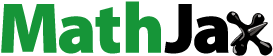
Abstract
Population demographics are used by biologists and managers to determine the quality of a fishery and set regulations to achieve desired management goals. Regulating a large river system fishery that spans a substantial geographical region is often complicated by spatially variable environmental factors that affect fish population dynamics (e.g. habitat, water quality); these dynamics are rarely examined within an entire system. In this study we compared spatial differences in population age and length at capture, growth, fecundity and body condition of bluegill Lepomis macrochirus among two different river reaches (e.g. basin width, gradient, velocity) of the Illinois River (Illinois, USA) and a disconnected floodplain lake, the Emiquon Preserve. Variations in selected population demographics were evaluated in relation to well-documented habitat characteristics (e.g. water quality, aquatic vegetation, backwater availability). Differences in bluegill demographic traits between the disconnected floodplain lake and river reaches were distinct, and variations between river reaches were sex specific. The floodplain lake generally had older bluegill that expressed a higher maximum length at age and a lower growth rate and fecundity than bluegill in river reaches. River bluegill differed between river reaches in male growth, and fecundity in both sexes. Body condition was not significantly different among bluegill populations. Early research on floodplain lakes indicates that this habitat supports greater fish growth and higher reproductive fitness than mainstem habitat. We conclude that increasing the availability of quality backwater habitat, even within a highly modified large river system like the Illinois River, could substantially increase the quality and stability of bluegill populations.
Introduction
Public interest in recreational angling of certain species of sportfish (i.e. fishes that provide recreational value for anglers) can influence their management and alter their ecology. The affinity for certain species varies both culturally and geographically. In North America, bluegill Lepomis macrochirus is one of the most important recreational fishes (Drake et al. Citation1997) and represents an essential component of many freshwater fisheries, given that bluegill is widely distributed and abundant in both lentic and lotic freshwater communities. Because of the recreational and economic value of bluegill, they are well-studied and their subsequent management as a natural resource is well-documented (see Spotte Citation2007). Additionally, with an ubiquitous distribution and highly adaptable life history, bluegill serve as a model organism in fisheries research (e.g. Drake et al. Citation1997; Aday et al. Citation2006; Hoxmeier et al. Citation2009). Assessing the spatial variation in bluegill demographics throughout an entire river system will provide better insight into management of bluegill populations.
Bluegill population demographics have most often been examined in relation to both natural and anthropogenic impacts. For example, researchers have analyzed spatial and temporal variability in environmental factors related to effects of size structure on reproductive investment (Roff Citation1983; Fox and Keast Citation1990; Jennings et al. Citation1997; Aday et al. Citation2002; ), growth and reproductive investment (Drake et al. Citation1997; Garvey et al. Citation2002; Aday et al. Citation2003; Oplinger et al. Citation2011), habitat use and reproduction (Zeug and Winemiller Citation2007), habitat-induced effects on growth and mortality of early life stages (Slipke et al. Citation2005; Rypel et al. Citation2012) and predation (Dewey et al. Citation1997). Research on bluegill populations has primarily focused on lentic habitats, but more-recent research has expanded into lotic systems (e.g. Gutreuter et al. Citation1999; Zeug and Winemiller Citation2007), including large, spatially complex rivers. Large floodplain river systems are comprised of diverse habitat types, including main channel, side channel and connected backwaters or floodplain lakes (Junk et al. Citation1989). Riverine fishes often use these habitats throughout their life cycle and can maximize their growth and fitness by shifting certain population dynamic attributes depending on the habitat type (Werner and Hall Citation1988; Junk et al. Citation1989; Bayley Citation1991). Bluegill occupy all riverine habitat types (e.g. Gutreuter et al. Citation1999; Rypel et al. Citation2012; DeBoer, Thoms et al. Citation2019) with their abundance being linked to backwater habitat and resource availability, seasonal changes and predation (Slipke et al. Citation2005; Zeug and Winemiller Citation2007; Stevens et al. Citation2010; Anderson et al. Citation2017).
Unfortunately, most large rivers and their habitat mosaics are highly modified; 65% of large rivers are fragmented by dams, or affected by hydrological regulation for flood control, hydroelectric generation, irrigation or navigation (Nilsson et al. Citation2005). Dredging, riverbank protection and other engineering activities, which are common in large rivers to control flooding and support commercial navigation, result in significant morphological alterations. These modifications are so severe that main river channels of modified rivers are often isolated from their backwaters and floodplains, thus diminishing the productivity of rivers (Junk et al. Citation1989; Delong Citation2005; Gupta Citation2007). Understanding differences in fish population dynamics along the entire geographical expanse of large rivers can help inform management discussions to help mitigate anthropogenic impacts to fish populations.
The Illinois River (Illinois, USA) is a highly modified river system comprised of a main channel, side channels and connected and disconnected backwater habitat that represents an ideal scenario for comparison of bluegill life history traits across a wide range of habitats. Similar to other heavily modified large rivers (e.g. Mississippi River), the Illinois River is marked by systemic changes that impact the function of the entire river. Currently, the Illinois River is highly modified by both navigation infrastructure and floodplain agriculture that has shifted it to a highly sediment-laden system with greatly reduced backwater habitat quality and quantity (Demissie et al. Citation2016). Additionally, previous research in the Illinois River system has clearly established that fish diversity differs between the upper and lower reaches of the river system (i.e. upper river and lower river) resulting from differences in land use, hydrogeomorphology and water quality (McClelland et al. Citation2012; Gibson-Reinemer et al. Citation2017; DeBoer, Thoms et al. Citation2019). Solomon et al. (Citation2019) also compiled population trends and demographics for selected sportfish species (including bluegill), in the La Grange Pool of the lower Illinois River that showed declining catch rates of large, older fish and stunted age structures – suggesting that environmental stressors in this pool could be contributing to these trends. However, despite clear evidence of spatial differences in both key environmental drivers and resulting fish community composition, there has been no assessment of spatial variation in population dynamics that may explain a portion of these differences. Therefore, the objective of this study was to compare the age and length at capture, growth, reproductive investment and body condition of bluegill among the upper and lower Illinois River and the Emiquon Preserve, a disconnected floodplain lake in the Illinois River system. Due to compounding exposure to anthropogenic influences (e.g. habitat degradation and invasive species) in the lower Illinois River, we predicted (1) bluegill in the upper Illinois River would exhibit a greater maximum length, reproductive fitness and condition than bluegill in the lower Illinois River, (2) bluegill in the lower Illinois River would have a greater growth rate than bluegill in the upper Illinois River and (3) bluegill in the disconnected Emiquon Preserve would have a greater growth rate, maximum length, reproductive fitness and condition than bluegill in the Illinois River as a whole because of fundamental differences between lentic and lotic systems.
Materials and methods
Study area
This study took place within three separate locations of the Illinois River system: upper Illinois River, lower Illinois River and a restored disconnected backwater lake called the Emiquon Preserve (). The Illinois River can be broadly categorized into two distinct regions (upper river and lower river) from its origin to its confluence with the Mississippi River based on geomorphology (Delong Citation2005; McClelland et al. Citation2012). The upper river originates from the confluence of the Des Plaines and Kankakee Rivers and extends downstream to the Great Bend near Hennepin, IL (RKM 334.1) and includes three navigation pools (Dresden Island Pool, Marseilles Pool and Starved Rock Pool) created by lock and dams. The lower river extends from the Great Bend downstream to the confluence of the Illinois and Mississippi Rivers and encompasses three navigation pools (Peoria Pool, La Grange Pool and Alton Pool; McClelland et al. Citation2012). The upper river is characterized by a narrow floodplain, rocky substrates, high gradient, aquatic vegetation and limited backwater habitat (primarily harbors and marinas), whereas the lower river is characterized by a wide floodplain, soft substrates, low gradient, no aquatic vegetation and has more abundant natural, contiguous backwater habitat – compared to the upper river ( Delong Citation2005; McClelland et al. Citation2012; LTRM Citation2016; De Jager and Rohweder Citation2017; DeBoer, Whitten et al. Citation2019; ). For example, in the upper river, the Dresden Island Pool has 2074 hectares of aquatic habitat with a total of 19.1% backwater habitat (15.5% connected and 3.6% disconnected). In contrast, in the lower river, the La Grange Pool has 11,535 hectares of aquatic habitat with a total of 73.9% backwater habitat (49.2% connected and 24.7% disconnected), representing more than five times more backwater area than that of the Dresden Island Pool (; Illinois River land use/cover by pool see LTRM Citation2016). Although the lower river has more backwater habitat area, 50% of the total backwater area is isolated behind agriculture floodplain levees, which are predominantly located in the wide valley of the lower river (Johnson and Hagerty Citation2008). Additionally, backwater habitat quality (i.e. depth, substrate composition and aquatic vegetation) in the lower river is degraded by sedimentation and lack of aquatic vegetation compared to backwater habitat in the upper river (Bhowmik and Demissie Citation1989; Demissie et al. Citation2016; De Jager and Rohweder Citation2017).
Figure 1. Map of the Illinois River watershed (in grey). Navigation pools are separated by locks and dams (L&D), indicated by perpendicular black lines. The upper Illinois River is comprised of pool 3, Dresden Island; pool 4, Marseilles; and pool 5, Starved Rock. The lower Illinois River is comprised of pool 6, Peoria; pool 7, La Grange; and pool 8, Alton. Emiquon is located adjacent to the La Grange Pool of the lower Illinois River ().
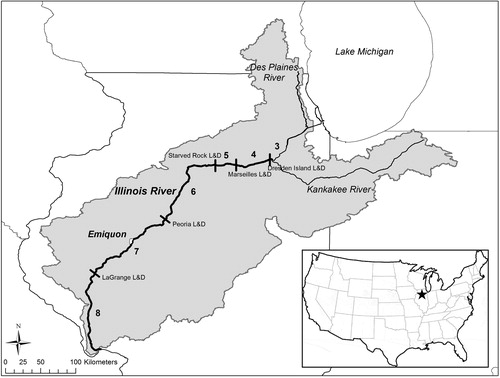
Figure 2. Map of the Illinois River basin (in gray) and main channel, side channels, and connected and disconnected backwaters (in white) of the Dresden Island Pool of the upper Illinois River and the La Grange Pool of the lower Illinois River with the Emiquon Preserve.
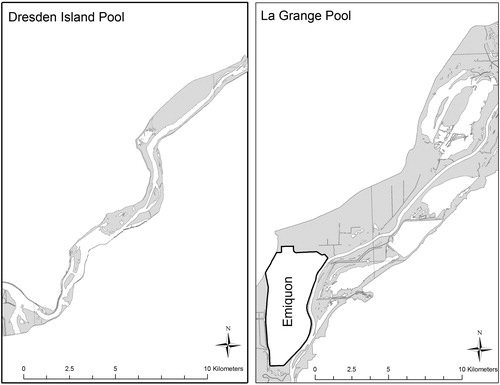
The Nature Conservancy’s Emiquon Preserve (hereafter ‘Emiquon’) is a 2723-ha disconnected floodplain lake complex consisting of two connected lake basins, directly adjacent to the La Grange Pool of the lower Illinois River and extending from RKM 194–202 (Lemke et al. Citation2017) in Fulton County, IL. The Nature Conservancy began aquatic restoration of Emiquon in 2007 (VanMiddlesworth et al. Citation2017), in part to help address the pervasive lack of quality backwater habitat in the lower river. This restoration included numerous activities, including the stocking of a variety of native fishes (VanMiddlesworth et al. Citation2017). After more than a decade of restoration, Emiquon has numerous attributes missing from the rest of the Illinois River: deep water, benthic diversity, a stable hydrograph and abundant aquatic vegetation, which provides high-quality overwintering habitat and subsequently, a productive local fishery (Lemke et al. Citation2017 and references therein). More recently, The Nature Conservancy completed a water management structure in 2016 between Emiquon and the lower Illinois River (RKM 197.6) to manage water levels in Emiquon (Lemke et al. Citation2017). During this study, Emiquon’s water management structure was not operational and Emiquon was not connected to the Illinois River.
Fish collection
Bluegill were collected with the goal of gathering a representative sample of adults over a wide range of sizes from each of the three sample locations in order to evaluate growth, reproductive investment and body condition. Bluegill that were potentially subadults (i.e. roughly < 50 mm and < 1 year) were removed from the analyses if sex was not determined (Solomon et al. Citation2019). Collections were completed during April and May 2015–2016 when water temperatures reached 12–15 °C to ensure gravid fish were sampled in prespawning condition. Bluegill were collected via pulsed direct-current (DC) electrofishing and fyke netting in all three sampling locations (for sampling gear specifications see Ratcliff et al. Citation2014). Targeted sampling was conducted in mainly side channels and backwater areas known to have high abundances of bluegill, such as artificial backwaters (marinas and harbors) in the upper river, natural backwaters in the lower river and remnant, flooded drainage ditches in Emiquon. All fish from the upper and lower river were collected from fully connected habitats; riverine bluegills were not collected from disconnected or semi-connected habitats. Following collection, fish were euthanized, placed on ice and returned to the lab at the Illinois River Biological Station in Havana, Illinois.
Data collection
In the lab, fish were measured for total length (nearest mm), weighed (nearest g), sexed (female and male; immature fish were removed), dissected to obtain gonad weight (nearest g) as an index of reproductive investment and sagittal otoliths were extracted for aging. Whole sagittal otoliths were rinsed in tap water, placed in coin envelopes and allowed to fully dry. After drying, whole otoliths were processed for age estimation by placing them in a black dish filled with tap water and photographing them with a Leica DMC2900 camera mounted on a Leica S8AP0 microscope. Otolith images were then independently aged for age at capture of each fish by two experienced readers to gain a consensus age, with a third reader consulted for any disagreements.
Statistical analyses
The comparability of size distribution of fish collected from each location was assessed by visually examining length–frequency histograms among sample locations (i.e. upper river, lower river and Emiquon). To examine growth, age and length-at-capture data, separate von Bertalanffy growth curves were constructed for female and male bluegill for each location,
where Lt is total length (mm) at age t (years), L∞ is theoretical maximum total length of an individual within the population, K is the growth coefficient (mm year–1) for the population and t0 is the theoretical time when the total length is zero (von Bertalanffy Citation1938; Isely and Grabowski Citation2007). The von Bertalanffy growth function was chosen because of its wide use in fisheries and potential comparability among future studies (Haddon Citation2011). Model parameter estimates L∞ and K and their standard errors were interpreted as the theoretical maximum length and growth rate for population and compared among sampled locations (age one male bluegill were removed from the growth model data because of their small sample size, n = 3 lower Illinois River). Growth models for female and male bluegill were compared among sample locations using the Likelihood Ratio Test to determine if there was a difference in parameters (i.e. L∞, K and t0). If there was a significant difference between the most complex growth model (i.e. all parameters differed among groups) and the simplest model (i.e. no parameters differed among groups) then a family of models was examined in a model-reduction method to determine which model adequately fitted the data (Ogle Citation2016). Once the best-fit model was identified, the model parameters and standard errors were interpreted to determine differences among locations indicated by nonoverlapping standard errors. Regression assumptions of normal distribution and equal variance (homoscedasticity) were checked with the most-complex model for each sex by interpreting residual plots and histograms. Data for both growth models met assumptions and were not transformed.
Reproductive investment of both female and male bluegill among locations was assessed using gonadosomatic index (GSI), expressed as gonad mass as a percentage of total body mass:
An analysis of variance (ANOVA) was performed on both female and male bluegill GSI to determine if they differed among sampling locations. If sampling location was significant, a Tukey Honest Significant Differences post-hoc analysis (Tukey HSD) was performed to identify differences among locations (Bonferroni p-value correction for multiple comparisons).
The overall body condition of bluegill populations among sample locations were assessed by examining mean relative weight (Wr) for both sexes,
where Wi is the measured weight of a fish and Wsi is the standard weight for a fish of a given length (Wege and Anderson Citation1978). To complete this analysis, the standard weight indices for individual fish were first calculated using the accepted bluegill coefficients,
where α is the y-intercept, β is the slope and L is the measured total length of a given fish. The bluegill coefficients used for the standard weight indices were α = –5.376 and β = 3.316 (Hillman Citation1982). All fish within the female and male datasets met the minimum length requirement (80 mm) for standard weight calculations. An ANOVA was performed on the mean relative weight among sampling locations for each female and male bluegill to determine if they differed.
All statistical analyses were performed using R statistical environment (version 3.5.0; R Core Team Citation2018), and growth analyses and relative weight indices were conducted using FSA: Fisheries Stock Analysis Package (Ogle et al. Citation2018). von Bertalanffy growth model start values were generated by using the vbStarts() function for male bluegill, but female bluegill start values were set (L∞ = maximum length in data set, K = 0.3 and t0 = 0) due to a poor starting value error indicated by a ‘singular gradient’ error when the algorithm failed during the model fitting. Additionally, in the among-group comparison, the iterations for the female bluegill growth model of K and t0 were increased from the default 50 to 100 iterations for the model to converge. Growth model parameters were estimated using the nls() function, and growth model reductions were completed with the Likelihood Radio Test using the lrt() function. All ANOVA tests were conducted using the lm() function and assumptions checked using residual plots and Levene’s Tests. Because these analyses were assessing spatial differences, bluegill collected in 2015 and 2016 were combined for all analyses, and an α of 0.05 was used for all analyses unless otherwise specified.
Results
A total of 457 bluegill were collected and processed for analyses (females n = 245, males n = 212; ). The average total length for female fish was 161.1 mm (range = 102–226 mm) in Emiquon, 135.2 mm (range = 99–179 mm) in the upper river and 141.2 mm (range = 91–185 mm) in the lower river (). The average total length for male fish collected was 180.6 mm (range = 120–215 mm) in Emiquon, 157.2 mm (range = 81–193 mm) in the upper river and 153.3 mm (range = 103–195 mm) in the lower river (). The average age of female fish collected by location was 2.95 years (range = 1–5 years) Emiquon, 2.64 years (range = 1–5 years) upper river and 2.27 years (range 1–8 years) lower river. For males, the average ages were 3.12 years (range = 2–5) Emiquon, 2.91 years (range = 2–5 years) upper river and 2.40 years (range = 2–5 years) lower river.
Figure 3. Length–frequency histogram constructed with length interval widths of 10 mm for female bluegill collected in each sample location (i.e. Emiquon, upper Illinois River, lower Illinois River).
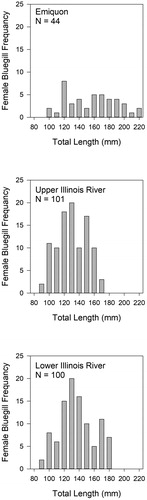
Figure 4. Length–frequency histogram constructed with length interval widths of 10 mm for male bluegill collected in each sample location (i.e. Emiquon, upper Illinois River, lower Illinois River).
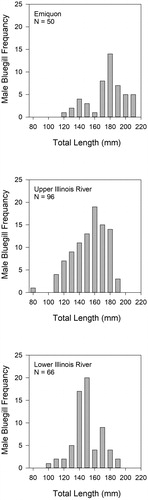
Table 1. Female and male bluegill population sample sizes (N), estimated von Bertalanffy growth curve theoretical maximum total length ± 1 standard error (L∞), growth coefficient ± 1 standard error (K), mean gonadosomatic index ± 1 standard error (GSI) and mean relative weight ± 1 standard error (Wr) for bluegill populations in the three sample locations (Emiquon, upper Illinois River, lower Illinois River).
Growth parameters (i.e. L∞, K, t0) from von Bertalanffy growth models differed between the most-complex and simplest models for both female and male bluegill indicating a significant difference among sampling locations for each sex (female χ2 = 93.625, p < .001; male χ2 = 74.458, p < .001; and ). The model reduction procedure indicated a difference in both L∞ and K parameters for both sexes (females: L∞ χ2 = 29.58, p < .001; K χ2 = 40.07, p < .001; males: L∞ χ2 = 16.47, p < .001; K χ2 = 29.61, p < .001; ). Male bluegill parameter estimates and nonoverlapping standard errors for L∞ indicate that Emiquon had a higher L∞ than the upper river and for K, indicated that the upper river had a higher K than the lower river (). For female bluegill, although the model indicated that L∞ was different among locations, estimates had overlapping standard errors (). This is likely due to L∞ being much greater in Emiquon than both the upper and lower river. Estimates for K and standard errors indicated the lower river had a greater K estimate than Emiquon ().
Figure 5. Female bluegill von Bertalanffy growth coefficient curves by sample location (Emiquon, upper Illinois River, lower Illinois River) within the Illinois River system.
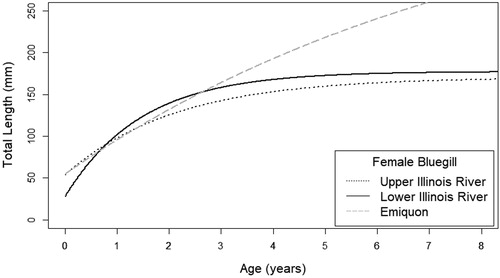
Figure 6. Male bluegill von Bertalanffy growth coefficient curves by sample (Emiquon, upper Illinois River, lower Illinois River) within the Illinois River system.
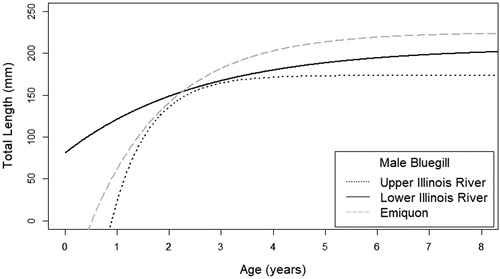
Both sexes had significantly different mean GSI by sample location, although the difference varied by sex (ANOVA p < .001; ). Female bluegill had a greater GSI in the lower river compared to both the upper river and Emiquon, but there was no difference between the upper river and Emiquon (Tukey HSD p < .05; p < .001; p = .3, ). Male bluegill had a significantly greater GSI in the upper river compared to both the lower river and Emiquon but no difference between the lower river and Emiquon (Tukey HSD p < .05; p < .001; p = .64; ). Mean body condition (i.e. Wr) of bluegill populations among sample locations for both sexes were not significantly different (ANOVA females df = 2, F = 2.7, p = .07; males df = 2, F = 2.5, p = .09; ).
Discussion
We observed marked differences in multiple bluegill population demographic parameters among the upper river, lower river and Emiquon; however, these spatial differences were largely sex dependent. Male bluegill varied in their growth rates, and both sexes differed in their reproductive investment between the upper and lower river, while maximum length and body condition at age did not differ among sampled locations. Bluegill in Emiquon were generally older fish with a higher maximum length and slower growth rate and lower reproductive investment. According to Pianka (Citation1970), this outcome suggests that the Emiquon bluegill population represents a stable, high density, competitive environment relative to the river. While these results support our predictions of spatial variation in population dynamics, they also show that bluegill are negatively impacted in the lower river, presumably due to the long history of highly modified geomorphological and anthropogenic environmental conditions.
The observed differences in Illinois River male bluegill growth and both male and female bluegill reproductive investment are likely a reflection of many factors; however, limited resource availability (i.e. food) can negatively impact bluegill (of both sexes) growth and reproductive investment. Differential resource availability, especially in food production and foraging habitat from backwaters, between the upper river and the resource-limited lower river could explain some of the observed variation. Male bluegill in the upper river have a significantly greater population growth and reproductive investment compared to the lower river, although neither of these seem to lead to significant differences in maximum length at age. In contrast, female bluegill in the lower river had a significantly greater reproductive investment compared to the upper river. For both sexes, bluegill collected from the upper river were older and male bluegill were larger (on average) than those from the lower river. Limited resources, and the fish’s perception of future limited resource availability, can reduce growth and reproductive investment and produce variable body size within a population (Diana Citation1987; Aday et al. Citation2006). Furthermore, restricted resource availability can have a stronger impact on reproductive investment in females than in males due to females’ relatively high energetic demand for gonad production (Whiteman Citation1997). For example, high resource availability in experimental ponds produced significantly longer and heavier juvenile bluegill of both sexes (Aday et al. Citation2006), and low resource availability decreased gonad maturation and reproductive output, but had no impact on the length and weight of immature or mature female bluegill (Fox and Crivelli Citation1998; Aday et al. Citation2006).
Extrapolating from experimental systems to in situ situations can be difficult, but in the case of the Illinois River, we have multiple lines of evidence for significant upper to lower river differences in both productivity and availability of key bluegill habitats and environmental conditions to make this assertion. First, the upper river has lower turbidity and abundant aquatic vegetation (VonBank et al. Citation2016) that could provide additional food resources (e.g. zooplankton and other macroinvertebrates, etc.) for multiple life-stages of fish compared to the turbid, unvegetated lower river (De Jager and Rohweder Citation2017; DeBoer, Whitten et al. Citation2019). Second, the lower river also has one of the largest invasive silver carp Hypophthalmichthys molitrix populations in the world, which has negatively impacted the plankton community (Sass et al. Citation2010; DeBoer et al. Citation2018). Comparatively, the upper river has a considerably lower silver carp density due to intensive removal efforts by contracted commercial fishers (e.g. Love et al. Citation2018) and a population that is mainly comprised of lower-river migrants (ACRCC Citation2020). Moreover, bigheaded carp (i.e. collectively silver carp and bighead carp H. nobilis) planktivory has been associated with the decline in abundance and condition of formerly dominant native planktivores in the Illinois River, such as gizzard shad Dorosoma cepedianum and bigmouth buffalo Ictiobus cyprinellus (Irons et al. Citation2007; Pendleton et al. Citation2017; Fritts et al. Citation2018), and is coincident with a change in the overall fish community of the La Grange Pool of the lower river (Solomon et al. Citation2016). Additionally, Emiquon has received low bluegill fishing pressure (15% of anglers 2015 and 31% of anglers 2016) compared to other sportfish such as largemouth bass Micropterus salmoides and crappie species Pomoxis spp., which could be impacting their growth and development (Low Citation2015; Hoff and Jugovic Citation2016). In comparison, the Illinois River is not known as a bluegill fishery but supports fishing tournaments for black basses Micropterus spp., sauger Sander canadensis and walleye S. vitreus in the upper river, and catfish tournaments (e.g. channel catfish Ictallurus punctatus, blue catfish I. furcatus flathead catfish Pylodictis olivaris) in the lower river (Illinois River 2020 Tournaments Citation2020). Ultimately, differences in bigheaded carp abundance, turbidity and aquatic vegetation between the upper and lower river may be causing variation in food availability that potentially explains our findings of lower growth rates and reproductive investment in male bluegill, but does not exclusively explain the greater growth and reproductive investment for female bluegill in the lower river.
Social interaction between large adult and juvenile male bluegill is known to influence reproductive investment by delaying maturation in juvenile male bluegill (Gross Citation1982; Jennings et al. Citation1997; Aday et al. Citation2003). Similarly, we found male bluegill from the upper river were larger and older on average compared to those from the lower river, which suggests social interactions between adult and juvenile bluegill could be another factor disproportionally influencing growth and reproductive investment. These interactions were not measured in this study. Long-term monitoring data supports a difference in bluegill abundance and size between the river reaches by showing that bluegill abundance (based on CPUE) on average is greater in the upper river, while individuals are (based on PSD) slightly larger on average than in the lower river (main- and side-channel habitat; DeBoer, Whitten et al. Citation2019). As a result, the size of mature males in the upper river could be influencing the maturation timeline and, ultimately, individual fitness.
Large floodplain rivers are naturally dynamic environments that functionally rely on a seasonal flood pulse to increase lateral connectivity to productive floodplain habitats for spawning and nursery areas for riverine fishes (Junk et al. Citation1989; Scott and Nielsen Citation1989; Bayley Citation1991). The disruption of this primary driver of fish productivity by anthropogenic factors (e.g. levees and altered flood pulse) affects the life history of fishes (Junk et al. Citation1989; Sparks Citation1995). Our data showed that female bluegill in the lower river, where some semblance of a natural flood pulse remains, have a greater reproductive investment than both the upper river and Emiquon, where the flood pulse is highly modified. Previous studies investigating centrarchid reproductive investment in spatially variable environments showed that early maturation, reproduction and a greater investment in reproduction is common (Fox and Keast Citation1991; Fox Citation1994). In the Illinois River, both the upper and lower river are impacted by altered hydrology with the flood pulse becoming more frequent, greater in magnitude and more often occurring outside of the historically normal season (Sparks et al. Citation1998; Milly et al. Citation2002; Kelly et al. Citation2017). Additionally, the lower river has well-documented sedimentation issues that have reduced bathymetric diversity, reduced the capacity of many off-channel areas to hold water, and caused the loss (and predicted, potential future loss) of many off-channel areas (Bellrose et al. Citation1983; Bhowmik and Demissie Citation1989; USGS 1999). In contrast, the upper river has relatively high quality off-channel habitats (active or abandoned marinas and harbors), although they are much more spatially limited than natural backwaters in the lower river (Chara-Serna et al. Citation2019). High sedimentation rates in the lower Illinois River increase environmental variability, decreasing backwater habitat quality by reducing dissolved oxygen levels and water temperatures during the winter, which negatively impact fish overwintering (i.e. surviving a winter season) in backwater habitat (e.g. Sheehan et al.Citation1990; Raibley et al. Citation1997; Theiling et al. Citation2014). Furthermore, our data indicate that female bluegill collected in the lower river were slightly larger and younger on average than those from the upper river, although the calculated theoretical maximum length did not differ. This is consistent with Solomon et al. (Citation2019) findings in the La Grange Pool of the lower river that identified a reduced age range for several sportfish species that use backwaters including bluegill. This pattern suggests that increased environmental variability (e.g. sedimentation, water quality, backwater overwintering habitat) is likely a major driver of differences in bluegill population demographics.
Assessing trends in sportfish population demographics between large rivers could provide information on the response of large rivers to management practices; although a potentially optimal comparison, these types of analysis can be problematic. By their nature, most comparable large rivers are found in biogeographically different regions, so measuring species-specific responses of important sportfish like bluegill to spatial variation in geomorphology can be confounded by other factors like latitude, climate and ecoregion (Counihan et al. Citation2018). Future river-to-river analysis must account for differences in geomorphology and anthropogenic modifications that typify the different rivers, as these features can drive differences in abiotic (e.g. river temperature, depth and discharge) and biotic (e.g. vegetation abundance, fish species composition and fish diversity) factors. The Illinois River is a tributary to the Upper Mississippi River, another large, floodplain river, which could serve as a comparable river system. Studies of bluegill population demographics within the Upper Mississippi River and lower Illinois River do exist, but none of these studies use directly comparable population demographic metrics (see Gutreuter et al. Citation1999; Smith et al. Citation2007; Solomon et al. Citation2016, Citation2019). Relatively few published studies exist on highly modified, large river bluegill population growth, reproductive investment and condition (e.g. Zeug and Winemiller Citation2007) among habitat types compared to publications on demographics in smaller lotic systems (e.g. Putman et al. Citation1995; Sammons and Maceina Citation2009), with the majority of studies conducted in lentic systems. Thus, to help evaluate differences in population demographics of popular sportfish species, additional system-wide studies need to be completed in large rivers to assess the health of fish communities and strengthen management practices.
Ultimately, this study identified spatial differences in bluegill population demographics that can be supported by differences in habitat availability and quality rather than random variability, although further investigation is needed. Specifically, we observed the presence of younger, rapidly growing and fecund female bluegill in the lower river suggesting a potential negative result of habitat availability and quality. To the best of our knowledge, this is the only study comparing bluegill demographics within the Illinois River system, but a more robust bluegill sample size could elucidate more distinctions among bluegill population demographics. Additionally, in order to fully understand what is driving differences between bluegill sexes within the Illinois River, which could be representative of highly modified large rivers in general, further work should include abiotic and biotic factors in their analysis of species-specific population dynamics to help solidify our understanding of spatial variations within these systems to better informing large river management and restoration. In particular, research is needed on how habitat improvements directly affect local portions of large river systems, such as reduction of sedimentation accumulation and wave energy in connected backwaters, an increase of vegetated wetland-type habitat (e.g. Sparks et al. Citation1990; Burdis et al. Citation2020), and reconnecting disconnected backwaters (Lemke et al. Citation2018). These habitat improvements could create pockets of refuge for riverine fishes, and ultimately, enhance fishery production in areas that display unsatisfactory undesirable population demographics.
Acknowledgements
The authors thank past and present biologists and technicians at the Illinois Natural History Survey’s Illinois River Biological Station for their assistance in data collection and processing. Fish care and use protocols were followed in accordance with the University of Illinois Institutional Animal Care and Use Committee (17018). The findings and conclusions in this article are those of the authors and do not necessarily represent the views of the U.S. Fish and Wildlife Service.
Disclosure statement
No potential conflict of interest was reported by the author(s).
Additional information
Funding
Notes on contributors
Andrya L. Whitten
Andrya L. Whitten is a large river fisheries ecologist for the Illinois Natural History Survey at the Illinois River Biological Station, Havana, Illinois USA. She primarily works on monitoring long-term trends in fish populations on the Illinois and Mississippi rivers.
Jason A. DeBoer
Jason A. DeBoer is a large-river fisheries ecologist with the Illinois River Biological Station – Illinois Natural History Survey, Havana, Illinois USA. His research focuses on monitoring long-term trends in fish populations in the Illinois and Mississippi rivers, and understanding the interactive effects of anthropogenic stressors and geomorphology on large river ecosystems.
Levi E. Solomon
Levi E. Solomon is an associate scientist and large river ecologist with the Illinois Natural History Survey's Illinois River Biological Station, Havana, Illinois USA. His research focuses on fish communities on the Illinois River, specifically focusing on long-term trends and population dynamics of sport and commercial fish species as well as impacts of invasive species to the existing riverine fish community.
Andrea K. Fritts
Andrea K. Fritts is a biologist with the U.S. Geological Survey, La Crosse, Wisconsin USA. Her research focuses on understanding the effects that invasive carps may have on native aquatic species. She also uses acoustic telemetry to study invasive carp behavior near navigation lock and dams to inform the design and testing of deterrent technologies.
Mark W. Fritts
Mark W. Fritts is a fish biologist with the US Fish and Wildlife Service, Onalaska, Wisconsin USA. His current research focuses on the behavior of invasive fishes in the Upper Mississippi River. Mark's previous research efforts included work on fish communities in large rivers, the role of endocrine disrupting compounds in fish reproduction, endangered sturgeon and mussel conservation, and the early life-history of sharks.
Richard M. Pendleton
Richard M. Pendleton is an aquatic ecologist at Cornell University in cooperation with the New York State Department of Environmental Conservation, New Paltz, New York USA. His research focuses on large river freshwater ecology and diadromous, endangered, and invasive species.
T. D. VanMiddlesworth
T. D. VanMiddlesworth is a district 2 assistant fisheries biologist for the North Carolina Wildlife Resource Commission Division of Inland Fisheries Management, Greenville, North Carolina USA. His work primarily focuses on management of anadromous fish including American Shad Alosa sapidissima, Blueback River Herring Alosa aesfivalis, and Striped Bass Morone saxatilis. He also focuses on management of sportfish such as Largemouth Bass Micropterus salmoides, Bluegill Lepomis macrochirus, Black Crappie Pomoxis nigromaculatus, Redear Sunfish Lepomis microlophus, Pumpkinseed Lepomis gibbosus, and catfish spp. as well as invasive species such as common carp Cyprinus carpio.
Andrew F. Casper
Andrew F. Casper is an aquatic ecologist and research affiliate at the Illinois Natural History Survey's Illinois River Biological Station, Havana, Illinois USA. His recent research themes have focused on the historical patterns and trends in river biota based on analysis of multi-decadal data sets and experiments from large rivers across North America.
References
- Aday DD, Kush CM, Wahl DH, Philipp DP. 2002. The influence of stunted body size on the reproductive ecology of bluegill Lepomis macrochirus. Ecol Freshwater Fish. 11(3):190–195.
- Aday DD, Philipp DP, Wahl DH. 2006. Sex-specific life history patterns in bluegill (Lepomis macrochirus): interacting mechanisms influence individual body size. Oecologia. 147(1):31–38.
- Aday DD, Wahl DH, Philipp DP. 2003. Assessing population-specific and environmental influences on bluegill life histories: a common garden approach. Ecology. 84(12):3370–3375.
- Anderson AM, Casper AF, McCain KNS. 2017. Fish indicators of ecosystem health: upper Mississippi River system. Urbana‐Champaign (IL): Illinois Natural History Survey, University of Illinois Urbana‐Champaign. Report No.: 2017_16.
- Asian Carp Regional Coordinating Committee (ACRCC). 2020. Asian Carp action plan for fiscal year 2020. Washington (DC): Council on Environmental Quality.
- Bayley PB. 1991. The flood pulse advantage and the restoration of river-floodplain systems. Regul Rivers Res Mgmt. 6(2):75–86.
- Bellrose FC, Havera SP, Paveglio FL Jr, Steffeck DW. 1983. The fate of lakes in the Illinois River valley. INHS Bull. 32(1–4):1–54.
- Bhowmik NG, Demissie M. 1989. Sedimentation in the Illinois River valley and backwater lakes. J Hydrol. 105(1–2):187–195.
- Burdis RM, DeLain SA, Lund EM, Moore MJC, Popp WA. 2020. Decadal trends and ecological shifts in backwater lakes of a large floodplain river: Upper Mississippi River. Aquat Sci. 82(2):27.
- Chara-Serna AM, Maxson KA, Anderson AM, Zalay BD, Casper AF. 2019. Zooplankton as an indicator of the ecosystem response to bigheaded carp suppression via commercial harvest in the Illinois River (2010–2017). Report prepared for the Springfield, IL: Illinois Department of Natural Resources, Division of Fisheries. Illinois Natural History Survey Report 2018(37).
- Counihan TD, Waite IR, Casper AF, Ward DL, Sauer JS, Irwin ER, Chapman CG, Ickes BS, Paukert CP, Kosovich JJ, et al. 2018. Can data from disparate long-term fish monitoring programs be used to increase our understanding of regional and continental trends in large river assemblages? PLoS One 13(1):e0191472..
- DeBoer JA, Anderson AM, Casper AF. 2018. Multi-trophic response to invasive silver carp (Hypophthalmichthys molitrix) in a large floodplain river. Freshw Biol. 63(6):597–611.
- DeBoer JA, Thoms MC, Casper AF, Delong MD. 2019. The response of fish diversity in a highly modified large river system to multiple anthropogenic stressors. J Geophys Res Biogeosci. 124(2):384–404.
- DeBoer JA, Whitten AL, Lubinski BJ, Chick JH. 2019. Long-term survey and assessment of large-river fishes in Illinois, 2018 (Project) F-101-R. Annual report prepared for the Illinois Department of Natural Resources, Division of Fisheries and the U. S. Fish and Wildlife Service. Illinois Natural History Survey Technical Report 2019 (15).
- De Jager NR, Rohweder JJ. 2017. Changes in aquatic vegetation and floodplain land cover in the Upper Mississippi and Illinois rivers (1989–2000–2010). Environ Monit Assess. 189(77).
- Delong MD. 2005. Upper Mississippi River basin. In: Benke AC, Cushing CE, editors. Rivers of North America. Burlington (MA): Elsevier Academic Press. p. 327–374.
- Demissie M, Getahun E, Keefer L. 2016. The sediment budget of the Illinois River: 1981–2015. Champaign (IL): Illinois State Water Survey Report of Investigation 122.
- Dewey MR, Richardson WB, Zigler SJ. 1997. Patterns of foraging and distribution of bluegill sunfish in a Mississippi River backwater: influence of macrophytes and predation. Ecol Freshwater Fish. 6(1):8–15.
- Diana JS. 1987. Simulation of mechanisms causing stunting in northern pike populations. Trans Am Fish Soc. 116(4):612–617.
- Drake MT, Claussen JE, Philipp DP, Pereira DL. 1997. A comparison of bluegill reproductive strategies and growth among lakes with different fishing intensities. North Am J Fish Manage. 17(2):496–507.
- Fox MG. 1994. Growth, density and interspecific influences on pumpkinseed life histories. Ecology 75(4):1157–1171.
- Fox MG, Crivelli AJ. 1998. Body size and reproductive allocation in a multiple spawning centrarchid. Can J Fish Aquat Sci. 55(3):737–748.
- Fox MG, Keast A. 1990. Effects of winterkill on population structure, body size, and prey consumption patterns of pumpkinseed in isolated beaver ponds. Can J Zool. 68(12):2489–2498.
- Fox MG, Keast A. 1991. Effects of overwinter mortality on reproductive life history characteristics of pumpkinseed (Lepomis gibbosus) populations. Can J Fish Aquat Sci. 48(9):1792–1799.
- Fritts AK, Knights BC, Lafrancois TD, Vallazza JM, Bartsch LA, Bartsch MR, Richardson WB, Bailey SW, Kreiling RM, Karns BN. 2018. Evaluating potential effects of bigheaded carps on fatty acid profiles of multiple trophic levels in large rivers of the Midwest, USA. Food Webs. 16:e00095.
- Garvey JE, Herra TP, Leggett WC. 2002. Protracted reproduction in sunfish: the temporal dimension in fish recruitment revisited. Ecol Appl. 12(1):194–205.2.0.CO;2]
- Gibson-Reinemer DK, Sparks RE, Parker JL, Deboer JA, Fritts MW, McClelland MA, Chick JH, Casper AF. 2017. Ecological recovery of a fiver fish assemblage following the implementation of the clean water act. Bioscience. 67(11):957–970.
- Gross MR. 1982. Sneakers, satellites, and parental: polymorphic mating strategies in North American sunfishes. Z Tierpschol. 60(1):1–26.
- Gupta A. 2007. Large rivers: geomorphology and management. Chichester (UK): Wiley.
- Gutreuter S, Bartels AD, Irons K, Sandheinrich MB. 1999. Evaluation of the flood-pulse concept based on statistical models of growth and selected fishes of the Upper Mississippi River system. Can J Fish Aquat Sci. 56(12):2282–2291.
- Haddon MJ. 2011. Modelling and quantitative methods in fisheries. 2nd ed. Boca Raton (FL): Chapman & Hall/CRC.
- Hillman WP. 1982. Structure and dynamics of unique bluegill populations [master’s thesis]. Columbia (MO): University of Missouri.
- Hoff M, Jugovic M. 2016. Emiquon preserve visitor use survey and creel survey report 2016. Annual report prepared for The Nature Conservancy: Emiquon Preserve.
- Hoxmeier RJH, Aday DD, Wahl DH. 2009. Examining interpopulation variation in bluegill growth rates and size structure: effects of harvest, maturation, and environmental variables. Trans Am Fish Soc. 138(2):423–432.
- Illinois River 2020 Tournaments. 2020. Illinois Department of Natural Resources. [accessed 2020 Jul 12]. https://www.ifishillinois.org/tournament/display_tournament.php?watersID=3615.
- Irons KS, Sass GG, McClelland MA, Stafford JD. 2007. Reduced condition factor of two native fish species coincident with invasion of non-native Asian carps in the Illinois River, U.S.A. Is this evidence for competition and reduced fitness? J Fish Biol. 71(suppl D):258–273.
- Isely JJ, Grabowski TB. 2007. Age and growth. In: Guy CS, Brown ML, editors. Analysis and interpretation of freshwater fisheries data. Bethesda (MD): American Fisheries Society. p. 187–228.
- Jennings MJ, Claussen JE, Philipp DP. 1997. Effect of population size structure on reproductive investment of male bluegill. North Am J Fish Manage. 17(2):516–524.
- Johnson BL, Hagerty KH, editors. 2008. Status and trends of selected resources of the Upper Mississippi River System. La Crosse (WI): U.S. Geological Survey, Upper Midwest Environmental Sciences Center. Technical Report: LTRMP 2008-T002. 102 pp + Appendixes A–B.
- Junk WJ, Bayley PB, Sparks RE. 1989. The flood pulse concept in river-floodplain systems. In: Dodge DP, editor. Proceedings of the International Large River Symposium (LARS). Canadian Special Publication of Fisheries and Aquatic Sciences. Vol. 106. p. 110–127.
- Kelly SA, Takbiri Z, Belmont P, Foufoula-Georgiou E. 2017. Human amplified changes in precipitation-runoff patterns in large river basins of Midwestern United States. Hydrol Earth Syst Sci. 21(10):5065–5088.
- Lemke MJ, Casper AF, Hagy HM, Chen H. 2018. Floodplain wetland restoration along the Illinois River. Chapter 4. In: Lenhart C, Smiley PC, editors. Ecological restoration in the Midwest: past, present, and future. Iowa City (IA): University of Iowa Press. p. 79–106.
- Lemke MJ, Walk JW, Lemke AM, Sparks RE, Blodgett KD. 2017. Introduction: the ecology of a river floodplain and the Emiquon preserve. Hydrobiologia 804(1):1–17.
- Long Term Resource Monitoring (LTRM). 2016. Upper Mississippi River System – systemic spatial data viewer, Upper Midwest Environmental Sciences Center, La Crosse (WI). [accessed 2020 Jun 12]. https://umesc.usgs.gov/management/dss/umrs_land_cover_viewer.html.
- Love SA, Lederman NJ, Anderson RL, DeBoer JA, Casper AF. 2018. Does aquatic invasive species removal benefit native fish? The response of gizzard shad (Dorosoma cepedianum) to commercial harvest of bighead carp (Hypophthalmichthys nobilis) and silver carp (H. molitrix). Hydrobiologia 817(1):403–412.
- Low L. 2015. Visitor use survey report 2015. Annual report prepared for The Nature Conservancy: Emiquon Preserve.
- McClelland MA, Sass GG, Cook TR, Irons KS, Michaels NN, O’Hara TM, Smith CS. 2012. The long-term Illinois River fish population monitoring program. Fisheries 37(8):340–350.
- Milly PCD, Wetherald RT, Dunne KA, Delworth TL. 2002. Increasing risk of great floods in a changing climate. Nature 415(6871):514–517.
- Nilsson C, Reidy CA, Dynesius M, Revenga C. 2005. Fragmentation and flow regulation of the world's large river systems. Science 308(5720):405–408.
- Ogle D. 2016. Introductory fisheries analyses with R. New York (NY): Chapman and Hall/CRC.
- Ogle DH, Wheeler P, Dinno A. 2018. FSA: Fisheries Stock Analysis. R package version 0.8.22. [accessed 2019 Jan 15]. https://github.com/droglenc/FSA.
- Oplinger RW, Wahl DH, Nannini MA. 2011. Effects of predators on growth and reproductive investment in bluegills: comparison of populations with differing size and age of maturation. Trans Am Fish Soc. 140(6):1532–1539.
- Pendleton RM, Schwinghamer C, Solomon LE, Casper AF. 2017. Competition among river planktivores: are native planktivores still fewer and skinnier in response to the Silver Carp invasion? Environ Biol Fish. 100(10):1213–1222.
- Pianka ER. 1970. On r- and K-selection. Am Nat. 104(940):592–597.
- Putman JH, Pierce CL, Day DM. 1995. Relationships between environmental variables and size-specific growth rates of Illinois stream fishes. Trans Am Fish Soc. 124(2):252–261.
- R Core Team. 2018. R: a language and environment for statistical computing. Vienna (Austria): R Foundation for Statistical Computing. https://www.R-project.org/.
- Raibley PT, Irons KS, O’Hara TM, Blodgett KD, Sparks RE. 1997. Winter habitats used by largemouth bass in the Illinois River, a large river-floodplain ecosystem. North Am J Fish Manage. 17(2):401–412.
- Ratcliff EN, Glittinger EJ, O’Hara TM, Ickes BS. 2014. Long term resource monitoring program procedures: fish monitoring. Reston (VA): U.S. Geological Survey.
- Roff DA. 1983. An allocation model of growth and reproduction in fish. Can J Fish Aquat Sci. 40(9):1395–1404.
- Rypel AL, Pounds KM, Findlay RH. 2012. Spatial and temporal trade-offs by bluegills in floodplain river ecosystems. Ecosystems 15(4):555–563.
- Sammons SM, Maceina MJ. 2009. Variation in growth and survival of bluegill and redbreast sunfish in Georgia rivers. North Am J Fish Manage. 29(1):101–108.
- Sass GG, Cook TR, Irons KS, McClelland MA, Michaels NN, O’Hara TM, Stroub MR. 2010. A mark-recapture population estimate for invasive silver carp (Hypophthalmichthys moxlitrix) in the La Grange Reach, Illinois River. Biol Invasions. 12(3):433–436.
- Scott MT, Nielsen LA. 1989. Young fish distribution in backwaters and main-channel borders of the Kanawha River, West Virginia. J Fish Biol. 35:21–27.
- Sheehan R, Lewis WM, Bodensteiner L. 1990. Winter habitat requirements and overwintering of riverine fishes (I). Springfield (IL): Illinois Department of Conservation. Federal Aid in Sport Fish Restoration Project F-79-R, Final Reports.
- Slipke JW, Sammons SM, Maceina MJ. 2005. Importance of the connectivity of backwater areas for fish production in Demopolis Reservoir, Alabama. J Freshwater Ecol. 20(3):479–485.
- Smith MA, Pegg MA, Irons KS. 2007. Analysis of fish age structure and growth in the Illinois River. La Crosse, Wisconsin: U.S. Geological Survey, Upper Midwest Environmental Sciences Center. LTRMP Technical Report 2007–T002. 55 p.
- Solomon LE, Pendleton RM, Chick JH, Casper AF. 2016. Long-term changes in fish community structure in relation to the establishment of Asian carps in a large floodplain river. Biol Invasions. 18(10):2883–2895.
- Solomon LE, Pendleton RM, Maxson KA, McQuaid JN, Gibson-Reinemer DK, Anderson CA, Anderson RL, Lampo EG, Lamer JT, Casper AF. 2019. Status, trends, and population demographics of selected sportfish species in the La Grange Reach of the Illinois River. INHS Bull. 42:2019002.
- Sparks RE. 1995. Need for ecosystem management of large rivers and their floodplains. BioScience 45(3):168–182.
- Sparks RE, Bayley PB, Kohler SL, Osborne LL. 1990. Disturbance and recovery of large floodplain River. Environ Manage. 14(5):699–709.
- Sparks RE, Nelson JC, Yin Y. 1998. Naturalization of the flood regime in regulated rivers. BioScience 48(9):706–720.
- Spotte S. 2007. Bluegills: biology and behavior. Bethesda (MD): American Fisheries Society.
- Stevens PW, Greenwood MFD, Idelberger CF, Blewett DA. 2010. Mainstem and backwater fish assemblages in the tidal Caloosahatchee River: implications for freshwater inflow studies. Estuaries Coast. 33(5):1216–1224.
- Theiling CH, Janvrin JA, Hendrickson J. 2014. Upper Mississippi River restoration: implementation, monitoring, and learning since 1986. Restor Ecol. 23(2):157–166.
- United States Geological Survey (USGS). 1999. Ecological status and trends of the Upper River System 1998: a report of the Long Term Resource Monitoring Program. La Crosse, Wisconsin: U.S. Geological Survey, Upper Midwest Environmental Sciences Center. LTRMP 99-T001. 236 p.
- VanMiddlesworth TD, McClelland NN, Sass GG, Casper AF, Spier TW, Lemke MJ. 2017. Fish community succession and biomanipulation to control two common aquatic ecosystem stressors during a large-scale floodplain lake restoration. Hydrobiologia. 804(1):73–88.
- von Bertalanffy L. 1938. A quantitative theory of organic growth. Hum Biol. 10:181–213.
- VonBank JA, Hagy HM, Casper AF. 2016. Energetic carrying capacity of riverine and connected wetlands on the upper Illinois River for fall-migrating waterfowl. Am Midl Nat. 176(2):210–221.
- Wege GW, Anderson RO. 1978. Relative weight (Wr): a new index of condition for Largemouth Bass. In: Novinger GD, Dillard JG, editors. New approaches to the management of small impoundments. Vol. 5 of Special Publication. Bethesda (MD): American Fisheries Society. p. 79–91.
- Werner EE, Hall DJ. 1988. Otogenetic habitat shifts in bluegill: the foraging rate-predation risk trade-off. Ecology. 69(5):1352–1366.
- Whiteman HH. 1997. Maintenance of polymorphism promoted by sex-specific fitness payoffs. Evolution. 51(6):2039–2044.
- Zeug SC, Winemiller KO. 2007. Ecological correlates of fish reproductive activity in floodplain rivers: a life-history based approach. Can J Fish Aquat Sci. 64:1291–1301.