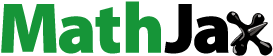
Abstract
We observed episodic zooplanktivory by largemouth bass (Micropterus salmoides; LMB) ≥ 150 mm total length (TL) on the cladoceran Daphnia spp. over two decades in a small oligotrophic lake. While zooplanktivory is beneficial for young-of-year and juvenile LMB, dietary utilization of Daphnia by adults is unusual because zooplankton are not energetically favorable and the LMB buccal cavity is poorly adapted for filter feeding. We classified the threshold of ≥ 38 Daphnia per feeding event as an episode of zooplanktivory based on data from a zooplankton specialist, the bluegill sunfish (Lepomis macrochirus). In this study, 1718 LMB were caught, marked, sampled, and released. Gut contents at the time of capture were collected via gastric lavage. We captured the same 612 LMB 3 or more times. Of these, approximately 26% had at least one episode of zooplanktivory and approximately 6% had multiple episodes. There was no relationship between the population sizes of Daphnia and LMB, but the yearly percentage of individual LMB with at least one episode of zooplanktivory significantly increased with LMB population density. Episodes of zooplanktivory were more likely to occur later in the season when Daphnia were more abundant in the lake. The probability of an individual LMB having a repeat episode of zooplanktivory was not significantly different from expected; however, the probability of a LMB not having a single episode was significantly greater than expected by random chance signifying that some LMB were better than others at eliminating Daphnia from their diets. The probability of episodic zooplanktivory significantly decreased with increasing fish TL and weight but significantly increased with increasing Daphnia abundance. LMB without episodes of zooplanktivory achieved significantly greater maximum weight and body condition factor than those with them, but there was no statistical difference in their maximum TL or longevity (duration between first and final capture of the same LMB). In contrast, LMB with episodes of zooplanktivory had significantly greater instantaneous growth rates than those without. These results suggest that LMB within the resource-limited lake partitioned prey resources when some LMB sporadically targeted under-utilized, energetically unfavorable prey.
Introduction
Numerous foraging studies have calculated the predicted rank order of preferred prey consumption congruent with net energetic gain (Werner Citation1979; Hodgson and Kitchell Citation1987; Kamil et al. Citation1987; Hughes Citation1990). Predators should increasingly avoid prey with lower energetic favorability (i.e. high-cost, low-reward) when prey of higher energetic favorability (low-cost, high-reward) become more available. Conversely, when higher quality prey becomes less abundant, predators are often forced to consume lower quality prey at an increasing rate. However, because many predators, especially piscivores, undergo ontogenetic niche shifts as they mature, their prey preference and energetic payoff varies with predator size class (Nisbet et al. Citation1989; Osenberg et al. Citation1994; Olson Citation1996, Post et al. Citation1997; Post Citation2003). As such, prey items that were energetically favorable and/or selected because of gape limitation when the predators were juveniles may not be preferred and cost-effective when they are adults.
Largemouth bass (Micropterus salmoides; LMB) undergo an ontogenetic niche shift early in their life history, often within the first year, from a diet of small invertebrates to fish (Werner and Gilliam Citation1984; Olson Citation1996). The timing of this shift is highly variable, but it is often associated with LMB total length (TL). The niche shift has been reported to occur between 20 and 140 mm but most often occurs between 40 and 60 mm TL (Bettoli et al. Citation1992; Olson Citation1996; Brown et al. Citation2001). Variability in the hatching of predator and prey populations, growth rates in earlier ontogenetic stages, and the availability of appropriate fish prey contribute to the timing of this ontogenetic niche shift (Olson Citation1996; Mittelbach and Persson Citation1998). As LMB are gape-limited predators (Hambright Citation1991), these factors influence the ability of LMB to effectively carry out size-selective predation that targets prey items with the greatest net energetic gain (Werner Citation1979). Subsequently, juvenile LMB that switch to piscivory before others in their cohort usually have the fastest growth rate (Shelton et al. Citation1979); this is especially important in northern lakes where body size and growth are key indicators of whether a fish will survive through the winter (Gutreuter and Anderson Citation1985; Ludsin and DeVries Citation1997; Mittelbach and Persson Citation1998). The switch to piscivory provides other advantages for LMB including increased fecundity over their lifespan (Post Citation2003). Foraging behavior research on LMB suggests that the abundance and accessibility of prey items influences prey choice (Sammons and Maceina Citation2006; Ahrenstorff et al. Citation2009). Subsequently, individual foraging preferences may vary via resource partitioning (Werner and Gilliam Citation1984; Ebenman and Persson Citation1988). This partitioning can increase the occurrence of foraging on underutilized or unfavorable prey items (Schindler et al. Citation1997).
Our study site, Paul Lake, is a representative glacially formed, resource-limited, and oligotrophic lake located in the north temperate zone of the United States (Wetzel Citation2001) and is near the northern limit of LMB distribution (Post et al. Citation1998). Compared to other lakes in the region with resembling morphometries and trophic classifications, Paul Lake has a reduced fish diversity (Tonn and Magnuson Citation1982) but greater densities reflective of the complete lack of recreational use and exploitation (Hodgson et al. Citation1993). As LMB are characteristic piscivores, the lake offers an opportunity to assess alternative foraging strategies by LMB because they have been consistently the only fish since the 1960s and exclusively the only piscivorous fish in the lake since 1978 (Hodgson and Kitchell Citation1987; Carpenter and Kitchell Citation1993; Dassow et al. Citation2018); therefore, they do not have the means to switch to piscivory beyond occasional cannibalism, which necessitates consumption of other prey with varying lower degrees of net energetic gain. Within this simplified fish community, we had the unique circumstance to investigate density-dependent changes in foraging behavior and individual foraging history in the absence of interspecific competition from other fishes. Research on Paul Lake produced a record of LMB diet and foraging ecology of over 30 years (1981–1982, 1984–2011) (Purdom et al. Citation2015; Brosseau and Hodgson Citation2016). This documentation, for example, has revealed that adult LMB in Paul Lake heavily relied upon benthic invertebrates (Odonata naiads) in the absence of fish (Hodgson and Kitchell Citation1987; Hodgson et al. Citation1993; Hodgson and Hodgson Citation2000; Purdom et al. Citation2015). Furthermore, such an extensive and long-term dataset as this provides the rare benefit of observing phenomena by individuals that may be masked within aggregate data. Specifically, analyzing individual recaptured LMB and their dietary records could identify any unexpected, unique foraging behaviors that would have otherwise been unnoticed within population-level summaries. For instance, by using individual records in lieu of population interpretations, some Paul Lake LMB have previously been shown to develop dietary specialization on high-cost metalimnetic Chaoborus spp. larvae (Rick et al. Citation2011) and water mites (Hydracarina) (Hodgson et al. Citation2008), which are typically distasteful to LMB (Kerfoot et al. Citation1980). These results signify deviations from expected foraging behaviors based on body size, ontogeny, and avoidance. In contrast, a subset of adult LMB also developed an individual preference for cannibalism of high-reward, low-cost young-of-year (YOY) LMB (Dassow et al. Citation2018).
Here, we use a segment (1984–2008, 25 years) of this long-term natural history record of LMB diets from Paul Lake to investigate episodic zooplanktivory on a high-cost, low-reward prey item, Daphnia spp. The large cladoceran, Daphnia, are an important diet item for YOY and juvenile LMB but should not be consumed by adult LMB that increased their size and energetic demand (Werner Citation1979; Hodgson and Kitchell Citation1987). However, Daphnia, which rank among the least energetically favorable prey for adult LMB (Werner Citation1979; Hodgson and Kitchell Citation1987; Hodgson and Hodgson Citation2000), consistently appeared in low levels with low importance in the guts of Paul Lake adult LMB when interpolated across multiple individuals and time using vetted and widely employed population-level metrics (Hodgson and Kitchell Citation1987; He et al. Citation1993; Purdom et al. Citation2015). These frequently employed methods include the application of the Index of Relative Importance (IRI, George and Hadley Citation1979) using individuals as replicates to describe the relative contributions of each food item to the overall population diet breadth. This approach has two weaknesses. First, a population response to density-dependent factors is the sum of numerous individual responses that will often go unnoticed using aggregate summaries (Schindler et al. Citation1997). Second, the IRI does not consider nutritional components, which makes it inadequate and inappropriate for foraging studies investigating energy harvest using prey items with different energetic values (Hart et al. Citation2002).
Using an a posteriori data extraction of this dataset, we examined the discrete dietary histories of individual LMB ≥ 150 mm TL with unique identification tags. This size limit is larger than the upper range of the reported TL where LMB should have already undergone an ontogenetic niche shift (Carlander Citation1977; Keast Citation1985; Bettoli et al. Citation1992). In Paul Lake, LMB of these larger sizes routinely consumed Odanata naids, Chaoborus larvae, Chironomidae pupae, and LMB YOY (Purdom et al. Citation2015), which are all more energetically favorable than Daphnia. Our objective was to better understand the extent of episodic foraging on Daphnia that may have been indiscernible while using the aggregative indices commonly applied during in situ fish population foraging research. We analyzed LMB prey choice records to investigate the existence and extent of episodic zooplanktivory by individuals in an LMB population. Specifically, we analyzed whether its occurrence depends on LMB population size, the time of year, or Daphnia abundance in the lake. We also tested the probability of episodic zooplanktivory reoccurring or never occurring within the same individual. Then, we examined how episodic zooplanktivory affects the body condition of LMB. In combination with the Paul Lake LMB foraging records of Hodgson et al. (Citation2008), Rick et al. (Citation2011), and Dassow et al. (Citation2018), which show individual predator preference, the broad history of prey choice and energetic yield elucidates vital information about long-term population dynamics of a piscivore.
Methods
Study site
Paul Lake is a small (1.7 ha, max depth 15.0 m) oligotrophic lake located in the Upper Peninsula of Michigan, USA (89°32’W; 46°13’N) on the property of University of Notre Dame Environmental Research Center and is known as one of the ‘Cascade Lakes’. It has long served as a control for ecosystem-level manipulations (Carpenter and Kitchell Citation1993; Carpenter et al. Citation2001; Carpenter and Pace Citation2018) including the pioneering whole lake experiment by Hasler (Citation1964). The lake is not open to the public and has been unmanipulated and unexploited since the early 1970s (Leavitt et al. Citation1989; Carpenter and Kitchell Citation1993) except for being labeled with NaH13CO3 in 2001 as part of a food web study (Carpenter et al. Citation2005). Paul Lake supports a fish assemblage dominated by LMB as the only piscivorous fish present (>95% of fish biomass at any one time, making the community effectively monospecific). Over the years, a very small number of minnows and pumpkinseed sunfish (Lepomis gibbosus) entered Paul Lake via a culvert under an earthen dam to the adjacent Peter Lake despite netting on both sides (Dassow et al. Citation2018). For a more complete history of the lake see Leavitt et al. (Citation1989) and Carpenter and Kitchell (Citation1993).
LMB sampling protocol and diet analysis
Paul Lake has been the site of continuous LMB research projects from 1984 to 2011 with a consistent sampling protocol between the years 1984 and 2008 (Carpenter and Kitchell Citation1993; Carpenter and Pace Citation2018; Dassow et al. Citation2018). Compared to the LMB sampled in manipulated lakes for which Paul Lake served as an unaltered control, Paul Lake population-level LMB diet aggregates (Hodgson et al. Citation1993) and foraging rates (He et al. Citation1993) were more consistent through time although all lakes showed variability. LMB were sampled using angling and electrofishing approximately every third week from May to August annually from 1984 to 2010 and averaged seven sampling periods per year. Individuals were measured to the nearest 1.0 mm and weighed to the nearest 1.0 g. The mean annual sample size for LMB ≥ 150 mm TL was 171 fish per year with a mean sample size of 22.7 fish per sampling effort. Individual stomach contents were collected using gastric lavage and preserved in 95% ethanol within 1 hour of capture. All LMB were released after processing. LMB were collected under appropriate collection permits using approved IACUC protocols. Specific diet sampling techniques are explained in greater detail in Hodgson and Kitchell (Citation1987) and Hodgson et al. (Citation1993). These methods have been repeatedly validated in reference to capturing population-level variability (Hodgson and Cochran Citation1988; Hodgson et al. Citation1989; Cline et al. Citation2012; Brosseau and Hodgson Citation2016). Further explanation on the consistency of the long-term sampling protocols and their validity for a posteriori investigations is reported in Dassow et al. (Citation2018).
LMB marking and recapturing
All LMB were assigned a unique identifier from either Floy or PIT tagging. The first time a LMB ≥ 150 mm TL was caught it was marked with a tag, sampled, and released. Any subsequent catch of the same LMB was considered a recapture. For clarity, a LMB caught twice had a mark and one recapture; a LMB caught three times had a mark and two recaptures. A double-tagging study to assess frequency of tag loss showed that it was insignificant (Hodgson et al. Citation1993). Individual LMB were frequently caught over multiple years. As a proxy for individual LMB age, we calculated longevity as the duration between first and final capture of the same fish.
LMB density
Annual whole lake LMB ≥ 150 mm TL population densities were calculated by averaging mark and recapture counts from mid-May and mid-August of the same year following the methods of Ricker (Citation1975) of fish captured from boat-mounted electroshocking. This method was consistent through all years of study. Additional information is reported in Hodgson et al. (Citation1993), Purdom et al. (Citation2015), and Dassow et al. (Citation2018).
Daphnia density
For this study, we focused solely on zooplanktivory on Daphnia because cladocerans dominate the Paul Lake zooplankton community and large-bodied Daphnia are the predominant cladoceran (Soranno et al. Citation1993a, Citation1993b). Zooplankton samples were collected weekly from late May to late August each year. We did not have zooplankton abundance data past 2008; hence we focused on the data collected between 1984 and 2008. Zooplankton were sampled using 2 vertical tows with an 80 µm mesh net through the upper two-thirds of the water column at the deepest point then pooled. Samples were filtered through 75 µm mesh, preserved in 75% ethanol, and the mean zooplankton abundance calculated for each week. Further details are reported in Carpenter and Kitchell (Citation1993) and Carpenter et al. (Citation2001).
Data selection
Within the data segment we analyzed (1984–2008), 1718 LMB were captured, marked, sampled, and released. Of those, we focused our analyses on two subsets of LMB. First, LMB caught 3 or more times (n = 612) were used to explore the probability of episode occurrence as well as the potential influence of episodic zooplanktivory on LMB body conditions in comparison to fish that did not exhibit any episodes. The 3-catch minimum was chosen as a tradeoff between examining multiple catches per fish while maintaining a large sample size. Second, LMB caught once (n = 728) represented a pool of independently sampled individuals free from the complications of choosing among recapture events per fish. Fish caught once were used to examine overall trends between episode occurrence and LMB morphology, LMB and Daphnia population structures, and seasonality. Variables examined were limited to those collected as part of the Paul Lake dataset; all were selected based on the hypothesis that each may influence, or be influenced by, LMB episodic zooplanktivory. Due to the a posteriori nature of this study, a comprehensive view of all potential variables influencing episodic zooplanktivory was not possible. As such, the chosen variables likely do not represent a complete model, thus all variables were analyzed individually in this exploratory effort (see Dassow et al. Citation2018 for additional information about this dataset and a posteriori analyses). Where multiple related tests were conducted, alpha values were Bonferroni corrected to reduce the likelihood of type I error.
Designating episodic zooplanktivory
We defined our threshold of episodic zooplanktivory by LMB based on data collected from a related Centrarchid, the bluegill sunfish (Lepomis macrochirus). The bluegill is considered a zooplankton specialist (Werner and Hall Citation1974) and has an extensive literature summarizing empirical foraging observations, experimental feeding trials, and modeled predation upon cladocerans. In a comparable lake with a similarly simplified fish assemblage nearby to Paul Lake within the same drainage basin (East Long Lake; 1.75 km away), we sampled gut contents from 150 bluegill ≥ 150 mm TL during 1992 using the same protocol reported here. There was no significant difference in Daphnia abundance between East Long and Paul lakes during 1992 (Wilcoxon rank sum test, Z = 1.23, p = 0.2197). We found that individuals preying on Daphnia averaged 37.7 (SE ± 13.4) per gut (collected but unreported data as part of Carpenter et al. Citation2001). This number of Daphnia per gut was within ranges of bluegill feeding data reported by O’Brien et al. (Citation1976), Elmore et al. (Citation1983), and Li et al. (Citation1985) that analyzed their specialization upon Daphnia and copepods. Thus, if a LMB gut sample had ≥ 38 Daphnia, then it was designated as an episode of zooplanktivory. The presence of 38 or more Daphnia in the gut sample of a mature LMB is unlikely an accident due to various morphological and behavioral attributes. The buccal cavity of mature LMB is poorly equipped for zooplanktivory (Alexander Citation1970; Drenner et al. Citation1978). Additionally, LMB employ ram feeding as opposed to size-selective filtering via pharyngeal teeth (Nyberg Citation1971; Carroll et al. Citation2004; Higham et al. Citation2005; Wainwright and Day Citation2007). For a more detailed examination, data for LMB that were caught 3 or more times were also subdivided into categories by how many episodes of zooplanktivory were observed per fish (1, 2, and 3 events). However, due to vast differences in the number of observations among these subdivision categories, statistical analysis was restricted to the broader categories of LMB that demonstrated episodes and those that did not.
Statistical analysis of recaptured LMB
We tested whether episodes of zooplanktivory reoccurred or never occurred within the first 3 catches of an LMB more often than would be expected by random chance. If we designate an episode of zooplanktivory as Z and non-episodes as N, the probability of observing a single episode of zooplanktivory was calculated for the first catch of these LMB by dividing the number of observed episodes of zooplanktivory (Z) by the total number of fish caught (55/612). This value (0.08987) was used to determine the expected probabilities of observing at least two episodes (probability of ZZN, ZNZ, NZZ, or ZZZ = 0.02932) and of observing zero episodes (probability of NNN = 0.7543) within three consecutive catches. The observed probabilities were calculated for the first three recorded catches of each LMB and compared to the expected probabilities using exact one-sided binomial tests with a Bonferroni corrected alpha value of 0.0250.
The effect of episodic zooplanktivory on LMB body condition was examined by determining whether fish that did or did not exhibit at least one episode differed in their maximum TL and/or weight. With only a few exceptions, maximum TL and weight values came from the last recapture. Maximum TL and weight data were not normally distributed; therefore, significant differences were determined using Wilcoxon rank sum analyses. We calculated the condition factor (K) from the maximum TL and weight (W) of each LMB, which is an index of ‘plumpness’ that implies maturity and gonad development following Moyle and Cech (Citation1996):
The difference in K between fish that did and did not exhibit episodic zooplanktivory was analyzed with a t-test as these data were normally distributed. Next, to determine if episodes influenced LMB longevity, the total span of time from the first to final observed catch per LMB was calculated and the difference analyzed via Wilcoxon rank sum test. Finally, the weights from the first and final catches were used to calculate the instantaneous growth rate (G0, the percentage growth rate per day per instant of time) as described by Allen (Citation1985):
where W1 and W2 represent the weight of a particular fish during its first and second (last) collection and t is the time duration in days between samples. The difference in G0 between fish that did and did not exhibit episodic zooplanktivory was analyzed via a Wilcoxon rank sum test as these data failed the assumption of normality.
Statistical analysis of LMB caught once
Linear regression was utilized to examine the relationship between mean annual population size of LMB ≥ 150 mm and the response variables mean annual Daphnia density per m2, mean annual Daphnia consumption, and the yearly percentage of LMB exhibiting at least one episode of zooplanktivory; these tests used a Bonferroni corrected alpha value of p = 0.0167. We used logistic regression analysis to determine the probability of exhibiting zooplanktivore episodes across LMB TL (n = 728), LMB weights (n = 718), and Daphnia density (n = 655) per m2 within the lake; the alpha value was Bonferroni corrected to p = 0.0167. For Daphnia density, because the plankton trawls and fish surveys did not occur on the same dates, density was matched to each LMB by grouping the Daphnia values into 10-day increments by Julian date (DOY). If more than one Daphnia density was available for a 10-day increment, the mean value was utilized in the analysis.
To determine whether LMB zooplanktivore episodes were more likely to occur based on the time of year that LMB were caught, we performed a chi-square analysis on the seasonal timing (early vs. late) of episode occurrence within LMB. LMB were defined as being caught in the early season if the catch date occurred on or prior to Julian date 195, while late season LMB were caught after this date. We chose this bifurcation to be consistent with the analysis of the same Paul Lake LMB population by Dassow et al. (Citation2018), where the threshold was based on spawning activity. We also examined whether Daphnia density significantly differed between the early and late sampling season; these data were not normally distributed and were therefore analyzed via a Wilcoxon rank sum test.
Results
The whole-lake population of LMB ≥ 150 mm averaged 256 individuals per year (95% CI 218–334), with a minimum of 91 (95% CI 71–114) in 1993 and a maximum 457 (95% CI 380–574) in 1999. The longevity between first and final capture averaged 879 days (median = 735, maximum = 5489). Of the 612 LMB caught 3 or more times, 454 never exhibited episodic zooplanktivory and averaged < 2 Daphnia per gut sample, whereas 158 were observed having at least one episode of zooplanktivory and averaged 23x more Daphnia in their guts (). The maximum number of Daphnia found in one gut sample was 696; 57 LMB consumed > 100 Daphnia in at least one episode. The average number of Daphnia per gut increased the more frequently an individual LMB was recaptured during an observed episode of zooplanktivory ().
Table 1. Individual LMB captured 3 or more times between 1984 and 2008.
Table 2. LMB with episodes of zooplanktivory subdivided by the number of times these recaptured fish were observed with ≥ 38 Daphnia in their gut.
There was no statistical relationship between the density of LMB ≥ 150 mm and the density of Daphnia in the lake (r2 = 0.00003, p = 0.981, ) or the number of Daphnia consumed (r2 = 0.14, p = 0.0650, ). These results are consistent with previous study in Paul Lake that showed population-level composites of zooplanktivory varied by year but were not correlated with LMB density (Purdom et al. Citation2015). However, the annual percentage of LMB with a zooplanktivore episode was significantly related to the number of LMB ≥ 150 mm (r2 = 0.23, p = 0.0161, ).
Figure 1. Annual estimate of LMB population density for fish measuring ≥ 150 mm in comparison to (A) mean Daphnia density per m2, (B) mean number of Daphnia observed within LMB gut contents, and (C) the annual percentage of LMB exhibiting at least one episode of zooplanktivory.
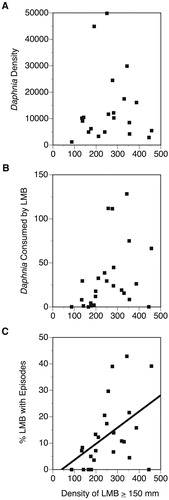
The observed probability of at least 2 episodes of zooplanktivory occurring within the first three catches for an LMB (0.03268) was not statistically different from the expected value (p = 0.343). In contrast, the observed probability of not observing an episode within three consecutive LMB catches (0.7892) was significantly greater than the expected value (p = 0.0236). The probability of LMB exhibiting zooplanktivorous episodes significantly decreased with increasing TL (χ2 = 46.92, p < 0.0001; ) and weight (χ2 = 53.80, p < 0.0001; ), whereas the probability of episodes increased with increasing Daphnia density (χ2 = 11.24, p = 0.0008; ). LMB zooplanktivore episodes were more frequent later in the season than earlier in the season (, χ2 = 51.15, p < 0.0001). Daphnia were significantly more abundant later in the sampling season, with approximately 25% more Daphnia observed later in the season (, Z = 2.16, p = 0.0309).
Figure 2. Logistic regression estimating the probability of observing episodes of zooplanktivory by LMB as a function of: (A) LMB TL, (B) LMB weight, and (C) Daphnia density per m2.
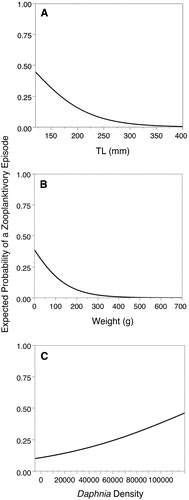
Figure 3. (A) the percentage of LMB observed to exhibit episodes of zooplanktivory that were caught early in the season on or prior to Julian DOY 195 or after this date; (B) the mean number of Daphnia observed within Paul Lake per m2 during early and late season sampling. Error bars represent ± 1 SE.
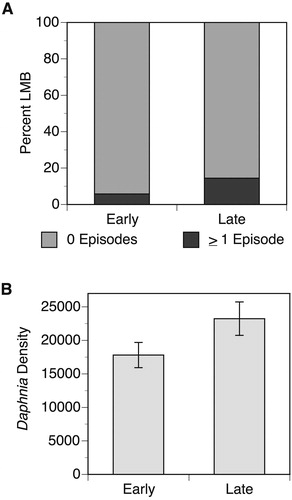
There was no significant difference between the maximum TL of LMB that did and did not exhibit zooplanktivore episodes (Z = −1.93, p = 0.0538); however, those LMB that never exhibited a zooplanktivore episode attained a significantly greater maximum weight (Z = −2.36, p = 0.0184). Similarly, those LMB that never exhibited a zooplanktivore episode achieved a significantly greater condition factor (K) than those with episodes of zoopklantivory (t = −2.22, p = 0.0270). There was no significant difference in the amount of time between the first and final catches (longevity) for LMB that did and did not exhibit episodic zooplanktivory (Z = 1.49, p = 0.136). Finally, the instantaneous growth rate was significantly greater (Z = 3.56, p = 0.0004) for LMB with at least one reported zooplanktivore episode (G0 = 0.15 ± 0.018) than those without (G0 = 0.13 ± 0.011).
Discussion
Analyzing the long-term foraging dynamics of LMB in Paul Lake with unique records allowed us to elucidate unexpected foraging behaviors that were inconspicuous in aggregative, population-level data. Previous analyses of the population showed foraging patterns were density-dependent and consumed a wide diet breadth ranging from zooplankton to benthic invertebrates to YOY LMB (Hodgson and Kitchell Citation1987; Hodgson and Hodgson Citation2000; Purdom et al. Citation2015). Generalist populations, such as LMB, often have considerable niche variation with instances of foraging individualization (Lomnicki Citation1988; Schindler et al. Citation1997; Bolnick et al. Citation2003; Bolnick et al. Citation2007; Dall et al. Citation2012). As such, changes in prey choice likely occur at the individual level as many unique foraging responses constitute a population-level change in prey selectivity. These are often difficult to detect in pooled sampling programs, but the individual nature of prey choice through long-term diet data collection from recaptured individuals may reveal the breadth and extent of dietary specialization (Schindler et al. Citation1997). By investigating dietary histories of individual LMB over multiple decades, we were able to determine that a specific prey resource, Daphnia, was partitioned and not utilized equally within the LMB population. During our exploratory analysis of the long-term Paul Lake dataset, we sought to identify any potential LMB that repeatedly consumed Daphnia, which could then be further investigated for individual foraging specialization similarly to previous cases of preference for mites (Hodgson et al. Citation2008), Chaoborus (Rick et al. Citation2011), and YOY LMB (Dassow et al. Citation2018). Foraging on Daphnia was most likely too sporadic to conservatively categorize some Paul Lake LMB as zooplankton specialists; however, several LMB demonstrated episodes of zooplanktivory, including individuals with multiple episodes. Our a posteriori data extraction exercise allows for further exploration afforded by such a large database that may not have been otherwise possible with shorter records of study. Below, we ask questions regarding patterns; offer broad, possible explanations; and propose future lines of research.
Are LMB selecting Daphnia?
Based on our 25-year natural history record, we propose that a subset (26% of LMB captured 3 or more times) of Paul Lake LMB ≥ 150 mm TL actively selected Daphnia as prey on occasions (i.e. episodic zooplanktivory). Paul Lake LMB population-level diet has previously been shown to increasingly add less cost-effective prey during periods of increased competition (Hodgson and Kitchell Citation1987; Schindler et al. Citation1997; Hodgson and Hodgson Citation2000; Purdom et al. Citation2015). While we found neither a relationship between Daphnia and LMB population densities nor a relationship between the number of Daphnia consumed and LMB population density, this was not entirely unexpected. Compared to other lakes in the watershed with contrasting food webs, Paul Lake zooplankton was dominated by an abundance of large-bodied Daphnia indicating low impact to their population density from zooplanktivory by fish (Soranno et al. Citation1993a, Citation1993b). Furthermore, episodes of zooplanktivory by some LMB were unexpected based on energetics, morphology, and behavior. Consistent with adult LMB buccal cavity adaptations (Alexander Citation1970; Drenner et al. Citation1978), foraging cost curves (Werner Citation1979; Hodgson and Kitchell Citation1987), and bioenergetics modeling (Hodgson and Hodgson Citation2000), Daphnia are predicted to be among the last prey selected, if selected at all. However, we found 158 LMB that were captured 3 or more times with Daphnia counts resembling those of the zooplankton specialist bluegill sunfish. These data suggest it is unlikely that the Daphnia found in LMB guts were the product of accident or coincidence from buccal suction or ram feeding of other prey. Although experimental feeding trials would aid in our determination of individual foraging preference and size selectivity (Hambright Citation1991), a comparison of experimental and empirical data collected from natural LMB populations showed prey were consistently smaller than modeled optimal size and selection rate matched availability (Hoyle and Keast Citation1987). In Paul Lake, the probability of individual LMB exhibiting an episode of zooplanktivory significantly increased with increasing Daphnia abundance. Additionally, we found LMB with episodes of zooplanktivory were more likely to be caught later in the season, when more Daphnia were available, than during the early season. This may be caused by the movement of some LMB from their reproductive nests in the littoral zone early in the season to the pelagic zone (Scott and Crossman Citation1973; Becker Citation1983), where Daphnia are more abundant (Brosseau and Hodgson Citation2016), later in the season.
Further examination of LMB behavior suggests that consuming Daphnia was intentional. Previously in Paul Lake, Daphnia predation partly supplemented LMB diets at the population level during periods of high population density and increased intraspecific competition before a partial winter kill during a 2-year study (Hodgson and Kitchell Citation1987). Additionally, there was evidence of size-selective zooplanktivory of Daphnia in the water column. Specifically, Daphnia sampled from LMB guts, on average, had carapace lengths nearly twice as long than ones sampled from the water column. Furthermore, the prevalence of diel vertical migration (DVM), which is a behavioral anti-zooplanktivory mechanism against visual predators that uses darkness as refugia, should decrease in the absence of zooplanktivory (Dodson Citation1990). However, Daphnia in Paul Lake demonstrated DVM (Brosseau et al. Citation2012; Farrell and Hodgson Citation2012), suggesting that Daphnia exhibited anti-zooplanktivory behavior and those LMB that consumed Daphnia moved into areas to actively forage on them. Similar behavior was previously identified where a subset of adult LMB in Paul Lake descended into the metalimnion during the day to forage on Chaoborus, which also employ DVM (Rick et al. Citation2011). While Chaoborus larvae are also important predators of Daphnia in Paul Lake (Carpenter and Kitchell Citation1993), their predation should not induce DVM by Daphnia because they are tactile hunters that feed at night (Swift and Forward Citation1981; Greene Citation1983; Kruppert et al. Citation2019). Rather, Daphnia undergo morphological changes and produce ‘neckteeth’ (cuticular protuberances) as their corresponding anti-predatory response to Chaoborus (Soranno et al. Citation1993c; Riessen Citation1999). Brosseau and Hodgson (Citation2016) found no sampling bias between littoral and pelagic zones in Paul Lake LMB, but further suggested that prey importance matched differences in prey availability between the zones and which zone LMB were caught.
Why are LMB selecting Daphnia?
We posit that Paul Lake LMB predation of Daphnia may have been the product of resource partitioning based on two lines of evidence. First, the yearly percentage of individual LMB with at least one episode of zooplanktivory was significantly related to LMB population density, which potentially demonstrated the need for increasingly including lower-ranked prey with population growth. Second, the probability of an individual LMB not having a single episode of zooplanktivory was significantly greater than would be expected by random chance. This means that certain individuals were likely better at eliminating Daphnia from their diets as predicted by energetic favorability than other LMB that may have resorted to consuming a lesser resource. Bioenergetics modeling showed that Paul Lake LMB were regularly food limited (He et al. Citation1993). Similarly, an expanded bioenergetics modeling trial that tested functional responses concluded that LMB in Paul Lake consistently exhibited low predation rates and obtained only a fraction of their maximum daily consumption rate (Essington et al. Citation2000). High-quality prey in Paul Lake were seasonal, such as YOY LMB (Post et al. Citation1997; Post Citation2003; Purdom et al. Citation2015; Dassow et al. Citation2018) or sporadic, including terrestrial vertebrates, crayfish, and a very small number of fish from Peter Lake (Hodgson and Hodgson Citation2000; Hodgson and Hansen Citation2005; Dassow et al. Citation2018). As such, they were not reliable staples beyond occasional opportunism. As an effectively monospecific fish assemblage (Elser et al. Citation1986) with intra- and intercohort competition (Dassow et al. Citation2018), the LMB population had the potential to develop individualized dietary behavior. Unique prey choice is often a response to intraspecific competition (Ward et al. Citation2006) that leads to preferences by individuals in a population (Schindler et al. Citation1997). For instance, trout inhabiting the same location developed individual prey preference and reduced dietary overlap (Bryan and Larkin Citation1972). Similarly, as intraspecific competition for food resources between Paul Lake LMB intensified, they increasingly relied upon lower-quality prey, but they did so in an unequal manner (Schindler et al. Citation1997). Specifically, LMB showed greater diet consistency, i.e. diet overlap between successive captures, meaning that individuals showed repeated specialized foraging behaviors for certain prey items that are different from their conspecifics. For example, some individuals repeatedly consumed Odonata naiads, others repeatedly consumed Chaoborus larvae, and so on, effectively partitioning the food resources without increasing overall population diet breadth. Ultimately, in the current study, the episodic zooplanktivory of Daphnia by some LMB may have reduced dietary overlap because prey resources were not being utilized equally between individuals.
Under conditions with limited availability of higher-quality prey in Paul Lake, one could propose a reasonable hypothesis that inferior competitors were relegated to incorporating episodes of zooplanktivory. In studies of predatory fish distribution and prey patchiness, patch selection was predicated on profitability (Milinski Citation1984; Regelemann Citation1984). Specifically, superior competitors decided where to forage and dispersed earlier with less repositioning than inferior competitors that dispersed around patch occupation by their superiors (Milinski Citation1984). Furthermore, experiments on the association between aggression and food intake in rainbow trout (Oncorhynchus mykiss) concluded that dominant individuals denied access to subordinates; individual recognition reduced the costs of competition and contesting resources (Johnsson Citation1997). LMB have also been shown to establish hierarchies based on dominance where they actively defend territories (Heidinger Citation1976) and exhibit home site fidelity (Hodgson et al. Citation1998; Waters and Noble Citation2004). Previous studies in Paul Lake documented that during rare periods when high-quality prey became available, a subset of LMB, presumably superior competitors, quickly switched to them, maximized their consumption and energetic yield, and foraged them to extirpation (Hodgson and Hodgson Citation2000; Purdom et al. Citation2015). Additionally, foraging behaviors and preferences that are learned early in life history can be maintained later in life (Colgan et al. Citation1986; Hughes et al. Citation1992), and the persistence of a peculiar foraging strategy throughout its life history may explain the presence of specialized behavior. Similarly, a model of foraging learning found that if the cost of switching prey is too high, then the predator should remain committed to a prey source assuming it deems the supply as sufficient (Dukas and Clark Citation1995). In the current study, a familiar foraging behavior or competitor may have placed emphasis on perceived successful prey capture rates, as Daphnia were consistently abundant, at the expense of increased net energetic gain.
What are the tradeoffs for LMB selecting Daphnia?
The unexpected episodes of zooplanktivory by Paul Lake LMB may be the possible application of a competitive compromise resulting in a reproductive tradeoff. As Daphnia are a low-profit prey for adult LMB, we expected that the body condition of those that demonstrated episodic zooplanktivory would be decreased compared to those that did not. A competition study for shared food resources and growth in amago trout (Oncorhynchus masou ishikawae) found that hierarchy caused unequal prey acquisition and growth depensation, which is the between-individuals difference in growth rates (Hakoyama and Iguchi Citation2001). Moreover, they concluded growth depensation caused long-term differences in resource acquisition and spatial distribution. Modifications to life histories are common responses by animal taxa with indeterminate growth in response to various stresses (Kozlowski Citation1996). For example, the aquatic snail Stagnicola elodes supported growth more than reproduction while under nutrient limitation, suggesting that it consistently performed at suboptimal rates and shifts food resources as a safety measure before shortages occur (Rollo and Hawryluk Citation1988). While experiencing food limitation, winter flounder (Pseudopleuronectes americanus) has been shown to maintain body size at the cost of gonad development (Tyler and Dunn Citation1976). Additionally, bachelor parrotfish (parisoma viride) traded growth against current reproduction to increase their likelihood of becoming territorial males with increased reproductive success (Rooij et al. Citation1995).
Accordingly, we found the probability of LMB exhibiting epispodic zooplanktivory significantly decreased with increasing LMB TL and weight. These data suggest that either smaller LMB were more likely to select Daphnia than larger LMB or those that consume Daphnia were less likely to grow as large as LMB that do not. However, while LMB without episodes of zooplanktivory achieved significantly greater maximum weight than those with episodes, there was no statistical difference in their maximum TL or longevity. These results indicate a difference in condition factor (K), or index of ‘plumpness’ (Moyle and Cech Citation1996). This index is a ratio of weight to TL, implying that fish with higher values are ‘plumper’ and have increased fitness because they have more energy to invest in gonadal development. Furthermore, it surmises that food availability is the mechanism. We found that LMB without a single episode of zooplanktivory had significantly greater condition factors (K) than those with episodes of zooplanktivory. Here, the LMB lacking episodes of zooplanktivory may have grown heavier over the same periods because they were likely able to invest more in gonad development, but LMB with episodes may have exploited Daphnia to ensure survival and somatic growth or maintenance at the expense of gonadal tissue. Unfortunately, we lack gonad data via necropsy because the hallmark of our multidecadal protocol was marking and recapturing live individuals. Nevertheless, Post et al. (Citation1998) found that LMB reproduction and recruitment in Paul Lake were controlled through a multitude of mechanisms, but elucidated a key point germane to the current study. Specifically, high numbers of adults did not yield large recruitment cohorts. This relationship may be influenced by variable investment in gonadal and somatic growth within the population. For example, the number of spawned eggs and the number of adults in a population is not consistently correlated and is a poor metric of successful recruitment due to complex interactions of factors including food availability and adult body condition (Elliot Citation1984; Sissenwine Citation1984; Post et al. Citation1998). In Paul Lake, adult LMB body condition was inversely correlated with population density due to the diminishing abundance of higher quality prey (Schindler et al. Citation1997). This establishes the possibility that only a subset of LMB were capable of consuming better prey and investing that corresponding energy in gonadal development.
Consistent with the assumptions of the condition factor (K) that indicate immature fish grow faster than their reproductively mature conspecifics (Moyle and Cech Citation1996), we found that LMB demonstrating episodic zooplanktivory had significantly greater instantaneous growth rates than those that did not. If it is assumed that LMB with episodic zooplanktivory were less likely to increase gonadal investment and reproduce than those without episodes, then they had lower overall energy requirements. Following spring spawning, reproductive female LMB abandon the nest (Kramer and Smith Citation1962), but their investment in gonad development is year-round and costly, requiring up to nearly 15x more energy than males (Adams et al. Citation1982). Reproductive male LMB, which do remain with the nest to guard their brood, exhibit costly behaviors, including burst swimming to fend off potential predators; energy expenditures of nesting males are approximately 2x greater than their non-nesting counterparts (Cooke et al. Citation2002).
Conclusions
Our results describing an unusual foraging behavior by LMB may be of interest to fisheries scientists and managers, especially when resolving population dynamics in a nutrient-limited lake at the northern edge of its distribution. Future research in Paul Lake using experimental feeding trials; measuring specific morphological traits, including gonads, jaws, and gill rakers; analyzing genetic loci; and applying liability-threshold trait models would undoubtedly improve our understanding of why certain LMB develop unique foraging patterns. Specialization on Chaoborus (Rick et al. Citation2011), water mites (Hodgson et al. Citation2008), YOY LMB (Dassow et al. Citation2018), and now episodic consumption of Daphnia demonstrates a LMB natural history that is far more individualistic than previously thought.
Acknowledgments
We thank the University of Notre Dame Environmental Research Center, along with many students and technicians for logistical support. C.J. Hodgson managed the Paul Lake database. M.M. Cutwa, J.J. Montemarano, and E.A. Albright provided helpful comments on earlier drafts of this manuscript. We also thank St. Norbert College and Georgia Southern University for their support of undergraduate research.
Disclosure statement
No potential conflict of interest was reported by the authors.
Data availability statement
The majority of the data used in this study are openly available at: https://lter.limnology.wisc.edu/index.php/data. Due to changes in multi-institutional collaborations, personnel, project scopes, funding sources, data management requirements, and intellectual property ownership over two decades, not all data have been released to the public domain.
Additional information
Funding
Notes on contributors
Tyler J. Butts
Tyler J. Butts was an undergraduate researcher at St. Norbert College and is currently a doctoral student at Iowa State University.
Jay Y. S. Hodgson
Jay Y. S. Hodgson was an undergraduate researcher at St. Norbert College and is now an Associate Professor of Biology at Georgia Southern University.
Michele Guidone
Michele Guidone is an Associate Professor of Biology at Georgia Southern University.
James R. Hodgson
James R. Hodgson is a Professor Emeritus at St. Norbert College and was the principal investigator of the long-term Paul Lake largemouth bass research projects.
References
- Adams SM, McLean RB, Parrotta JA. 1982. Energy partitioning in largemouth bass under conditions of seasonally fluctuating prey availability. Trans Am Fish Soc. 111(5):549–558.
- Ahrenstorff TD, Sass GG, Helmus MR. 2009. The influence of littoral zone coarse woody habitat on home range size, spatial distribution, and feeding ecology of largemouth bass (Micropterus salmoides). Hydrobiologia. 623(1):223–233.
- Alexander RM. 1970. Mechanics of feeding action of various teleost fishes. J Zool. 162(2):145–156.
- Allen KR. 1985. Comparison of the growth rate of brown trout (Salmo trutta) in a New Zealand stream with experimental fish in Britain. J Anim Ecology. 54(2):487–495.
- Becker GC. 1983. Fishes of Wisconsin. Madison (WI): University of Wisconsin Press; p. 1052.
- Bettoli PW, Maceina MJ, Noble RL, Betsill RK. 1992. Piscivory in largemouth bass as a function of aquatic vegetation abundance. N Am J Fish Manage. 12(3):509–516.
- Bolnick DI, Svanback R, Araujo MS, Persson L. 2007. Comparative support for the niche variation hypothesis that more generalized populations also are more heterogeneous. Proc Natl Acad Sci USA. 104(24):10075–10079.
- Bolnick DI, Svanback R, Fordyce JA, Yang LH, Davis JM, Hulsey CD, Forister ML. 2003. The ecology of individuals: incidence and implications of individual specialization. Am Nat. 161(1):1–28.
- Brosseau C, Cline TJ, Cole JJ, Hodgson JR, Pace ML, Weidel BC. 2012. Do Daphnia use metalimnetic organic matter in a north temperate lake? An analysis of vertical migration. IW. 2(4):193–198.
- Brosseau C, Hodgson J. 2016. Do littoral sampling schemes adequately represent largemouth bass diets? Bios. 87(2):67–72.
- Brown GE, LeBlanc VJ, Porter LE. 2001. Ontogenetic changes in the response of largemouth bass (Micropterus salmoides, Centrarchidae, Perciformes) to heterospecific alarm pheromones. Ethology. 107(5):401–414.
- Bryan JE, Larkin PA. 1972. Food specialization by individual trout. J Fish Res Board Can. 29(11):1615–1624.
- Carlander KD. 1977. Handbook of freshwater fishery biology: life history data on centrarchid fishes of the United States and Canada. Ames (IA): Iowa State University Press.
- Carpenter SR, Cole JJ, Hodgson JR, Kitchell JF, Pace ML, Bade D, Cottingham KL, Essington TE, Houser JN, Schindler DE. 2001. Tropic cascades, nutrients, and lake productivity: whole-lake experiments. Ecol Monogr. 71(2):163–186.
- Carpenter SR, Cole JJ, Pace ML, Van de Bogert M, Bade DL, Bastviken D, Gille CM, Hodgson JR, Kitchell JF, Kritzberg ES. 2005. Ecosystem subsides: terrestrial support of aquatic food webs from 13C addition to contrasting lakes. Ecology. 86(10):2737–2750.
- Carpenter SR, Kitchell JF, editors. 1993. The trophic cascade in lakes. New York (NY): Cambridge University Press; p. 385.
- Carpenter SR, Pace ML. 2018. Synthesis of a 33-yr series of whole-lake experiments: effects of nutrients, grazers, and precipitation-driven water color on chlorophyll. L&O Letters. 3:419–427.
- Carroll AM, Wainwright PC, Huskey SH, Collar DC, Turingan RG. 2004. Morphology predicts suction feeding performance in centrarchid fishes. J Exp Biol. 207(22):3873–3881.
- Cline TJ, Weidel BC, Kitchell JF, Hodgson JR. 2012. Growth response of largemouth bass (Micropterus salmoides) to catch-and-release angling: a 27-year mark-recapture study. Can J Fish Aquat Sci. 69(2):224–230.
- Colgan PW, Brown JA, Orsatti SD. 1986. Role of diet and experience in the development of feeding behaviour in largemouth bass, Micropterus salmoides. J Fish Biol. 28(2):161–170.
- Cooke SJ, Philipp DP, Weatherhead PJ. 2002. Parental care patterns and energetics of smallmouth bass, Micropterus dolomieu, and largemouth bass, Micropterus salmoides, monitored with activity transmitters. Can J Zool. 80(4):756–770.
- Dall SRX, Bell AM, Bolnick DI, Ratnieks FLW. 2012. An evolutionary ecology of individual differences. Ecol Lett. 15(10):1189–1198.
- Dassow CJ, Collier A, Hodgson JYS, Buelo CD, Hodgson JR. 2018. Filial cannibalism by largemouth bass (Micropterus salmoides): a three-decade natural history record from a small northern temperate lake. J Freshw Ecol. 33(1):361–379.
- Dodson S. 1990. Predicting diel vertical migration of zooplankton. Limnol Oceanogr. 35(5):1195–1200.
- Drenner RW, Strickler JR, O’Brien WJ. 1978. Capture probability: the role of zooplankton escape in selective feeding of planktivorous fish. J Fish Res Board Can. 35(10):1370–1373.
- Dukas R, Clark CW. 1995. Searching for cryptic prey: a dynamic model. Ecology. 76(4):1320–1326.
- Ebenman B, Persson L. 1988. Size-structured populations: ecology and evolution. New York (NY): Springer; p. 284.
- Elliot JM. 1984. Numerical changes and population regulation young migratory trout Salmo trutta in a lake district stream, 1966-83. J Anim Ecol. 53:327–350.
- Elmore JL, Vodopich DS, Hoover JJ. 1983. Selective predation by bluegill sunfish (Lepomis macrochirus) on three species of Diaptomus (Copepoda) from subtropical Florida. J Freshw Ecol. 2(2):183–192.
- Elser MM, Elser JJ, Carpenter SR. 1986. Paul and Peter Lakes: a liming experiment revisited. Am Midl Nat. 116(2):282–295.
- Essington TE, Hodgson JR, Kitchell JF. 2000. The role of satiation in the functional response of a piscivore. Can J Fish Aquat Sci. 57(3):548–556.
- Farrell AM, Hodgson JR. 2012. Zooplankton diel migrations in lakes of contrasting food webs. Bios. 83(1):12–16.
- George EL, Hadley WF. 1979. Food and habitat partitioning between rock bass (Ambloplites rupestris) and smallmouth bass (Micropterus dolomieui) young of the year. Trans Am Fish Soc. 108(3):253–261.
- Greene CH. 1983. Selective predation in freshwater zooplankton communities. Int Rev Ges Hydrobiol Hydrogr. 68(3):297–315.
- Gutreuter SJ, Anderson RO. 1985. Importance of body size to the recruitment process in largemouth bass. Trans Am Fish Soc. 114(3):317–327.
- Hakoyama H, Iguchi K. 2001. Transition from a random to an ideal free to an ideal despotic distribution: the effect of individual difference in growth. J Ethol. 19(2):129–137.
- Hambright KD. 1991. Experimental analysis of prey selection by largemouth bass: role of predator mouth width and prey body depth. Trans Am Fish Soc. 120(4):500–508.
- Hart RK, Calver MC, Dickman CR. 2002. The index of relative importance: an alternative approach to reducing bias in descriptive studies of animal diets. Wildl Res. 29(5):415–421.
- Hasler AD. 1964. Experimental limnology. BioScience. 14(7):36–38.
- He X, Kitchell JF, Hodgson JR, Wright RA, Soranno PA, Lodge DM, Cochran PA, Benkowski DA, Bouwes NW. 1993. Roles of fish predation: piscivory and planktivory. In: Carpenter SR, Kitchell JF, editors. The trophic cascade in lakes. New York (NY): Cambridge University Press; p. 85–102.
- Heidinger RC. 1976. Synopsis of biological data on the largemouth bass Micropterus salmoides (Lacapède 1802). FAO (Food and Agriculture Organization of the United Nations) Fisheries Synopsis 115. Rome (Italy); 85 pp. http://www.fao.org/3/ap918e/ap918e.pdf.
- Higham TE, Day SW, Wainwright PC. 2005. Sucking while swimming: evaluating the effects of ram speed on suction generation in Bluegill sunfish Lepomis macrochirus using digital particle image velocimetry. J Exp Biol. 208(Pt 14):2653–2660.
- Hodgson JR, Carpenter SR, Gripentrog AP. 1989. Effect of sampling frequency on intersample variance and food consumption estimates of non-piscivorous largemouth bass. Trans Am Fish Soc. 118(1):11–19.
- Hodgson JR, Cochran PA. 1988. The effect of sample methodology on diet analysis in largemouth bass (Micropterus salmoides). Verh Int Verein Limnol. 23(3):1670–1675.
- Hodgson JR, Hansen EM. 2005. Terrestrial prey items in the diet of largemouth bass, Micropterus salmoides, in a small north temperate lake. J Freshw Ecol. 20(4):793–794.
- Hodgson JR, He X, Kitchell JF. 1993. The fish populations. In: Carpenter SR, Kitchell JF, editors. The trophic cascade in lakes. New York (NY): Cambridge University Press; p. 43–68.
- Hodgson JR, Hodgson CJ, Hodgson JYS. 2008. Water mites in the diet of largemouth bass. J Freshw Ecol. 23(2):327–331.
- Hodgson JR, Kitchell JF. 1987. Opportunistic foraging by largemouth bass (Micropterus salmoides). Am Midl Nat. 118(2):323–336.
- Hodgson JR, Schindler DE, He X. 1998. Homing tendency of three piscivorous fishes in a northern temperate lake. Trans Am Fish Soc. 127:1080–1083.
- Hodgson JY, Hodgson JR. 2000. Exploring optimal foraging by largemouth bass (Micropterus salmoides) from three experimental lakes. Verh Int Verein Limnol. 27(4):1757–1762.
- Hoyle JA, Keast A. 1987. The effect of prey morphology and size on handling time in a piscivore, the largemouth bass (Micropterus salmoides). Can J Zool. 65:972–1977.
- Hughes RN, editor. 1990. Behavioral mechanisms of food selection. New York: Springer-Verlag; p. 886.
- Hughes RN, Kaiser MJ, Mackney PA, Warburton K. 1992. Optimizing foraging behavior through learning. J Fish Biol. 41(sb):77–91.
- Johnsson JI. 1997. Individual recognition affects aggression and dominance relations in rainbow trout, Oncorhynchus mykiss. Ethology. 103(4):267–282.
- Kamil AC, Krebs JR, Pulliam HR, editors. 1987. Foraging behavior. New York (NY): Plenum Press; 676 pp.
- Keast A. 1985. The piscivore feeding guild of fishes in small freshwater ecosystems. Environ Biol Fish. 12(2):119–129.
- Kerfoot WC, Kellogg DL, Strickler JR. 1980. Visual observations of live zooplankters: evasion, escape, and chemical defenses. In: Kerfoot WC, editor. Evolution and ecology of zooplankton communities. Hanover (NH): University Press of New England; p. 10–27.
- Kozlowski J. 1996. Optimal allocation of resources explains interspecific life history patterns in animals with indeterminate growth. Proc R Soc Lond B. 263:559–566.
- Kramer RH, Smith LL. 1962. Formation of year classes in largemouth bass. Trans Am Fish Soc. 91(1):29–41.
- Kruppert S, Deussen L, Weiss LC, Horstmann M, Wolff JO, Kleinteich T, Gorb SN, Tollrian R. 2019. Zooplankters’ nightmare: the fast and efficient catching basket of larval phantom midges (Diptera: Chaoborus)). PLoS One. 14(3):e0214013.
- Leavitt PR, Carpenter SR, Kitchell JF. 1989. Whole-lake experiments: the annual record of fossil pigments and zooplankton. Limnol Oceanogr. 34(4):700–717.
- Li TL, Wetterer JK, Hairston NG Jr. 1985. Fish size, visual resolution, and prey selectivity. Ecology. 6:1175–1729.
- Lomnicki A. 1988. Population biology of individuals. Princeton (NJ): Princeton University Press; 240 pp.
- Ludsin SA, DeVries DR. 1997. First-year recruitment of largemouth bass: the interdependency of early life stages. Ecol Appl. 7(3):1024–1038.
- Milinski M. 1984. Competitive resource sharing: an experimental test of a learning rule for ESSs. Anim Behav. 32:901–911.
- Mittelbach GG, Persson L. 1998. The ontogeny of piscivory and its ecological consequences. Can J Fish Aquat Sci. 55(6):1454–1465.
- Moyle P, Cech JJ. 1996. Fishes: an introduction to ichthyology. 3rd ed. Englewood Cliffs (NJ): Prentice-Hall; p. 590.
- Nisbet RM, Gurney WSC, Murdoch WW, McCauley E. 1989. Structured population models: a tool for linking effects at individual and population level. Biol J Linnean Soc. 37(1–2):79–99.
- Nyberg DW. 1971. Prey capture in largemouth bass. Am Midl Nat. 86(1):128–144.
- O’Brien WJ, Slade NA, Vinyard GL. 1976. Apparent size as the determinant of prey selection by bluegill sunfish (Lepomis macrochirus). Ecology. 57(6):1304–1310.
- Olson MH. 1996. Ontological niche shifts in largemouth bass: variability and consequences for first-year growth. Ecology. 77(1):179–190.
- Osenberg CW, Olson MH, Mittelbach GG. 1994. Stage structure in fishes: resource productivity and competition gradients. In: Stouder DJ, Fresh KL, Feller RJ, editors. Theory and application in fish feeding ecology. Columbia (SC): University of South Carolina Press; p. 151–170.
- Post DM. 2003. Individual variation in the timing of ontogenetic niche shifts in largemouth bass. Ecology. 84(5):1298–1310.
- Post DM, Carpenter SR, Christensen DL, Cottingham KL, Kitchell JF, Hodgson JR, Schindler DE. 1997. Seasonal effects of variable recruitment of a dominant piscivore on pelagic food web structure. Limnol Oceanogr. 42(4):722–729.
- Post DM, Kitchell JF, Hodgson JR. 1998. Interaction among adult demography, spawning date, growth rate, predation, overwinter mortality, and the recruitment of largemouth bass in a northern lake. Can J Fish Aquat Sci. 55(12):2588–2600.
- Purdom T, Schoofs A, Hodgson JR, Maki T, Hodgson JY. 2015. A thirty year diet record of largemouth bass (Micropterus salmoides) from a small north temperate lake. Bios. 86(1):20–30.
- Regelemann K. 1984. Competitive resource sharing: a simulation model. Anim Behav. 32:226–232.
- Riessen HP. 1999. Chaoborus predation and delayed reproduction in Daphnia: a demographic modeling approach. Evol Ecol. 13(4):339–363.
- Rick AR, Hodgson JR, Seekell DA. 2011. Foraging specialization by the opportunistic largemouth bass (Micropterus salmoides). J Freshw Ecol. 26(3):435–439.
- Ricker WE. 1975. Computation and interpretation of biological statistics of fish populations (Fisheries Research Board of Canada, Ottawa Bulletin). Ottawa: Department of the Environment, Fisheries and Marine Service; p. 191.
- Rollo CE, Hawryluk MD. 1988. Compensatory scope and resource allocation in two species of aquatic snails. Ecology. 69(1):146–156.
- Rooij JM, Bruggemann JH, Videler JJ, Breeman AM. 1995. Plastic growth of the herbivorous reef fish Sparisoma viride: field evidence for a trade-off between growth and reproduction. Mar Ecol Prog Ser. 122:93–105.
- Sammons SM, Maceina MJ. 2006. Changes in diet and food consumption of largemouth bass following largescale hydrilla reduction in Lake Seminole, Georgia. Hydrobiologia. 560(1):109–120.
- Schindler DE, Hodgson JR, Kitchell JF. 1997. Density-dependent changes in individual foraging specialization of largemouth bass. Oecologia. 110(4):592–600.
- Scott WB, Crossman EJ. 1973. Freshwater fishes of Canada. Fisheries Research Board of Canada Bulletin No. 184. Ottawa, Canada: Fisheries Research Board of Canada; 966 pp.
- Shelton WL, Davies WD, King TA, Timmons TJ. 1979. Variations in the growth of the initial year class of largemouth bass in West-Point-Reservoir, Alabama and Georgia. Trans Am Fish Soc. 108(2):142–149.
- Sissenwine MP. 1984. Why do fish populations vary? In: May RM, editor. Exploitation of marine communities. New York (NY): Springer-Verlag; p. 59–94.
- Soranno PA, Carpenter SR, Elser MM, 1993a. Zooplankton community dynamics. In: Carpenter SR, Kitchell JF, editors. The trophic cascade in lakes. New York (NY): Cambridge University Press; p. 116–152.
- Soranno PA, Carpenter SR, He X. 1993b. Zooplankton biomass and body size. In: Carpenter SR, Kitchell JF, editors. The trophic cascade in lakes. New York (NY): Cambridge University Press; p. 172–188.
- Soranno PA, Carpenter SR, Moegenburg SM. 1993c. Dynamics of the phantom midge: implications for zooplankton. In: Carpenter SR, Kitchell JF, editors. The trophic cascade in lakes. New York (NY): Cambridge University Press; p. 103–115.
- Swift MC, Forward RB. 1981. Chaoborus prey capture efficiency in the light and dark. Limnol Oceanogr. 26(3):461–466.
- Tonn WM, Magnuson JJ. 1982. Patterns in the species composition and richness of fish assemblages in northern Wisconsin Lakes. Ecology. 63(4):1149–1149.
- Tyler AV, Dunn RW. 1976. Ration, growth, and measures of somatic and organ condition in relation to meal frequency in winter flounder Pseudopleuronectes americanus, with hypotheses regarding population homeostasis. J Fish Res Board Can. 33(1):63–75.
- Wainwright PC, Day SW. 2007. The forces exerted by aquatic suction feeders on their prey. J R Soc Interface. 4(14):553–560.
- Ward AJW, Webster MM, Hart PJB. 2006. Intraspecific food competition in Fishes. Fish Fisheries. 7(4):231–261.
- Waters DS, Noble RL. 2004. Spawning season and nest fidelity of largemouth bass in a Tropical Reservoir. N Am J Fish Manage. 24(4):1240–1251.
- Werner EE. 1979. Niche partitioning by food size in fish communities. In: Stroud RH, Clepper AE, editors. Predator-prey systems in fisheries management. Washington, DC: Sport Fishing Institute; p. 311–322.
- Werner EE, Gilliam JF. 1984. The ontogenetic niche and species interactions in size structured populations. Annu Rev Ecol Syst. 15(1):393–425.
- Werner EE, Hall DJ. 1974. Optimal foraging and the size selection of prey by the bluegill sunfish (Lepomis macrochirus). Ecology. 55(5):1042–1052.
- Wetzel RG. 2001. Limnology: lake and river ecosystems. 3rd ed. San Diego (CA): Academic Press. p. 1006.