Yellow and Escambia River systems. Final report to the Florida Marine Research Institute, Fish and Wildlife Conservation Commission. Tallahassee (FL): Florida Marine Research Institute, Fish and Wildlife Conservation Commission; p. 32.
- Crocker CE, Cech JJ. 1996. The effects of hypercapnia on the growth of juvenile white sturgeon, Acipenser transmontanus. Aquaculture. 147:293–299. doi:10.1016/s0044-8486(96)01411-1
- Dkhar EN, Dkhar PS, Anal JMH. 2014. Trace elements analysis in drinking water of Meghalaya by using graphite furnace-atomic absorption spectroscopy in relation to environmental health issues. J Chem. 2014:1–8. doi:10.1155/2014/975810
- Duncan MS, Wrege BM, Parauka FM, Isely JJ. 2011. Seasonal distribution of Gulf of Mexico sturgeon in the Pensacola bay system, Florida. J Appl Ichthyol. 27(2):316–321. doi:10.1111/j.1439-0426.2011.01724.x
- Elsdon TS, Gillanders BM. 2003. Reconstructing migratory patterns of fish based on environmental influences on otolith chemistry. Rev Fish Biol Fish. 13(3):217–235. doi:10.1023/B:RFBF.0000033071.73952.40
- Fox DA, Hightower JE, Parauka FM. 2000. Gulf Sturgeon spawning migration and habitat in the Choctawhatchee River system, Alabama-Florida. Trans Am Fish Soc. 129(3):811–826.
- Fox DA, Hightower JE, Parauka FM. 2002. Estuarine and nearshore marine habitat use by Gulf Sturgeon from the Choctawhatchee River system, Florida. Am Fish Soc Sympos. 28:111–126.
- Gilanders BM. 2001. Trace metals in four structures of fish and their use for estimates of stock structures. Fish Bull. 99:410–419.
- Gilbert B, Hussain E, Jirsa F, Avenant-Oldewage A. 2017. Evaluation of trace elements and metal accumulation and edible risk associated with consumption of Labeo umbratus from the Vaal Dam, South Africa. IJERPH. 14(7):678–693.
- Godfrey JD, Bryant DM, Williams MJ. 2003. Radio-telemetry increases free-living energy costs in the endangered Takahe Porphyrio mantelli. Biol Conserv. 11:35–38.
- Graustein WC. 1989. 87Sr/86Sr ratios measure the sources and flow of strontium in terrestrial ecosystems. In: Rundel PW, Ehleringer JR, Nagy KA, editors. Stable isotopes in ecological research. Ecological studies (analysis and synthesis). Vol. 68. New York, NY: Springer; p. 491–512.
- Harris JE, Parkyn DC, Murie DJ. 2005. Distribution of Gulf sturgeon in relation to benthic invertebrate prey resources and environmental parameters in the Suwannee River estuary, Florida. Trans Am Fish Soc. 134(4):975–990.
- Havrylkoff JM, Peterson MS, Slack WT. 2012. Assessment of the seasonal usage of the lower Pascagoula River estuary by Gulf sturgeon (Acipenser oxyrinchus desotoi). J Appl Ichthyol. 28(5):681–686.
- Heinrich PV, McCulloh RP. 1999. Mineral resources map of Louisiana. Baton Rogue, LA: Louisiana Department of Natural Resources, Office of Mineral Resources.
- Heise RJ, Slack WT, Ross ST, Dugo MA. 2005. Gulf sturgeon summer habitat use and fall migration in the Pascagoula River, Mississippi, USA. J Appl Ichthyol. 21(6):461–468.
- Helfman GS, Collette BB, Facey DE. 1997. The diversity of fishes. Boston (MA): Blackwell Science Publishing.
- Hightower JE, Zehfuss KP, Fox DA, Parauka FM. 2002. Summer habitat use by Gulf sturgeon in the Choctawhatchee River, Florida. J Appl Ichthyol. 18(4–6):595–600.
- Hobbs JA, Bennett WA, Burton J, Gras M. 2007. Classification of larval and adult Delta smelt to nursery areas by use of trace elemental fingerprinting. Trans Am Fish Soc. 136(2):518–527.
- Hobbs JA, Lewis LS, Ikemiyagi N, Sommer T, Baxter RD. 2010. The use of otolith strontium isotopes (87Sr/86Sr) to identify nursery habitat for a threatened estuarine fish. Environ Biol Fish. 89(3–4):557–569.
- Hogan JD, Blum MJ, Gilliam JF, Bickford N, McIntyre PB. 2014. Consequences of alternative dispersal strategies in a putatively amphidromous fish. Ecology. 95(9):2397–2408.
- Jobling M. 1995. Environmental biology of fishes. London: Chapman and Hall; p. 455.
- Kennedy BP, Blum JD, Folt CL, Nislow KH. 2000. Using natural strontium isotopic signatures as fish markers: methodology and applications. Can J Fish Aquat Sci. 57(11):2280–2292.
- Kennedy BP, Klaue A, Blum JD, Folt CL, Nislow KH. 2002. Reconstructing the lives of fish using Sr isotopes in otoliths. Can J Fish Aquat Sci. 59(6):925–929.
- Liu K-B, McCloskey TA, Bianchette TA, Keller G, Lam NSN, Cable JE, Arriola J. 2014. Hurricane Isaac storm surge deposition in a coastal wetland along Lake Pontchartrain, southern Louisiana. J Coast Res. 70:266–273.
- Loewen TN, Carriere B, Reist JD, Halden NM, Anderson WG. 2016. Linking physiology and biomineralization processes to ecological inferences on the life history of fishes. Comp Biochem Physiol. 202:123–140. doi:10.1016/j.cbpa.2016.06.017
- McNaught AD, Wilkinson A. 1997. Compendium of chemical terminology: the gold book. 2nd ed. London: Blackwell Science; p. 464.
- Mississippi Department of Environmental Quality. 2007. Citizens guide to water quality in the Pearl River Basin; Jackson, MS: Mississippi Department of Environmental Quality. p. 32.
- Morrow JV Jr., Kirk JP, Killgore KJ, Rogillio HE, Knight C. 1996. Distribution and population attributes of gulf sturgeon in the lower Pearl river system, Louisiana. Proceedings of the Annual Conference Southeastern Association of Fish and Wildlife Agencies; Held in Hot Springs, AR. 5–9 October 1996, 50:79–90. pp. 723.
- Morrow JV Jr., Kirk JP, Killgore KJ, Rogillio HE, Knight C. 1998. Status and recovery potential of Gulf of Mexico sturgeon in the Pearl River system, Louisiana–Mississippi. N Am J Fish Manage. 18(4):798–808. doi:10.1577/1548-8675(1998)018<0798:SARPOG>2.0.CO;2
- Nakano T. 2016. Potential Uses of Stable Isotope Ratios of Sr, Nd, and Pb in Geological Materials for Environmental studies. Proc Jpn Acad, Series B Phy Biol Sci. 92:167–184.
- Nielsen J. 1998. Scientific sampling effects: electrofishing California’s endangered fish populations. Fisheries. 23(12):6–12.
- Phelps QE, Hupfeld RN, Whitledge GW. 2017. Lake sturgeon Acipenser fulvescens and shovelnose sturgeon Scaphirhynchus platorynchus environmental life history revealed using pectoral fin-ray microchemistry: implications for interjurisdictional conservation through fishery closure zones. J Fish Biol. 90(2):626–639. doi:10.1111/jfb.13242
- Phelps QE, Whitledge GW, Tripp SJ, Smith KT, Garvey JE, Herzog DP, Ostendorf DE, Ridings JW, Crites JW, Hrabik RA, et al. 2012. Identifying river of origin for age-0 Scaphirhynchus sturgeons in the Missouri and Mississippi rivers using fin ray microchemistry. Can J Fish Aquat Sci. 69(5):930–941. doi:10.1139/f2012-038
- Pikitch EK, Doukakis P, Lauck L, Chakrabarty P, Erickson DL. 2005. Status, trends, and management of sturgeon and paddlefish fisheries. Fish Fish. 6:233–265. doi:10.1111/j.1467-2979.2005.00190.x
- Pracheil BM, George R, Chakoumakos BC. 2019. Significance of otolith calcium carbonate crystal structure diversity to microchemistry studies. Rev Fish Biol Fish. 29(3):569–588. doi:10.1007/s11160-019-09561-3
- Pracheil BM, Hogan JD, Lyons J, McIntyre PB. 2014. Using hard-part microchemistry to advance conservation and management of North American freshwater fishes. Fish. 39:451–465. doi:10.1080/03632415.2014.937858
- Pracheil BM, Lyons J, Hamann EJ, Short PH, McIntyre PB. 2018. Lifelong population connectivity between large rivers and their tributaries: a case study of shovelnose sturgeon from the Mississippi and Wisconsin rivers. Ecol Freshwater Fish. 28:20–32. doi:10.1111/eff.12423
- Price TD, Burton JH, Bentley RA. 2002. The characterization of biologically available strontium isotope ratios for the study of prehistoric migration. Archaeometry. 44(1):117–135.
- Richir J, Gobert S. 2016. Trace elements in marine environments: occurrence, threats, and monitoring with special focus on the coastal Mediterranean. J Environ Anal Toxicol. 6:349–368.
- Rogillio HE. 1992. Status survey of Gulf sturgeon in Louisiana, Project E1-1. Final Report. Atlanta: Louisiana Department of Wildlife and Fisheries. 6pp.
- Rogillio HE, Rabalais EA, Forester JS, Doolittle CN, Granger WJ, Kirk JP. 2001. Status, movement, and habitat use of Gulf sturgeon in the Lake Pontchartrain basin, Louisiana. Final Report. Baton Rouge: Louisiana Department of Wildlife and Fisheries and National Fish and Wildlife Foundation, Shell Marine Habitat Program.
- Rogillio HE, Ruth RT, Behrens EH, Doolittle CN, Granger WJ, Kirk JP. 2007. Gulf Sturgeon movements in the Pearl River drainage and the Mississippi Sound. N Am J Fish Manag. 27(1):89–95.
- Rorabacher D. 1991. Statistical treatment for rejection of deviant values: critical values of Dixon's Q parameter and related subrange ratios at the 95% confidence level. Anal Chem. 63(2):139–146.
- Secor DH, Henderson-Arzapalo A, Piccoli PM. 1995. Can otolith microchemistry chart patterns of migration and habitat utilization in anadromous fishes? J Exp Marine Biol Ecol. 192(1):15–33.
- Secor DH, Rooker JR. 2000. Is otolith strontium a useful scalar of life cycles in estuarine fishes? Fish Res. 46(1–3):359–371.
- Snead JI, McCulloh RP. 1984. Geologic map of Lousiana. Lousiana Geological Survey.
- Stevenson JT, Secor DH. 2000. Age determination and growth of Hudson River Atlantic sturgeon, Acipenser oxyrinchus. Fish Bull. 98:153–166.
- Sulak KJ, Clugston JP. 1998. Early life history stages of Gulf sturgeon in the Suwannee River, Florida. Trans Am Fish Soc. 127(5):758–771.
- Sulak KJ, Clugston JP. 1999. Recent advances in life history of Gulf of Mexico sturgeon, Acipenser oxyrinchus desotoi, in the Suwannee River, Florida, USA: a synopsis. J of Appl Ichthyol. 15:116–128.
- Sulak KJ, Randall M. 2002. Understanding sturgeon life history: enigmas, myths, and insights from scientific studies. J Appl Ichthyol. 18(4–6):519–528.
- Sulak KJ, Randall MT, Edwards RE, Summers TM, Luke KE, Smith WT, Norem AD, Harden WM, Lukens RH, Parauka F, et al. 2009. Defining winter trophic habitat of juvenile Gulf Sturgeon in the Suwannee and Apalachicola rivermouth estuaries, acoustic telemetry investigations. J Appl Ichthyol. 25(5):505–515.
- Thompson DE. 2009. Economic minerals map of Mississippi. Jackson, MS: Mississippi Department of Environmental Quality.
- Thompson DE. 2011. Geologic map of Mississippi. Jackson, MS: Mississippi Department of Environmental Quality.
- USDA NRCS (US Department of Agriculture, Natural Resources Conservation Service). 2006. Land resource regions and major land resource areas of the United States, the Caribbean, and the Pacific Basin. United States Department of Agriculture Handbook 296. Washington DC: USDA NCRS.
- USFWS (US Fish and Wildlife Service) and GSMFC (Gulf States Marine Fisheries Commission). 1995. Gulf sturgeon recovery–management plan. Atlanta: USFWS.
- Veinott G, Northcote T, Rosenau M, Evans RD. 1999. Concentrations of strontium in the pectoral fin rays of the white sturgeon (Acipenser transmontanus) by laser ablation sampling–inductively coupled plasma mass spectrometry as an indicator of marine migrations. Can J Fish Aquat Sci. 56(11):1981–1990.
- Willmes M, Glessner JJG, Carleton SA, Gerrity PC, Hobbs JA. 2016. 87Sr/86Sr isotope ratio analysis by laser ablation MS-ICP-MS in scales, spines, and fin rays as a non-lethal alternative to otoliths for reconstructing fish life history. Can J Fish Aquat Sci. 73(12):1852–1860.
- Woolnough DA, Downing JA, Newton TJ. 2009. Fish movement and habitat use depends on water body size and shape. Ecol Freshwater Fish. 18(1):83–91.