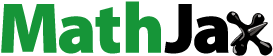
Abstract
Diatoms are a type of photosynthetic phytoplankton that can acclimate to the light level of their environment. Diatoms from different habitats exhibit different photoacclimation characteristics; those from relatively low-light intensity habitats present relatively high photosynthetic activity, high light-harvesting pigment (Chla) content, and low xanthophyll content. Conversely, diatoms from high-light intensity environments have lower photosynthetic activity, less Chla content, and efficient xanthophyll cycling. Hence, diatoms can maintain high growth and photosynthetic activity within a wide range of light intensities. Whether the mechanisms of such adaptability are a consequence of biological photoacclimation to different light intensities or interspecific differences is unknown. Here, we show that differences in photoacclimation ability are more significant than species differences. We found similar species compositions in low- and high-light habitats of the Hanjiang River, China; however, there were remarkable differences in rapid light-response curve parameters. The photoacclimation of algae and trends in their photosynthetic activity can be estimated by rapid detection techniques. We isolated three diatom species common to both habitats and grew them under various light intensities, finding that they have an excellent ability to acclimate to local light conditions. Diatoms can use physiological strategies to handle light fluctuations, but only for short periods. Our results provide a theoretical basis for controlling algal blooms through light management. With knowledge of the photoacclimation characteristics of diatoms and according to the availability of local water conservancy facilities, it is possible to use light management to control diatom blooms more efficiently than with conventional techniques, thereby reducing water usage.
Introduction
Diatom blooms break out frequently worldwide, leading to serious environmental problems and drinking water crises in some regions (Hijnen et al. Citation2007). The Hanjiang River is the largest tributary of the Yangtze River in China. Diatom blooms have occurred 11 times in the Hanjiang River since 1992 (Table S1, supplementary material). The middle route of the South-to-North Water Transfer Project (MRP) diverts 9.5 billion m3 of water annually from the Danjiangkou reservoir (located in the middle and upper reaches of the Hanjiang River) to Beijing Tuancheng Lake. This reduces the flow in the middle and lower reaches of the Hanjiang River. To remedy this, some 3.2 billion m3 of water is diverted annually from the Yangtze River (which has a high sediment concentration) to the Hanjiang River at Xinglong. The probability of diatom blooms has increased by 40.2% since operation of the MRP began. One proposed approach of diatom-bloom control is to increase water discharges from upstream reservoirs (Yang et al. Citation2012). However, during a diatom bloom in the Hanjiang River in 2018, the water flow was as high as 900 m3/s (Xin et al. Citation2019). This means that in order to prevent and control diatom blooms in this river, greater water flow is needed, which will inevitably aggravate the trade-off between storing water in the dry season and maintaining high flows.
Diatoms are considered to be well adapted to light. Diatom can develop distinct adaptive strategies to acclimate to the light environment of their habitats. Usually, diatoms living in relatively low-light habitats, such as turbid estuaries, shaded stream segments and high-latitude lakes, have relatively high photosynthetic activity and amounts of light-harvesting pigment (chlorophyll a, Chla) but low xanthophyll contents (Rodriguez et al. Citation2012). Conversely, diatoms living in habitats with relatively high-light intensities, such as shallow water, stream segments with abundant sunlight and low-latitude lakes, have relatively low photosynthetic activity, low Chla contents and an efficient xanthophyll cycle (Gameiro et al. Citation2011; Huisman et al. Citation2004; Polimene et al. Citation2014). When exposed to light conditions opposite to that of their original habitat, diatoms exhibit lower photosynthetic efficiency and decreased growth rates (Shi et al. Citation2016). Previous research has shown that diatoms from different habitats with distinct light intensities presented different photoacclimation. However, in these studies, the species collected from low-light habitats have been different to those collected from high-light habitats. Hence, it is difficult to determine whether the differences in photoacclimation are mainly due to interspecific differences or to acclimation to the environment. Additionally, most research related to photoacclimation in diatoms has been conducted via laboratory experiments after the algae has been isolated and purified. However, laboratory experiments take a long time, such that the appropriate time for controlling a diatom bloom may be missed. Therefore, a rapid detection method is needed.
Physiological regulation is the main photoprotective response of non-motile species to light stress (Barnett et al. Citation2015). Diatoms can use the xanthophyll cycle mechanism, which is usually expressed as nonphotochemical quenching (NPQ) to relieve high-light stress (Dimier et al. Citation2009), and increase the Chla concentration per cell to withstand low-light stress (Brunet et al. Citation2011). Diatoms of high-light habitat can increase the surface area of the chloroplast and increase the Chla concentration after just a few hours of low-light exposure (Laviale et al. Citation2009; Migné et al. Citation2004). Diatoms of low-light habitat show higher NPQ after a few minutes of high-light exposure (KaShino et al. Citation2002). However, it remains unclear how long such physiological regulation response to continuing light stress based on diurnal change can persist among different populations of diatoms of the same species that live in different habitats.
This study aimed to explore three hypotheses. First, the photoacclimation (high-light acclimated or low-light acclimated) of algae can be determined by rapid detection. Second, differences in the photoacclimation of diatoms are due to biological acclimation to different light levels rather than to interspecific differences. Third, the physiological regulation of diatoms under continuing light stress cannot be maintained for long periods. To test these three hypotheses, we isolated the same diatom species from both high- and low-light habitats and compared their growth rates and photosynthetic activities under five light intensities (5–320 μmol photons m−2 s−1). Confirmation of these hypotheses would suggest that it is possible to use lower amounts of water to control diatom blooms efficiently by using light management at locations with appropriate water management infrastructure. Suitable infrastructure includes the Yangtze River to Han River Water Transfer Project, which could be used to control high-light-acclimated algae, or the water conservancy infrastructure in the mainstream of the Hanjiang River, which could be used to control low-light-acclimated algae.
Materials and methods
Sampling and analysis
The algae samples used in the main experiment in 2017 were collected from similar locations to those used for a preliminary experiment in 2016. Sampling was carried out at three pairs of sites in Hanjiang River from Zhongxiang to Xiantao in March 2017. We defined two habitats at each pair of sites as low-light habitat (LLH, light intensity at 0.5 m depth < 50 μmol photons m−2 s−1) and high-light habitat (HLH, light intensity at 0.5 m depth > 300 μmol photons m−2 s−1). The habitat pairs were about 1–5 km apart from each other and had similar physical and chemical characteristics but different light intensities. Remarkable differences in diatom rapid light-response curve (RLC) parameters but similar species compositions (Table S2, supplementary material) were found in each habitat pair. Parameters of the rapid light-response curves (RLCs) of three pairs of samples collected from LLHs and HLHs were measured initially in the field and after 5 hours of low-light (LL, 5 μmol photons m−2 s−1) and high-light (HL, 320 μmol photons m−2 s−1) treatment.
Chlorophyll a fluorescence parameters
Photosynthetic performance was measured by using a pulse amplitude-modulated fluorescence monitoring system (Phyto-PAM, Walz, Effeltrich, Germany).
The effective photochemical efficiency
The effective quantum yield of PS II (△F/F′m), considered as effective photochemical efficiency, was measured using the quenching method (Schreiber et al. Citation1995). Values of △F/F′m were caculated by utilising EquationEq. (1)(1)
(1) .
(1)
(1)
where F′m was the maximum fluorescence after saturating light pulses, Fs was the fluorescence at actinic light.
Rapid light-response curve (RLC) parameters
RLC was used to demonstrate the relative change of photosynthetic performance under increasing light intensities (Ihnken et al. Citation2010). Each sample was exposed to 12 increasing actinic light from 3 to 1480 μmol photons m−2 s−1 for 10 s of every light level (PAR), photosynthetic parameters was obtained by fitting the model of Platt et al (Citation1981) with the least-squares method. Photosynthetic parameters included photon-capturing efficiency (α), light saturation point (Ik) and maximum electron transport rate (rETRm).
(2)
(2)
The rETRm represented maximum relative electron transport rate of the RLC and attributes to changes in Calvin cycle enzymes (RUBISCO) activity and concentration (Behrenfeld et al. Citation2004). The parameter α was the initial slope of RLC which reflects the photon-capturing efficiency under limit light conditions (Genty et al. Citation1989).
(3)
(3)
The light saturation point of photosynthesis (Ik) indicates the PAR at which photosynthesis is saturated.
Isolation and culture of diatom species
For further confirmation of the photoacclimation characteristics of algae, we isolated a total of six diatom strains (three species × two habitats) from the Hanjiang River at Shayang (LLH2 and HLH2). These culture strains were maintained two weeks in the laboratory after isolation until the experiment. It lasted about three months from the isolation of diatoms to the end of the experiment. The physicochemical indexes of the two habitats are shown in . The three diatom species isolated were Stephanodiscus hantzschii Grunow, Melosira granulate (Ehr.) Ralfs and Synedra ulna Kützing (). We isolated them according to standard phycological methods (Stein and Hellebust Citation1979) and then made a monoculture from them in D1 medium (Zhang and Huang Citation1991). All the cultures were transferred weekly to fresh D1 medium to avoid nutrient depletion.
Table 1. Physicochemical index of the two habitats in Hanjiang River at Shayang.
Table 2. Information of diatoms used in this study.
Growth experiment
Growth experiment of six strains diatoms under different light intensities were conducted in illuminated incubators with a 14/10 light/dark cycle (20 °C day, 18 °C night). The light intensity was set at 5, 20, 80, 160 and 320 μmol photons m−2 s−1 with a cool-white fluorescent light. Each light treatment was repeated with triplicate cultures to minimise errors. We placed each of the cultures (200 ml with an initial algal concentration of about 2.0 × 106 cells L−1) in a 250 mL flask. Samples were harvested every second day to estimate the cell density and photosynthetic parameters over the 16-day experiment.
Growth rate
Diatom samples preserved with 1% Lugol's iodine solution were counted using counting chambers under an Olympus CX31 light microscope. The specific growth rate of each strain was calculated based on EquationEq. (4)(4)
(4) :
(4)
(4)
where μ is the specific growth rate (d−1), Bt2 is the cell density on the last day of the logarithmic growth phase; Bt1 is the cell density on the first day of the logarithmic growth phase.
Chlorophyll a concentration
Chla concentration was determined from light absorbance at 665, 645, and 630 nm according to EquationEq. (5)(5)
(5) (Stein and Hellebust Citation1979) with a spectrophotometer (Shimadzu UV2550, Japan).
(5)
(5)
Chla concentration were expressed as pg Chla per microgram of carbon (pg Chla μg C−1). The carbon content of each strain of diatom was estimated by biovolume according to Warnaars and Hondzo (Citation2006): log10C = 0.758 log10V − 0.422, where V is the cell volume in cubic micrometres and C is cell carbon in micrograms.
Xanthophyll cycle pigment content
The diatom suspension was gently filtered through a Whatman GF⁄F glass fibre filter and stored at −80 °C until pigment extraction. The filters were ground and left overnight in darkness at 4 °C in glass tubes containing 5 mL of methanol. The extracts were centrifuged and the supernatants filtered with 0.45 μm nylon membranes. Sample volumes of 50 μL were injected into a Ultra Performance Liquid Chromatography (UPLC) system (Waters Acquity H-Class, Waters, USA) with fluorescent detector (λex = 431 nm, λem = 660 nm) and an Acquity UPLC BEH C18 column (2.1 × 100 mm, 1.7 μm, Waters). Pigments were quantified and analysed according to the methods used by Lohr and Wilhelm (Citation2001). The amounts of the photoprotective pigments diadinoxanthin (Ddx) and diatoxanthin (Dtx) were measured on days 1, 3, 7 and 13.
Non-photochemical quenching
Non-photochemical quenching (NPQ) was usually used to determined excess heat dissipation in algae (Lavaud et al. Citation2004). NPQ was calculated by using EquationEq. (6)(6)
(6) (KaShino et al. Citation2002).
(6)
(6)
where F′m is the maximum fluorescence yield of light-adapted sample, and Fm is the maximum fluorescence yield following dark adaptation of 5 min.
Statistical analysis
Differences in the growth rate, Chla concentration, Dtx concentration, RLC parameters, △F/F′m and NPQ values of the algal collected from different habitats were compared using one-way ANOVA followed by Tukey's post-hoc tests. ANOVA was performed using SPSS 18.0. For all statistical analyses, significance was set at P < 0.05. The data are presented as mean ± standard deviation. Correlation analysis was used to quantify relationships between the growth rate on day 7 and light intensity.
Results
Rapid light-response curve (RLC) parameters of algae samples
The RLC parameters (α, rETRm, Ik) of algal samples from different habitats were compared at initial collection (0 h) and after 5 h of LL or HL treatment (, ). At the initial stage, the α values of algae samples from the LLH were significantly higher than those from the HLH (P < 0.05). The rETRm and Ik values of algal samples from the LLH were significantly lower than those from the HLH (P < 0.05). After 5 hours HL treatment, the α values decreased, while the Ik and rETRm values increased significantly in LLH algal samples (P < 0.05) (–(c)). After 5 hours LL treatment, the α values increased, while the Ik and rETRm values decreased significantly in algal samples from the HLH (P < 0.05) (–(f)).
Figure 1. Rapid light-response curve of algal samples from different habitats treated with low- and high-light intensities. LL: Low-light intensity (5 μmolphotons m−2 s−1); HL: High-light intensity (320 μmolphotons m−2 s−1). Data are medians ± standard deviations, n = 3.
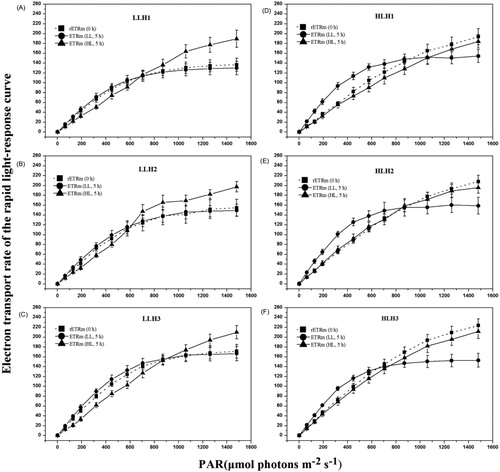
Table 3. Photosynthetic parameters of algal samples from different habitats treated with low- and high-light intensities.
Growth of diatoms
Obvious differences in growth rates were found in LLH and HLH diatoms in the laboratory experiments (). When cultured in LL, the cell density of LLH diatoms and HLH diatoms both grew slowly (). LLH diatoms underwent a growth acceleration at 20 μmol photons m−2 s−1, leading to a much higher density than that of HLH diatoms (P < 0.05) (). The growth advantage of HLH diatoms over LLH diatoms was reversed at the light intensity of 80 μmol photons m−2 s−1 (). The growth rates of HLH diatoms were 0.6 times (P < 0.05) and 1.3 times (P < 0.01) higher than those of LLH diatoms at light intensities of 160 and 320 μmol photons m−2 s−1, respectively (). In the present study, light intensity is positively correlated to the growth rate of HLH diatoms (r = 0.67, P < 0.05) but negatively to the growth rate of LLH diatoms (r = −0.59, P < 0.05).
Effective quantum yield of PS II (△F/F′m)
In general, the △F/F′m values of LLH diatoms decreased with increasing light intensities, while those of HLH diatoms got maximum value at relative high-light intensities. The maximum △F/F′m value (0.592 ± 0.002) of S. hantzschii(LLH) was observed at 5 μmol photons m−2 s−1 on day 7 (). The maximum △F/F′m value (0.612 ± 0.026) of S. hantzschii(HLH) was found at 160 μmol photons m−2 s−1 on day 9 (). M. granulata(LLH) had higher △F/F′m values at 20 and 80 μmol photons m−2 s−1, while in the 320 μmol photons m−2 s−1 treatment they decreased dramatically from day 7(). The △F/F′m values of M. granulata(HLH) reached a maximum at 160 μmol photons m−2 s−1 (). The △F/F′m values of S.ulna(LLH) reached a maximum of 0.608 ± 0.015 at 20 μmol photons m−2 s−1 on day 7 (). The △F/F′m values of S.ulna(HLH) presented an obvious decrease at 5 μmol photons m−2 s−1 (). In the present study, light intensity is positively correlated to △F/F′m values of HLH diatoms (r = 0.530, P < 0.05) but negatively to△F/F′m values of LLH diatoms (r = −0.694, P < 0.05).
Chlorophyll a concentration
For LLH diatoms, no significant differences in Chla concentration were found between LL and HL treatments (). In contrast, the Chla concentration of HLH diatoms presented an obvious fluctuation when exposed to the low-light environment, with a rapid initial increase followed by a gradual decrease (). A significant increase in Chla concentration in the LL treatment (ChlaLL) on day 3 was found in S. hantzschii(HLH) (P < 0.01), followed by S. ulna(HLH) and M. granulata(HLH) (P < 0.05). The ChlaLL of three strains of HLH diatoms showed obvious increases under LL from days 3 to 11 but only a slight increase under HL.
Xanthophyll cycle pigment content
These different preconditions resulted in significant differences in the Dtx contents of the cultures. HLH diatoms contained high amounts of Dtx at the onset of the measurements, whereas LLH diatoms contained almost no Dtx (). The Dtx concentrations of LLH and HLH diatoms were significantly higher on day 5 and day 7 (P < 0.05). However, three cultures of LLH diatoms showed significant decreases in Dtx on day 13 (P < 0.05).
Figure 6. Variations in diatom NPQ and diatoxanthin concentration. Lines shows the variations in the NPQ values of LLH and HLH diatoms under LL or HL treatment. Columns show the concentrations of diatoxanthin in six strains of diatoms under HL on days 1, 3, 7 and 13. Values are the median ± standard deviation of triplicate measurements. HL: 320 μmol photons m−2 s−1. (A) LLH diatoms; (B) HLH diatoms.
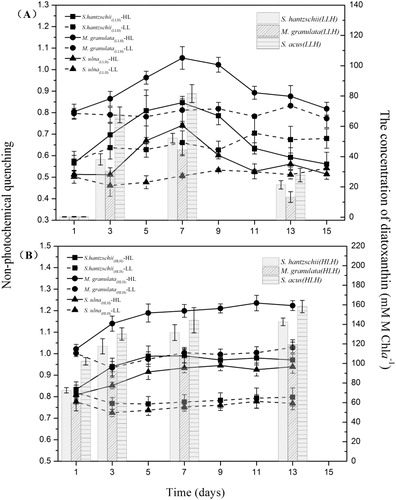
Non-photochemical quenching
The NPQ of LLH diatoms presented obvious increases under HL from day 3 followed by a gradual decrease from day 9, while that under LL showed a minor fluctuation around the initial value (). The NPQ of HLH diatoms exhibited a distinct pattern of variation in contrast with LLH diatoms (). The NPQ values under HL were much higher than those under LL in HLH diatoms during the whole 16-day experiment. No obvious fluctuations were found in the NPQ of HLH diatoms under the LL treatments.
Discussion
Rapid detection of photoacclimation of algal samples
High α and low rETRmax and Ik values are considered common features of low-light-acclimated species, while low α and high rETRmax and Ik values are common features of high-light-acclimated species (Dimier et al. Citation2009). In the present study, the field measurements of high α and low rETRmax and Ik values in algal samples from LLHs are indicative of their low-light acclimation to their original habitat. Low α and high rETRmax and Ik values show the immediate physiological regulations that occur for HL acclimation after 5 hours’ HL treatment. Meanwhile, no obvious fluctuations in RLC parameters were found after 5 hours’ LL treatment of algal samples from LLHs, further indicating that they were low-light-acclimated algae and would maintain high photosynthetic activity at relatively low-light intensities. In the same way, algae from HLHs were high-light acclimated and would maintain high photosynthetic activity at relatively high-light intensities. Low-light intensity will make the RLC parameters of algae from HLHs exhibit the opposite trend. The photoacclimation of algae can be estimated through this type of simple monitoring over short periods. LLH and HLH phytoplankton communities with similar species compositions showed large differences in RLC parameters, suggesting that the light history plays a greater role in photoacclimation than species type. Laboratory experiments further confirmed this inference.
Growth and photosynthetic characteristics of HLH and LLH diatoms
In this study, the higher effective quantum yield (△F/F’m) values of LLH diatoms presented at relatively low-light intensities. The △F/F’m values of HLH diatoms increased with light intensity. The △F/F’m values were closely related to the photosynthetic rate (mg C [mg Chla]−1h−1 and assimilation rate of CO2 (Hartig et al., Citation1998), which can reflect the growth rate of algae (Edwards and Baker, Citation1993). The growth rates showed similar trends as the △F/F’m values. The same diatom species from different habitats showed distinct optimum range of light intensity, which indicated habitat-specific patterns.
Previous studies support our results from another point of view. Summarising the results of previous surveys (e.g., Yao et al. Citation2012; Luan et al. Citation2010), it is clear that diatoms dominate the phytoplankton composition of the main rivers of China, and that the dominant species in each water system are similar (). By analysing the distributions of several common dominant species of diatoms around the world, it can be seen that the same species not only thrive in environments with extremely low-light intensities, they also dominate environments with extremely high-light intensities. Strains of F. crotonensis isolated from a low-light habitat in Lake Erie acclimated well to fairly low irradiances in the laboratory (Hartig and Wallen Citation1986) and maintained a high growth rate at 27.6 μmol photons m−2 s−1 (Rhee and Gotham Citation1981). Meanwhile, F. crotonensis also became the dominant species in Lake Aydat with a light intensity of 150 μmol photons m−2 s−1 (Dauta et al. Citation1990). The optimum light intensity for S. acus was 63.5 μmol photons m−2 s−1 in the River Spree, Germany (Köhler Citation1994), but was in the range of 200–300 μmol photons m−2 s−1 in Lake Melangsee (Schmitt and Nixdorf Citation1999). These results suggested that the optimum light intensity for diatom growth is mainly determined by the historical light environment from another point of view.
Table 4. Diatom densities in the main rivers of China.
Physiological regulation
Diatoms can regulate their Chla concentration and chloroplast area when exposed to different light intensities which, in turn, affects their light utilisation (Laviale et al. Citation2009). In the present study, the obvious increase in the Chla concentration HLH diatoms indicates an active response to LL. The regulation of Chla may slow down the decrease in the growth rates of HLH diatoms in LL. The obvious decrease of Chla concentration of HLH diatoms in the later stage of the experiment suggested Chla regulation could help diatoms respond to LL stress in the short period.
NPQ is dependent on the xanthophyll cycle and can help dissipate excess energy through thermal radiation (Lavaud et al. Citation2004). In the present study, a steady-state NPQ component was observed during the earlier stages of high-light illumination, which was dependent on the diatoxanthin formed by the light-activated diadinoxanthin cycle. This NPQ component corresponded to the Dtx-dependent NPQ that was described by several authors as being the main photoprotective mechanism in diatoms (Lavaud et al. Citation2002; Goss et al. Citation2006). There were high Dtx concentrations and NPQ values in HLH diatoms under HL, suggesting that high-light-acclimated algae species have a higher pool of xanthophyll-cycling pigments per cell than low-light-acclimated species (Dimier et al. Citation2009). An increase in NPQ values and decrease in α under HL was found for LLH algae. Increased energy dissipation and decreased photon-capturing efficiency acted as the principal forms of photoinhibition regulation in LLH algae. Physiological regulation may have helped LLH diatoms maintain a certain growth rate in the middle stage of the experiment. At scales of hours to days, there was a coherence in photoprotective pigment (Ddx and Dtx) responses to changing light levels (KaShino et al. Citation2002). However, the obvious decrease of Dtx concentrations of LLH diatoms at later stages suggested this would not be efficient for physiological regulation when diatoms face stable and continued light stress. The relatively low growth rates of LLH diatoms under HL compared to the other four light intensities may be due to a large amount of energy dissipation being dependent on the xanthophyll cycle (Moline Citation1998). The NPQ was consistent with the Dtx concentration and peaked near day 7 but decreased obviously on day 13. The decrease in NPQ after day 7 does not necessarily indicate that there is a process by which LLH diatoms became adapted to intense light with lesser expression of NPQ. We speculate that intense light causes severe physiological damage that lowers NPQ expression. The decrease in the △F/F′m values of LLH diatoms under HL in the later period indicates low photosynthetic activity. In a previous study, the content of D1 protein increased in the recovery stage after a short period of light stress, but it was still lower than that before stress (Han Citation2016). Unlike the short-term adaptation system, photosystem II does not easily reverse its dark response after several days to weeks of light stress, and the speed of D1 protein recombination is not as fast as that of PSII damage (Ensminger et al., Citation2006). The results of the present study confirm our hypotheses that diatoms have excellent photoacclimation capabilities and use physiological strategies to handle short-term light fluctuations.
In general, the locations at which algae were sampled were similar in 2016 and 2017. This indicates that there was steady photoacclimation of algae to their local habitat. We predicted that the diatoms would undergo steady light acclimation to their local habitat and that their resting spores would be well preserved. In other studies, Prochlorococcus can be divided into low-light-acclimated and high-light-acclimated types according to light adaptability. There are obvious differences in pigment composition and gene sequences between these two ecotypes (Johnson et al., Citation2006). The sequences of phycoerythrin and phycobiliprotein in Prochlorococcus and Synechococcus change greatly to adapt to light (Ting et al., Citation2002). Diatoms with different ecotypes can acclimate low-light and high-light in local habitat steadily. The changes in pigment composition and gene sequences between diatom ecotypes require further research.
In conclusion, the diatoms displayed excellent and steady acclimation to local light conditions and the differences in photoacclimation were more significant between habitat light levels than between species. The photoacclimation of algae and trends in their photosynthetic activity can be estimated by rapid detection (Chlorophyll a fluorescence parameters). Diatoms can use physiological strategies to handle light fluctuations, but only for short periods. Furthermore, controlling light intensity can play an important role in reducing phytoplankton activity of phosphorus uptake and photosynthesis simultaneously because the optimum light intensity required for the growth of diatom is consistent with the required light intensity of high affinity for phosphorus absorption (Shi et al. Citation2015). This provides a theoretical basis for controlling algal blooms through light management. According to the photoacclimation characteristics of diatoms and local water conservancy facilities, it is possible to use less water to control diatom blooms efficiently by using light management.
Supplemental Material
Download MS Word (17.6 KB)Acknowledgements
The authors thank Dr. Qiang Yang (Trinity College, Dublin) for their valuable comments. The authors thank Dr. Lingling Zheng (FACHB, Institute of Hydrobiology, Chinese Academy of Sciences, Wuhan) for offering technical support in diatom isolation.
Disclosure statement
No potential conflict of interest was reported by the author(s).
Additional information
Funding
Notes on contributors
Pengling Shi
Dr. Pengling Shi is a teacher at Hunan University of Arts and Science, China. She studies the relationship between light and diatoms in rivers.
Ping Xie
Dr. Ping Xie is a researcher at Institute of Hydrobiology, Chinese Academy of Sciences, China. He is a wetland ecologist who studies the influence of disturbance on freshwater ecosystems.
Lanhai Liu
Dr. Jingzhen Cui and Lanhai Liu are teachers at Hunan University of Arts and Science, China. They studies the distribution and photosynthetic activity of phytoplankton in southern rivers.
Jingzhen Cui
Dr. Jingzhen Cui and Lanhai Liu are teachers at Hunan University of Arts and Science, China. They studies the distribution and photosynthetic activity of phytoplankton in southern rivers.
Xiaoqing Wang
Dr. Pinhong Yang is a professor at Hunan University of Arts and Science, China, where he has taught in the disciplines of ecology. He works in freshwater ecology.
Pinhong Yang
Dr. Xiaoqing Wang is a professor at Hunan Agricultural University, China, where he has taught in the disciplines of hydrobiology, and worked with the responses of phytoplankton physiology to the light stress.
References
- Barnett A, Méléder V, Blommaert L, Lepetit B, Gaudin P, Vyverman W, Sabbe K, Dupuy C, Lavaud J. 2015. Growth form defines physiological photoprotective capacity in intertidal benthic diatoms. Isme J. 9(1):32–14.
- Behrenfeld MJ, Prasil O, Babin M, Bruyant F. 2004. In search of a physiological basis for covariations in light-limited and light-saturated photosynthesis. J Phycol. 40(1):4–25.
- Brunet C, Johnsen G, Lavaud J, Roy S. 2011. Pigments and photoacclimation processes. In Roy S, Johnsen G, Llewellyn C, Skarstad E, editors. Phytoplankton pigments, characterization, chemotaxonomy and application in oceanography, Cambridge University Press, Cambridge, p. 445–454.
- Dauta A, Devaux J, Piquemal F, Boumnich L. 1990. Growth rate of four freshwater algae in relation to light and temperature. Hydrobiologia. 207(1):221–226.
- Dimier C, Giovanni S, Ferdinando T, Brunet C. 2009. Comparative ecophysiology of the xanthophyll cycle in six marine phytoplanktonic species. Protist. 160(3):397–411.
- Edwards GE, Baker NR. 1993. Can CO2 assimilation in maize leaves be predicted accurately from chlorophyll fluorescence analysis? Photosynth Res. 37(2):89–102.
- Ensminger I, Busch F, Huner NPA. 2006. Photostasis and cold acclimation: sensing low temperature through photosynthesis. Physiol Plant. 126(1):28–44.
- Gameiro C, Zwolinski J, Brotas V. 2011. Light control on phytoplankton production in a shallow and turbid estuarine system. Hydrobiologia. 669(1):249–263.
- Genty B, Briantais JM, Baker NR. 1989. The relationship between the quantum yield of photosynthetic electron transport and quenching of chlorophyll fluorescence. Biochim Biophys Acta Gen Subj. 990(1):87–92.
- Goss R, Pinto EA, Wilhelm C, Richter M. 2006. The importance of a highly active and ΔpH-regulated diatoxanthin epoxidase for the regulation of the PS II antenna function in diadinoxanthin cycle containing algae. J Plant Physiol. 163(10):1008–1021.
- Han Y. 2016. Regulation of non-photochemical quenching in photosynthetica acclimation of two xerophytic shrubs to fluctuating environments (In Chinese). Doctor, Beijing Forestry University.
- Hartig P, Wolfstein K, Lippemeier S, Colijn F. 1998. Photosynthetic activity of natural microphytobenthos populations measured by fluorescence (PAM) and C-14-tracer methods: a comparison. Mar Ecol Prog Ser. 166:53–62.
- Hartig JH, Wallen DG. 1986. The influence of light and temperature on growth and photosynthesis of Fragilaria crotonensis Kitton. J Freshw Ecol. 3(3):371–382.
- Hijnen WA, Dullemont YJ, Schijven JF, Hanzens-Brouwer AJ, Rosielle M, Medema G. 2007. Removal and fate of Cryptosporidium parvum, Clostridium perfringens and small-sized centric diatoms (Stephanodiscus hantzschii) in slow sand filters. Water Res. 41(10):2151–2162.
- Huisman J, Sharples J, Stroom JM, Visser PM, Kardinaal WEA, Verspagen JMH, Sommeijer B. 2004. Changes in turbulent mixing shift competition for light between phytoplankton species. Ecology. 85(11):2960–2970.
- Ihnken S, Eggert A, Beardall J. 2010. Exposure times in rapid light curves affect photosynthetic parameters in algae. Aquat Bot. 93(3):185–194.
- Johnson ZI, Zinser ER, Coe A, McNulty NP, Woodward EMS, Chisholm SW. 2006. Niche partitioning among Prochlorococcus ecotypes along ocean-scale environmental gradients. Science. 311(5768):1737–1740.
- Köhler J. 1994. Origin and succession of phytoplankton in a river-lake system (Spree, Germany). Hydrobiologia. 289:73–83.
- Kashino Y, Kudoh S, Hayashi Y, Suzuki Y, Odate T, Hirawake T, Satoh K, Fukuchi M. 2002. Strategies of phytoplankton to perform effective photosynthesis in the North Water. Deep Sea Res Part Ii. 49(22-23):5049–5061.
- Lavaud J, Rousseau B, Etienne AL. 2002. In diatoms, a transthylakoid proton gradient alone is not sufficient to induce a non-photochemical fluorescence quenching. FEBS Lett. 523(1-3):163–166.
- Lavaud J, Rousseau B, Etienne AL. 2004. General features of photoprotection by energy dissipation in planktonic diatoms (Bacillariophyceae). J Phycol. 40(1):130–137.
- Laviale M, Prygiel J, Lemoine Y, Courseaux A, Creach A. 2009. Stream periphyton photoacclimation response in field conditions: effect of community development and seasonal CHANGES(1). J Phycol. 45(5):1072–1082.
- Lohr M, Wilhelm C. 2001. Xanthophyll synthesis in diatoms: quantification of putative intermediates and comparison of pigment conversion kinetics with rate constants derived from a model. Planta. 212(3):382–391.
- Luan Z, Fan Y, Men X. 2010. Environmental quality evaluation of Songhua River in Harbin using diatom assemblages (In Chinese). J Lake Sci. 22:86–92.
- Migné A, Spilmont N, Davoult D. 2004. In situ measurements of benthic primary production during emersion: seasonal variations and annual production in the Bay of Somme (eastern English Channel, France). Cont Shelf Res. 24(13/14):1437–1449.
- Moline MA. 1998. Photoadaptive Response During the Development of a Coastal Antarctic Diatom Bloom and Relationship to Water Column Stability. Limnol Oceanogr. 43(1):146–153.
- Platt T, Gallegos C, Harrison W. 1981. Photoinhibition of photosynthesis in natural assemblages of marine phytoplankton. J Mar Res. 38:687–701.
- Polimene L, Brunet C, Butenschön M, Martinez-Vicente V, Widdicombe C, Torres R, Allen JI. 2014. Modelling a light-driven phytoplankton succession. J Plankton Res. 36(1):214–229.
- Rhee GY, Gotham IJ. 1981. The effect of environmental factors on phytoplankton growth: light and the interactions of light with nitrate limitation. Limnol Oceanogr. 26:649–659.
- Rodriguez P, Solange Vera M, Pizarro H. 2012. Primary production of phytoplankton and periphyton in two humic lakes of a South American wetland. Limnology. 13(3):281–287.
- Schmitt M, Nixdorf B. 1999. Spring phytoplankton dynamics in a shallow eutrophic lake. Hydrobiologia. 143:269–276.
- Schreiber U, Bilger W, Neubauer C. 1995. Chlorophyll fluorescence as a nonintrusive indicator for rapid assessment of in vivo photosynthesis. Berlin: Springer Berlin Heidelberg.
- Shi P, Shen H, Wang W, Chen W, Xie P. 2015. The relationship between light intensity and nutrient uptake kinetics in six freshwater diatoms. J Environ Sci – China. 34:28–36.
- Shi P, Shen H, Wang W, Yang Q, Xie P. 2016. Habitat-specific differences in adaptation to light in freshwater diatoms. J Appl Phycol. 28(1):227–213.
- Stein JR, Hellebust JA. 1979. Culture methods and growth measurements. Cambridge: Cambridge University Press.
- Ting CS, Rocap G, King J, Chisholm SW. 2002. Cyanobacterial photosynthesis in the oceans: the origins and significance of divergent light-harvesting strategies. Trends Microbiol. 10(3):134–142.
- Warnaars T, Hondzo M. 2006. Small‐scale fluid motion mediates growth and nutrient uptake of Selenastrum capricornutum. Freshwater Biol. 51(6):999–1015.
- Xin X, Wang Y, Hu S, Li J. 2019. Cause analysis of Diatom Bloom of Lower Reaches of Hanjiang River in 2018 (In Chinese). Water Resourc Power. 37:25–28.
- Yang Q,Xie P,Shen H,Xu J,Wang P,Zhang B. 2012. A novel flushing strategy for diatom bloom prevention in the lower-middle Hanjiang River. Water Res. 46(8):2525–2534.
- Yao X, Liu D, Yang Z, Fang X, Tian Z. 2012. Distribution characteristics of phytoplankton community structure in Xiangxi Bay of Three Gorges Reservoir during spring and summer (In Chinese). J Sichuan Univ (Eng Sci Ed). 44:211–220.
- Zhang Z, Huang X. 1991. Research methods of freshwater plankton (In Chinese). Science Press, Beijing.