ABSTRACT
Purpose
Atropine has been proven to be effective in retarding myopia progression. However, the underlying mechanism remains unknown. Our purpose was to detect morphological and functional changes caused by atropine during myopic inhibition.
Method
Twenty 2-week-old guinea pigs were randomly assigned to either the saline group (n = 10) or the atropine group (n = 10). Form-deprived myopia (FDM) and intravitreal injections were applied on the right eyes. The injections were given every 3 days, lasting for 2 weeks. The left eyes served as control. Ocular refraction, axial length, retinal, and choroidal thickness were collected at the start and the end of the experiment. Retinal function was evaluated via full-field electroretinogram (ERG) at the end of treatment.
Results
The interocular differences (experimental eye minus control eye) of refraction error (RE), vitreous chamber depth (VCD), and axial length (AL) in the saline group were significantly greater than those in the atropine group (RE, VCD: P < .001, AL: P < .0001). The differences in choroidal thickness between the two groups did not reach statistical significance. However, a decreasing trend of choroidal thickness was observed in the saline group but not in the atropine group. Furthermore, the interocular differences of total retinal and outer retinal thickness in the atropine group were much thicker than in the saline group (P < .001 and P < .01, respectively). The treatment did not affect inner retinal thickness. In photopic ERG, the atropine-treated FDM eyes showed significantly greater a-wave amplitudes compared to the saline group.
Conclusion
During the process of inhibiting FDM, atropine showed an effect on the outer retina, most likely on the cones, in guinea pigs.
Introduction
In recent decades, myopic prevalence has increased dramatically, especially in East Asia, where more than 80% of adolescents aged 17–18 are myopic,Citation1 giving rise to a parallelly increasing prevalence of high myopia,Citation2 which may induce blindness. Therefore, preventing myopia progression has become imperative for public health. Currently, the emerging control methods for myopia include optical, surgical, behavioural, and pharmaceutical interventions. Among those options, atropine is the most effective treatment in retarding myopic shift and axial length elongation, working in a dose-dependent manner.Citation3 When assessing axial length (AL), which is more reliable than refraction error (RE), the efficacy of 0.05% atropine over 1 year was 53%.Citation4
Although numerous clinical trials have proved the efficacy of atropine,Citation5 the underlying mechanism remains unclear. Most research in this area has focused on biomolecules and signal pathways relating to atropine. Injections of atropine into the eyes of a chicken increased vitreal dopamine concentrations temporarily and stimulated retinal activity markers ZENK and c-Fos in the inner nuclear layer.Citation6 Atropine also reduced the levels of retinal GABA transporter 1 (GAT-1), which was elevated in mouse lens-induced myopic (LIM) eyes.Citation7 Atropine inhibited the expression and secretion of transforming growth factor (TGF-β2), which is involved in regulating scleral remodeling within the retinal pigment epithelium (RPE) cells in vitro.Citation8 Expressions of scleral anti-remodeling and circadian rhythm-related genes were altered by atropine treatment in human scleral fibroblasts in vitro, detected via next-generation sequencing.Citation9 In addition to these biomolecular studies, other research elucidated that choroidal thickness (ChT) was slightly increased in chicken LIM eyes by atropine.Citation10 Meanwhile, in chicken form-deprived myopic (FDM) eyes, atropine thickened the scleral fibrous layer and thinned the cartilaginous scleral layer, reversing the typical changes induced by myopia.Citation11
Nonetheless, few studies have illustrated retinal functional and morphologic changes during the process of myopic inhibition mediated by atropine. Safal Khanal et al.Citation12 recorded global flash multifocal electroretinogram responses to hyperopic/myopic defocus in healthy adults before and 24 h after the instillation of 1 drop of atropine. They found that atropine enhanced the activity of the inner layers of the peripheral retina under myopia defocus, while no such effect was detected under hyperopic defocusing.
This study investigated the functional and morphologic changes in the retina induced by atropine on a guinea pig FDM model, aiming to provide insight into how atropine retards myopic progression.
Materials and methods
Animal treatments
This research was approved by the Ethics Committee at Aier Eye Hospital Group. Two-week-old, pigmented guinea pigs (Cavia porcellus, the English short-hair stock) were purchased from Hunan Taiping Biotechnology Co. Ltd. and raised in an animal facility in Central South University. The illuminance was approximately 300 lux, under a daily 12:12 h light/dark cycle. The room temperature was maintained at 25°C. Food and water were provided ad libitum, with fresh vegetables supplied twice a day. According to the ARVO statement for the Use of Animals in Ophthalmic and Vision Research, all care and treatment of animals were carried out.
Form-deprivation myopia was induced for 2 weeks in all guinea pigs. Opaque balloon hull facemasks occluded the right eyes, with the left eyes serving as an internal control. The facemasks were checked twice a day and renewed if necessary. We randomly assigned the guinea pigs into the atropine group (n = 10) or saline group (n = 10). Intravitreal injections were performed simultaneously with patching. To avoid the interference of diurnal rhythm, injections were performed at the same time (from 10:00 to 11:00 a.m.) every 3 days for 2 weeks.Citation13 The guinea pigs were anaesthetized when intraperitoneal injections occurred using pentobarbital sodium (50 mg/kg, Sigma, St. Louis, MO, USA), with additional topical anesthesia provided by 0.5% proparacaine hydrochloride (Alcon, Puurs, Belgium). After removing the face mask and rinsing the conjunctival sac with chloramphenicol eye drops, the needle pierced into the vitreous chamber at 1 mm posterior to the ora serrata. The atropine group was injected with 5 µL of freshly prepared 2% atropine each time (100 µg, R003239, RHAWN, Shanghai, China), dissolved in 0.9% saline, via micro-syringe (32 G, 7654–01, Hamilton, Switzerland). The saline group was injected with 0.9% saline equivalent. The conjunctival sac was filled with erythromycin ointment after the injection, and the facemask was replaced quickly. During injections, eyes were restricted from any visual stimuli for less than 30 s.
Refraction and ocular biometric measurements
Ocular biometrics were measured before and after the treatment without cycloplegia. Refractive errors were measured without cycloplegia using eccentric infrared photoretinoscopy in hand-held awake animals.Citation14 Measurements were repeated 5 times; values were averaged and recorded as final refractive error. Animals with refractive error of either eye less than +1.00 D or anisometropia more than 1.00 D were excluded from the study at baseline.
The eyes’ axial dimensions were measured via A-scan ultrasonography (Axis Nano, Quantel, France) as previously described.Citation15 Topical anesthesia was administered prior to the measurements. A rubber tube filled with distilled water was attached to the probe to improve resolution for the guinea pig eye. The adjusted tip was moistened with water before touching the cornea gently. The tip was moved delicately to align the tube in the axis of the transducer. Vitreous chamber depth and axial length were recorded. The final values for each eye were the average of three repeated measurements.
Optical Coherence Tomography (OCT)
Retinal and choroidal thickness were evaluated at the start and end of the experiment by optical coherence tomography (OCT, Micron IV, Phoenix Research Lab, Pleasanton, CA, USA) under anesthesia. Pupils were dilated by instillation of tropicamide phenylephrine (Mydrin-P, Santen, Suzhou, China). After using a sodium hyaluronate gel to protect the cornea surface, the guinea pig ocular fundus was monitored with a contact lens designed for rats. The parameters for image control, such as contrast and brightness, were adjusted during the measurement to improve the quality of images. At least four images acquired horizontally near the optic disc were applied for analysis for each eye.
Images were then analyzed through a semi-automated segmentation algorithm (Insight software, Phoenix Research Lab) to obtain the total retinal, outer retinal, and choroidal thickness. The retinal thickness was defined as the distance from the inner retinal boundary to the RPE layer. The outer retinal thickness was the length between the outer plexiform layer and the RPE layer. The inner retinal layer thickness was obtained by subtracting the retinal thickness from the outer retinal thickness. The choroidal thickness was measured from the RPE layer to the outer choroidal border. The borderlines were automatically recognized by Insight software program and then rectified manually if necessary, from which the raw data containing about 1000 evenly distributed points throughout each image were generated. The thickness was calculated by averaging the distance between borderlines at those points in raw data. As choroidal thickness varied with diurnal rhythm,Citation16 all the images were taken between 15:00 and 17:00.
Electroretinography (ERG)
At the end of the experiment, full-field flash ERGs were performed using Ganzfeld (Q450, Roland Consult, Wiesbaden, Germany) stimuli between 13:00 and 15:00 to assess retinal function. The animals were dark-adapted for at least 3 h, then placed on a 37°C-warming pad under anesthesia during the measurement to maintain body temperature. General anesthesia was achieved using pentobarbital sodium. The pupils were under dim red light. Gold wire loop electrodes were placed on the cornea wetted with saline binocularly as the active electrode. Needle references and ground electrodes were embedded under the skin of the cheek and buttocks, respectively.
Scotopic ERGs were performed using white LED stimuli without background lighting. Six intensities (0.01, 0.3, 1, 3, 10, and 30 cd·s/m2) were given to generate rod-dominant and mixed responses as the intensities increased. For intensities between 0.01 and 3 cd·s/m2, ERGs were averaged from 5 single flashes. The intervals for each intensity were 5, 30, 30, and 30 s. For intensities of 10 and 30 cd·s/m2, the responses to three single flashes were averaged. The intervals were 30 s and 50 s, respectively. The rods were saturated by being exposed to white light (25 cd·s/m2) for 10 minutes before the photopic ERG’s recordings. Five intensities (0.3, 1, 3, 10, 30 cd·s/m2) presented at 0.9 Hz were utilized to produce cone-dominant responses. ERGs were averaged from 50 flashes for each intensity. Both scotopic and photopic ERGs signals were band-pass-filtered between 1 and 300 Hz for a- and b-waves. The amplitude of the a-wave was measured from the baseline to the trough, and the peak-time was measured from the stimulus onset to the trough of the a-wave. The amplitude of the b-wave was measured from the trough of the a-wave to the peak of the b-wave and the peak-time was from stimulus onset to the peak of the b-wave.
Statistical analysis
Data analyses were performed using GraphPad Prism 8 (GraphPad Software, La Jolla, CA, USA). The interocular differences were derived from the experimental eyes versus the control eyes. All the data were reported as the mean ± SEM. The normal distributions of the data were checked with a Kolmogorov–Smirnov test. A two-tailed paired t-test was applied to compare the differences between the treated and the control eyes. A two-way mixed ANOVA with a Bonferroni post hoc test was applied to compare the intergroup differences after the treatment. The longitudinal and ERG differences were analyzed using two-way repeated measures ANOVA. The differences were defined to be significant at P < .05.
Results
Effects of atropine on refractive error and ocular biometrics
The primary parameters are listed in , which displayed data at baseline, after the treatment, and the changes induced by the treatment. No significant differences were observed in refractive error and ocular biometrics between the two groups at baseline (between the FDM eyes: RE: P = .09, VCD: P = .21, AL: P = .17; between the fellow eyes: RE: P = .12, VCD: P = .28, AL: P = .17, unpaired t-test). In line with previous studies, the saline-treated FDM eyes showed a distinct myopic shift, which manifested as significant increases at the end of the treatment in the refractive error (RE), vitreous chamber depth (VCD), and axial length (AL) compared to the control eyes (RE: P = .003; VCD: P < .001; AL: P < .001, paired t-test). Atropine treatment attenuated the myopic shift within FDM eyes. The changes in refractive error and vitreous chamber depth were not statistically different from the control eyes (RE: P = .10, VCD: P = .92, paired t-test), while the changes in axial length were significantly smaller in the atropine-treated FDM eyes (P = .02, paired t-test). The relatively shorter axial length was derived from a shallower anterior chamber depth and thinner lens thickness, although the differences were not statistically significant compared to the control eyes (see data in supplementary material).
Table 1. Data of refraction and ocular biometrics in the two groups (mean ± SEM)
After 2 weeks of treatment, atropine significantly reduced the interocular differences in RE compared to the saline group (F1,18 = 8.449, −0.71 ± 0.42 D vs. −2.84 ± 0.57 D, P < .01, , two-way mixed ANOVA), as well as in VCD (F1,18 = 13.48, 0.01 ± 0.04 mm vs. 0.18 ± 0.03 mm, P < .01, , two-way mixed ANOVA) and the AL measurements (F1,18 = 28.09, −0.09 ± 0.03 mm vs. 0.24 ± 0.05 mm, P < .0001, , two-way mixed ANOVA). The interocular differences in ocular biometrics were increased after the treatment in the saline group compared with baseline (RE: P < .001; VCD: P < .001; AL: P < .0001, two-way repeated ANOVA). Meanwhile the interocular differences in the atropine group were not altered after the treatment. These results suggest that intravitreal injection of atropine partially prevented myopic progression induced by deprivation. A regression analysis on the interocular differences of animals at week 2 revealed a significant linear correlation between RE and VCD (r2 = 0.59, P < .0001, ), and between RE and AL (r2 = 0.56, P < .0001, ), indicating that the myopia model in this study approximated axial myopia.
Figure 1. Interocular differences in RE (a), VCD (b), and AL (c) Before and after form-deprivation treatment in the saline and the atropine groups (mean ± SEM, experimental – fellow). The myopic progression in FDM eyes was significantly retarded in the atropine group. Interocular differences after the treatment were plotted, showing a significant linear correlation between RE and VCD (d), and between RE and AL (e). (a–c) Two-way mixed ANOVA with a Bonferroni post hoc test: **: P < .01; ***: P < .001; ****: P < .0001; D -E: linear regression analysis, n = 10. RE: refractive error, VCD: vitreous chamber depth, AL: axial length
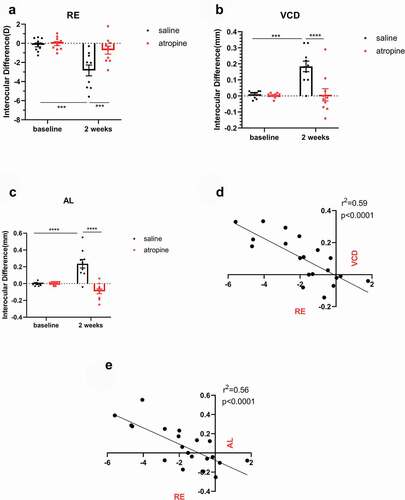
Effects of atropine on retinal and choroidal thickness
The data of retinal and choroidal thickness before and after treatment are shown in . Although a distinct myopic shift was observed, the retinal and outer retinal thickness of the saline-treated FDM eyes were comparable with the treated eyes (retinal thickness: P = .87, outer retinal thickness: P = .97, paired t-test). In contrast, the change in thicknesses in the atropine-treated FDM eyes were significantly greater than in the control eyes (retinal thickness: P = .007, outer retinal thickness: P = .008, paired t-test). Although the thickness of the inner retina demonstrated an increasing trend within the atropine-treated eyes, the differences were not significant. The inner retinal thickness changes in the two groups were close, with no statistical significance found in between-group nor contralateral comparisons. The choroidal thickness of the FDM eyes decreased within the saline-treated group; however, the difference of change was not statistically significant compared to the control eyes (P = .06, paired t-test). The change in choroidal thickness of the atropine-treated FDM eyes was similar to the fellow eyes (P = .73, paired t-test).
Table 2. Data of retinal and choroidal thickness in the two groups (mean ± SEM)
The comparisons of interocular differences in retinal and choroidal thicknesses are shown in . The interocular differences of the retinal thickness in the atropine group increased significantly compared with the saline group after treatment (F1,12 = 11.93, saline vs. atropine: 0.66 ± 2.32 vs. 9.42 ± 1.58 µm, P < .01, two-way mixed ANOVA). The outer retinal thickness change trend was similar to the retinal thickness. The interocular differences of the outer retinal thickness increased significantly in the atropine group at the end of the experiment (F1,12 = 12.97, saline vs. atropine: 0.21 ± 1.46 µm, 7.12 ± 1.49 µm, P < .01, two-way mixed ANOVA, ). Meanwhile, the inner retinal thickness was not affected by treatment (F1,12 = 0.04, saline vs. atropine: 0.46 ± 1.59 µm, 2.30 ± 1.68 µm, P = .44, two-way mixed ANOVA, ), indicating that the differences of retinal thickness were mainly derived from the outer retinal thickness change.
Figure 2. Interocular differences in retinal thickness (a) Outer retinal thickness, ORT, (b) Inner retinal thickness, IRT (c), and choroidal thickness, ChT (d) before and after treatment in the saline and atropine groups (mean ± SEM, experimental – fellow). The retinal thickness (a) and the outer retinal thickness (b) were increased with the treatment of atropine, while they were unaffected in the saline group. The inner retinal thickness showed no difference between the two groups (c). There was a decreasing trend for ChT in the saline-treated FDM eyes, yet the trend was diminished by atropine treatment (d). Two-way mixed ANOVA with a Bonferroni post hoc test: **: P < .01; ***: P < .001, n = 7
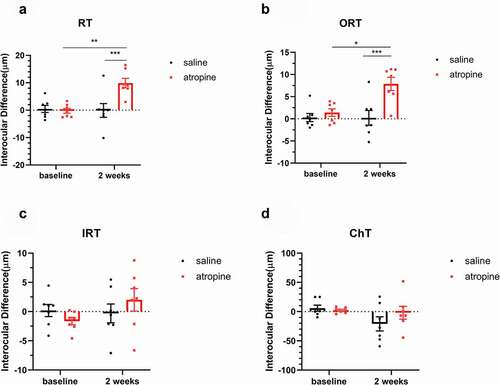
The interocular differences of ChT was not affected by the treatments (F1,12 = 0.73, P = .41, two-way mixed ANOVA). A decreasing trend of interocular difference of ChT in the saline group was observed, whereas the difference did not reach statistical significance (baseline vs. week 2: 5.89 ± 5.22 µm, −20.99 ± 12.11 µm, P = .08, two-way repeated ANOVA, ). In contrast, atropine inhibited the decrease of choroidal thicknesses (baseline vs. week 2: 2.30 ± 1.77 µm, −1.91 ± 10.96 µm, P > .99, two-way repeated ANOVA, ).
Effects of atropine on retinal function
The ERG curve of one representative guinea pig from each group is shown in . The mean scotopic and photopic a-wave amplitudes of the FDM eyes in the two groups are shown in . In photopic and scotopic ERG, the a-wave amplitudes in the saline-treated FDM eyes show a declining trend when compared to the control eyes. However, the differences were not statistically significant (). However, the amplitudes of atropine-treated FDM eyes were close to and even higher than the control eyes under 1 and 3 cd·s/m2 in the photopic ERG (atropine-treated FDM vs. fellow eyes: 6.60 ± 0.78 µV vs. 5.30 ± 0.72 µV under 1 cd·s/m2, P = .04; 11.84 ± 1.16 µV vs. 8.96 ± 0.86 µV under 3 cd·s/m2, P = .03, paired t-test, ). Moreover, the a-wave amplitudes in the atropine-treated FDM eyes were significantly higher than those of the saline-treated FDM eyes under the stimulation of 10 and 30 cd·s/m2 in the photopic ERG (F1,14 = 16.62, P = .001, two-way repeated ANOVA). Under 10 and 30 cd·s/m2, the a-wave amplitudes of the saline- and atropine-treated FDM eyes were 13.70 ± 1.15 µV vs. 19.46 ± 2.12 µV, P = .03; 15.93 ± 1.57 µV vs. 23.64 ± 2.18 µV, P = .01, respectively ().
Figure 3. ERG wave of a representative guinea pig from the saline group (left) and the atropine group (right). The black curve indicates the FDM eye, while the red curve indicates the control eye
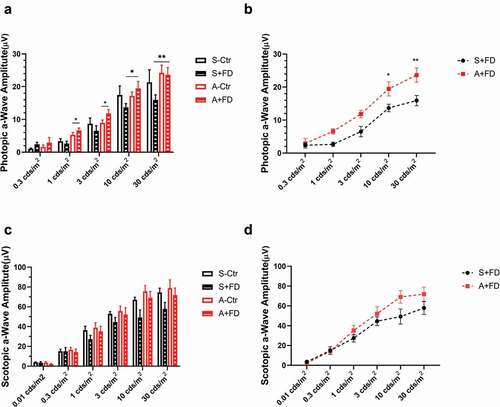
Figure 4. a-Wave amplitudes in the photopic (a), scotopic (c) adapted ERG. The comparison of the FDM eyes between two groups in the photopic (b), scotopic (d) adapted ERG (mean ± SEM). In the photopic ERG (a), the saline-treated FDM eyes show a declining trend in a-wave amplitudes compared to the control eyes. The differences were not statistically significant. The amplitudes in the atropine-treated FDM eyes were higher than the control eyes under the stimulation of 1 and 3 cd·s/m2 (A, paired t-test). The a-wave amplitudes increased significantly in the atropine-treated FDM eyes compared to the saline group under the stimulation of 10 and 30 cd·s/m2 (a and b, two-way repeated measures ANOVA with Bonferroni post hoc test). In the scotopic ERG, the a-wave amplitudes of atropine-treated FDM eyes tended to be higher than those of the saline-treated FDM eyes under higher stimulus intensity, but the differences were not significant (c,d): *: P < .05, **: P < .01, n = 8
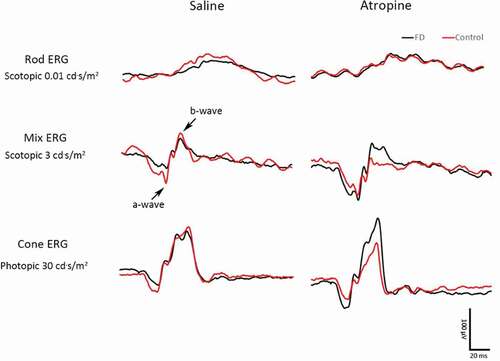
In the scotopic ERG, atropine increased in the a-wave amplitudes under higher stimulus intensity, however the differences were not significant (F1,14 = 1.992, P = .18, two-way repeated ANOVA, ). The b-wave amplitudes and the peak-times under each condition were not influenced by atropine treatment (data not shown).
Discussion
In this study, atropine effectively retarded the development of FDM in guinea pigs. Meanwhile, atropine increased the outer retinal thickness and a-wave amplitudes in photopic ERG, indicating that atropine may affect the function of the outer retina, most likely of the cones, during the inhibition of myopia.
Atropine inhibited FDM progression
Atropine has been proven to effectively suppress myopia caused by negative lenses,Citation7,Citation17 form deprivation,Citation18,Citation19 and flickering light.Citation20 Consistent with the existing conclusions, our study showed that atropine significantly inhibited the myopic shift and extra elongation of vitreous chamber depth. Moreover, the axial length of atropine-treated FDM eyes was shorter than that of the saline group. Diether et al.Citation21 found that an intravitreal injection of 2500 µg of atropine was a relatively high dosage into chicken LIM eyes producing a shorter axial length than in the control eyes. When imposing the negative lenses on the eyes after the cessation of the injections, only 50% of the chickens developed myopia. The author, therefore, speculated on the possible occurrence of retinal damage caused by high-dose atropine. In our study, structural and functional damage to the retina in atropine-treated eyes was ruled out by OCT and ERG. In a previous study, the injection of 70 µg atropine every 3 days, lasting for 4 weeks, also inhibited FDM in guinea pigs. However, the axial length of the deprived eyes was still longer than that of the control.Citation22 The treatment in our study was a higher dosage and a shorter duration. It was observed in a clinical study that the inhibition of the axial length induced by high-dose atropine was distinct in children during the first year, then weakened in the second year.Citation23 It is possible that the slow growth of the axial length in our study was a temporal effect derived from high-dose atropine.
It is worth noticing that the change in axial length in atropine-treated control eyes was greater than that in saline-treated control eyes. As the changes in vitreous chamber depth were nearly identical in both control groups, the discrepancy in axial length mainly resulted from anterior segment, including anterior chamber depth and lens thickness. Although of no statistical significance, the atropine-treated control eyes showed relatively deeper anterior chamber depth and thicker crystalline lens. This agrees with a previous study, in which the guinea pigs developed deeper anterior chamber depth and thicker lens in atropine-treated control eyes as well.Citation22 Goldberg et al. found that atropine increased lens thickness significantly in open chick eyes under illumination of 2 Hz luminance flicker, but not of blue/yellow color flicker nor steady light,Citation20 indicating that atropine could influence ocular anterior segment under certain stimulus. However, how atropine affected the untreated contralateral eyes was not clear. It is unlikely for the fellow eyes to acquire enough atropine through diffusion. One possible mechanism was the binocular neural linkage,Citation21 which requires further research.
In addition to the ocular biometrics, choroidal thickness is another indicator for myopia progression. Choroidal thicknesses correlated closely with axial length and refractive error in Chinese children and decreased early in myopia progression.Citation24 In our study, the choroidal thicknesses of FDM eyes in the saline group decreased but did not reach statistical significance (P = .05). It was found in previous studies that the vitreous chamber depth of guinea pigs elongated maximally,Citation25 and the choroidal thicknesses decreased significantlyCitation26 after being form-deprived for 4 weeks. Therefore, the reason for the marginal statistical significance in our study may be the shorter inducing duration. Compared with a noticeably decreasing trend in the saline group, the choroidal thicknesses of the FDM eyes in the atropine group was close to that of the control eyes, implying that atropine may prevent the choroid from thinning in myopic eyes. This deduction is in consistence with two recent studies. Xiangtian Zhou and his colleagues found that the decreases of choroidal thickness and choroidal blood perfusion in guinea pig FDM eyes were restrained by atropine.Citation27 The other evidence is that intravitreal injection of atropine into chick eyes under normal visual input increased the choroidal thickness temporarily, with a peak amplitude after 2 h.Citation28
Atropine increased outer retinal thickness
Outer retinal thickness correlates negatively with AL in highly myopic eyes.Citation29 The thinner outer retina is associated with decreasing retinal light sensitivity, indicating early visual field defects in highly myopic eyes.Citation30 In this study, the retinal and outer retinal thickness were not altered in saline injected FDM eyes, implying that the eyes deprived for 2 weeks were in the early stages of myopia, with an evident myopic shift but no obvious retinal structural damage. Our study found that the retinal and outer retinal thickness was increased by atropine, while the inner retinal thickness was not influenced. Hence, the thickening of the retinal thickness was derived from the outer retina, which may indicate an impact on the outer retina from atropine.
Inconsistently, a clinical trial in which myopic children were treated with 0.25% atropine for 1 year showed that the peripapillary retinal thickness was unchanged.Citation31 Considering that atropine affected neuronal responses merely on the peripheral but not on the central retina,Citation12 it is plausible that the peripheral retinal thickness in children might be influenced by atropine. Besides, an ARVO abstract states that the topical application of atropine for 6 weeks slowed the myopic progression and choroid thinning of the LIM model in guinea pigs but did not change the retinal thickness.Citation32 As for eye drops, less than 5% of the applied dose reached the intraocular tissues.Citation33 One drop of a 50 µL, 1% solution contains approximately 500 µg of atropine. Thus, less than 25 µg of atropine entered into the ocular, far less than the dose intravitreally injected in our study (100 µg). We assume that the different dose may be the main reason for the inconsistent outcome of the retinal thickness.
Atropine enhanced retinal function
The ERG amplitudes were reduced in myopic eyes, and latency was delayed, especially in excessively extended eyes.Citation34 In chicken FDM eyes, the a-wave amplitudes decreased, while the b-wave amplitudes were unaffected.Citation35 The responses from both waves were impaired by FDM in rats.Citation36 But neither of the waves were changed in mouse LIM.Citation37 The ERG responses of the FDM guinea pigs in our study were similar to those in chickens. Although a decreasing trend was observed, the a-wave amplitudes in the saline-treated FDM eyes were not statistically different from the control eyes. This could be evidence that the FDM eyes were at the early stage of myopia in our study.
In contrast with the saline group, the decreasing trend of ERG response was diminished by atropine. The a-wave amplitudes of atropine-treated eyes were significantly higher than those of the saline-treated eyes. It was found in a clinical trial that topical atropine did not prevent the gradual decline of cone function in myopic children.Citation38 However, when multifocal ERG was applied, an enhanced peripheral retinal response caused by atropine was recorded in adults.Citation12 This may be interpreted as full-field ERG covered the regional retina disparity. Thus, atropine might work on the peripheral rather than the central retina.
The increasing a-wave amplitudes in the atropine group were only significant in the photopic ERG with a stimulus intensity of 1 cd·s/m2, implying that the effect may be exerted mainly on the cones. Cones play an important role in emmetropization and myopia. The reduced amplitudes and delayed latencies in myopic subjects resulted primarily from cone function loss, especially in peripheral areas.Citation39 In a genetically modified mouse model of cone dysfunction, FDM induced a more severe myopic shift than in the wild-type counterparts.Citation40 This raised the possibility that high-dose atropine inhibits myopia by preserving the function of the cones.
However, the role of amacrine cells cannot be excluded with the lack of oscillatory potential during ERG testing in the present study. In a recent study, Khanal et al.Citation41 found that atropine reduced the oscillatory potential amplitudes in human, a sign of participation of amacrine cells which synthesize and release neurotransmitters important for retinal function. It is conceivable that introduction of atropine changed the activity of amacrine cells, hence altered the release of neurotransmitters, for instance, boosted release of dopamine.Citation6 Additionally, a delayed scotopic a-wave peak time without affecting amplitude induced by atropine was reported,Citation41 suggesting a role for rods. It is known that in chicken form-deprived eyes, the rod outer segments were elongated and “pushed” the retinal pigment epithelium layer.Citation42 Moreover, functional rods are critical to emmetropization and response to form deprivation in mice.Citation43 The effect of atropine on rods in myopia inhibition is worth further investigation.
Choroidal thicknesses and choroidal blood flow are highly correlated, with both decreasing in FDMM and LIM eyes and increasing during recovery.Citation44 In fact, the change of choroidal blood flow is prior to and more transient than choroidal thicknesses.Citation45 The responses from photoreceptors and RPE were maintained primarily by choroidal circulation.Citation46 As atropine halted the decreasing trend of choroidal thicknesses in FDM eyes, an enhanced retinal function may be an indirect impact from atropine. Atropine may also work directly on the retina as it altered the amounts of several retinal activity markers and neurons.Citation6
There are limitations in our study: (1) Lack of concentration and duration gradients. Hence, the conclusion only applies to a high-dose and short-period situation; (2) The outcomes of OCT and ERG applied only to the overall retina. However, the proportion and density of cones vary in different retinal quadrants in guinea pigs.Citation47 It would be necessary to distinguish the reactions from different retinal areas; (3) Our conclusion was derived from imaging and functional detection. Biological staining and scanning should be conducted to reveal the microstructural changes in a future study.
In conclusion, our results showed that the intravitreal injection of atropine thickened the outer retinal thickness and enhanced the photoreceptor responses in photopic ERG in the process of inhibition of FDM in guinea pigs, implying that atropine may have an impact on cones in inhibiting eye growth.
Supplemental Material
Download PDF (235.7 KB)Acknowledgments
The authors thank Dr Heping Xu for reviewing the manuscript and his helpful advice.
Disclosure statement
No potential conflict of interest was reported by the author(s).
Supplementary material
Supplemental data for this article can be accessed on the publisher’s website
Additional information
Funding
References
- Morgan IG, Ohno-Matsui K, Saw S-M. Myopia. Lancet (London, England). 2012;379(9827):1739–48. doi:https://doi.org/10.1016/S0140-6736(12)60272-4.
- Dong L, Kang YK, Li Y, Wei WB, Jonas JB. Prevalence and time trends of myopia in children and adolescents in China: a systemic review and meta-analysis. Retina. 2019;40(3):399–411. doi:https://doi.org/10.1097/IAE.0000000000002590.
- Huang J, Wen D, Wang Q, McAlinden C, Flitcroft I, Chen H, Saw SM, Chen H, Bao F, Zhao Y. Efficacy comparison of 16 interventions for myopia control in children: a network meta-analysis. Ophthalmology. 2016;123(4):697–708. doi:https://doi.org/10.1016/j.ophtha.2015.11.010.
- Yam JC, Jiang Y, Tang SM, Law AKP, Chan JJ, Wong E, Ko ST, Young AL, Tham CC, Chen LJ, et al. Low-concentration atropine for myopia progression (lamp) study: a randomized, double-blinded, placebo-controlled trial of 0.05%, 0.025%, and 0.01% atropine eye drops in myopia control. Ophthalmology. 2019;126(1):113–24. doi:https://doi.org/10.1016/j.ophtha.2018.05.029.
- Lin L, Lan W, Liao Y, Zhao F, Chen C, Yang Z. Treatment outcomes of myopic anisometropia with 1% atropine: a pilot study. Optom Vis Sci. 2013;90(12):1486–92. doi:https://doi.org/10.1097/OPX.0000000000000097.
- Mathis U, Feldkaemper M, Wang M, Schaeffel F. Studies on retinal mechanisms possibly related to myopia inhibition by atropine in the chicken. Graefes Arch Clin Exp Ophthalmol. 2020;258(2):319–33. doi:https://doi.org/10.1007/s00417-019-04573-y.
- Barathi VA, Chaurasia SS, Poidinger M, Koh SK, Tian D, Ho C, Iuvone PM, Beuerman RW, Zhou L. Involvement of gaba transporters in atropine treated myopic retina as revealed by itraq quantitative proteomics. J Proteome Res. 2014;13(11):4647–58. doi:https://doi.org/10.1021/pr500558y.
- Tan J, Deng Z-H, Liu S-Z, Wang J-T, Huang C. Tgf-beta2 in human retinal pigment epithelial cells: expression and secretion regulated by cholinergic signals in vitro. Curr Eye Res. 2010;35(1):37–44. doi:https://doi.org/10.3109/02713680903374190.
- Hsiao Y-T, Chang W-A, Kuo M-T, Lo J, Lin H-C, Yen M-C, Jian S-F, Chen Y-J, Kuo P-L. Systematic analysis of transcriptomic profile of the effects of low dose atropine treatment on scleral fibroblasts using next-generation sequencing and bioinformatics. Int J Med Sci. 2019;16(12):1652–67. doi:https://doi.org/10.7150/ijms.38571.
- Nickla DL, Zhu X, Wallman J. Effects of muscarinic agents on chick choroids in intact eyes and eyecups: evidence for a muscarinic mechanism in choroidal thinning. Ophthalmic Physiol Opt. 2013;33(3):245–56. doi:https://doi.org/10.1111/opo.12054.
- Gallego P, Martinez-Garcia C, Perez-Merino P, Ibares-Frias L, Mayo-Iscar A, Merayo-Lloves J. Scleral changes induced by atropine in chicks as an experimental model of myopia. Ophthalmic Physiol Opt. 2012;32(6):478–84. doi:https://doi.org/10.1111/j.1475-1313.2012.00940.x.
- Khanal S, Turnbull PRK, Lee N, Phillips JR. The effect of atropine on human global flash mferg responses to retinal defocus. Invest Ophthalmol Vis Sci. 2019;60(1):218–25. doi:https://doi.org/10.1167/iovs.18-24600.
- Nickla DL, Jordan K, Yang J, Singh P. Effects of time-of-day on inhibition of lens-induced myopia by quinpirole, pirenzepine and atropine in chicks. Exp Eye Res. 2019;181:5–14. doi:https://doi.org/10.1016/j.exer.2019.01.008.
- Schaeffel F, Hagel G, Eikermann J, Collett T. Lower-field myopia and astigmatism in amphibians and chickens. J Opt Soc Am A Opt Image Sci Vis. 1994;11(2):487–95. doi:https://doi.org/10.1364/JOSAA.11.000487.
- Schaeffel F, Howland HC. Properties of the feedback loops controlling eye growth and refractive state in the chicken. Vision Res. 1991;31(4):717–34. doi:https://doi.org/10.1016/0042-6989(91)90011-S.
- Nickla DL. Ocular diurnal rhythms and eye growth regulation: where we are 50 years after lauber. Exp Eye Res. 2013;114:25–34. doi:https://doi.org/10.1016/j.exer.2012.12.013.
- Schmid KL, Wildsoet CF. Inhibitory effects of apomorphine and atropine and their combination on myopia in chicks. Optom Vis Sci. 2004;81(2):137–47. doi:https://doi.org/10.1097/00006324-200402000-00012.
- Schwahn HN, Kaymak H, Schaeffel F. Effects of atropine on refractive development, dopamine release, and slow retinal potentials in the chick. Vis Neurosci. 2000;17(2):165–76. doi:https://doi.org/10.1017/S0952523800171184.
- Luft WA, Ming Y, Stell WK. Variable effects of previously untested muscarinic receptor antagonists on experimental myopia. Invest Ophthalmol Vis Sci. 2003;44(3):1330–38. doi:https://doi.org/10.1167/iovs.02-0796.
- Goldberg LA, Rucker FJ. Opposing effects of atropine and timolol on the color and luminance emmetropization mechanisms in chicks. Vision Res. 2016;122:1–11. doi:https://doi.org/10.1016/j.visres.2016.03.001.
- Diether S, Schaeffel F, Lambrou GN, Fritsch C, Trendelenburg AU. Effects of intravitreally and intraperitoneally injected atropine on two types of experimental myopia in chicken. Exp Eye Res. 2007;84(2):266–74. doi:https://doi.org/10.1016/j.exer.2006.09.019.
- Zou L, Liu R, Zhang X, Chu R, Dai J, Zhou H, Liu H. Upregulation of regulator of g-protein signaling 2 in the sclera of a form deprivation myopic animal model. Mol Vis. 2014;20:977–87.
- Chia A, Chua WH, Cheung YB, Wong WL, Lingham A, Fong A, Tan D. Atropine for the treatment of childhood myopia: safety and efficacy of 0.5%, 0.1%, and 0.01% doses (atropine for the treatment of myopia 2). Ophthalmology. 2012;119(2):347–54. doi:https://doi.org/10.1016/j.ophtha.2011.07.031.
- Jin P, Zou H, Zhu J, Xu X, Jin J, Chang TC, Lu L, Yuan H, Sun S, Yan B, et al. Choroidal and retinal thickness in children with different refractive status measured by swept-source optical coherence tomography. Am J Ophthalmol. 2016;168:164–76. doi:https://doi.org/10.1016/j.ajo.2016.05.008.
- Lu F, Zhou X, Zhao H, Wang R, Jia D, Jiang L, Xie R, Qu J. Axial myopia induced by a monocularly-deprived facemask in Guinea pigs: a non-invasive and effective model. Exp Eye Res. 2006;82(4):628–36. doi:https://doi.org/10.1016/j.exer.2005.09.001.
- Chen W, Zhang H, Zhang Y, Wang Q, Wang Y, and Li ZW. Relationship between aquaporin-1 protein expression and choroidal thickness during the recovery of form-deprivation myopia in Guinea pigs. Curr Eye Res. 2020; 45(6) :705–12. doi:https://doi.org/10.1080/02713683.2019.1689275.
- Zhou X, Zhang S, Zhang G, Chen Y, Lei Y, Xiang J, Xu R, Qu J, Zhou X. Increased choroidal blood perfusion can inhibit form deprivation myopia in Guinea pigs. Invest Ophthalmol Vis Sci. 2020;61(13):25. doi:https://doi.org/10.1167/iovs.61.13.25.
- Mathis U, Feldkaemper MP, Schaeffel F. Effects of single and repeated intravitreal applications of atropine on choroidal thickness in alert chickens. Ophthalmic Res. 2021;64(4):664–74. doi:https://doi.org/10.1159/000515755.
- Flores-Moreno I, Ruiz-Medrano J, Duker JS, Ruiz-Moreno JM. The relationship between retinal and choroidal thickness and visual acuity in highly myopic eyes. Br J Ophthalmol. 2013;97(8):1010–13. doi:https://doi.org/10.1136/bjophthalmol-2012-302836.
- Wu Q, Chen Q, Lin B, Huang S, Wang Y, Zhang L, Lin H, Wang J, Lu F, Shen M. Relationships among retinal/choroidal thickness, retinal microvascular network and visual field in high myopia. Acta Ophthalmol. 2020;98(6):e709–e714. doi:https://doi.org/10.1111/aos.14372.
- Chan LW, Hsieh YT, Hsu WC, Cheng HC, Shen EP. Optic disc parameters of myopic children with atropine treatment. Curr Eye Res. 2017;42(12):1614–19. doi:https://doi.org/10.1080/02713683.2017.1359846.
- Zhu Q, Goto S, Torres JA, Wildsoet C. 1% atropine slows down defocus-induced myopia in contact lens-wearing Guinea pigs. Invest Ophthalmol Vis Sci. 2020;61:3399–3399.
- Gaudana R, Ananthula HK, Parenky A, Mitra AK. Ocular drug delivery. AAPS J. 2010;12(3):348–60. doi:https://doi.org/10.1208/s12248-010-9183-3.
- Sachidanandam R, Ravi P, Sen P. Effect of axial length on full-field and multifocal electroretinograms. Clin Exp Optom. 2017;100(6):668–75. doi:https://doi.org/10.1111/cxo.12529.
- Fujikado T, Hosohata J, Omoto T. Erg of form deprivation myopia and drug induced ametropia in chicks. Curr Eye Res. 1996;15(1):79–86. doi:https://doi.org/10.3109/02713689609017614.
- Chen P, Xu L, Zhang J, Cai X, Yang Y, Yu J, Qiu J, Ge J, Yu K, Zhuang J. Up-regulation of sorcs1, an important sorting receptor, in the retina of a form-deprivation rat model. Cell Mol Neurobiol. 2020;40(3):395–405. doi:https://doi.org/10.1007/s10571-019-00740-1.
- Jiang X, Kurihara T, Kunimi H, Miyauchi M, Ikeda S-I, Mori K, Tsubota K, Torii H, Tsubota K. A highly efficient murine model of experimental myopia. Sci Rep. 2018;8(1):2026. doi:https://doi.org/10.1038/s41598-018-20272-w.
- Chia A, Li W, Tan D, Luu CD. Full-field electroretinogram findings in children in the atropine treatment for myopia (atom2) study. Doc Ophthalmol. 2013;126(3):177–86. doi:https://doi.org/10.1007/s10633-012-9372-8.
- Kawabata H, Adachi-Usami E. Multifocal electroretinogram in myopia. Invest Ophthalmol Vis Sci. 1997;38:2844–51.
- Chakraborty R, Yang V, Park HN, Landis EG, Dhakal S, Bergen MA, Iuvone PM, Pardue MT. Lack of cone mediated retinal function increases susceptibility to form-deprivation myopia in mice. Exp Eye Res. 2019;180:226–30. doi:https://doi.org/10.1016/j.exer.2018.12.021.
- Khanal S, Rathod SN, Phillips JR. The acute effect of atropine eye drops on the human full-field electroretinogram. Doc Ophthalmol. 2021;142(3):315–28. doi:https://doi.org/10.1007/s10633-020-09806-8.
- Liang H, Crewther DP, Crewther SG, Barila AM. A role for photoreceptor outer segments in the induction of deprivation myopia. Vision Res. 1995;35(9):1217–25. doi:https://doi.org/10.1016/0042-6989(94)00241-D.
- Park H, Jabbar SB, Tan CC, Sidhu CS, Abey J, Aseem F, Schmid G, Iuvone PM, Pardue MT. Visually-driven ocular growth in mice requires functional rod photoreceptors. Invest Ophthalmol Vis Sci. 2014;55(10):6272–79. doi:https://doi.org/10.1167/iovs.14-14648.
- Zhang S, Zhang G, Zhou X, Xu R, Wang S, Guan Z, Lu J, Srinivasalu N, Shen M, Jin Z, et al. Changes in choroidal thickness and choroidal blood perfusion in Guinea pig myopia. Invest Ophthalmol Vis Sci. 2019;60(8):3074–83. doi:https://doi.org/10.1167/iovs.18-26397.
- Fitzgerald ME, Wildsoet CF, Reiner A. Temporal relationship of choroidal blood flow and thickness changes during recovery from form deprivation myopia in chicks. Exp Eye Res. 2002;74(5):561–70. doi:https://doi.org/10.1006/exer.2002.1142.
- Fujino T, Hamasaki DI. The effect of occluding the retinal and choroidal circulations on the electroretinogram of monkeys. J Physiol. 1965;180(4):837–45. doi:https://doi.org/10.1113/jphysiol.1965.sp007734.
- Lindenau W, Kuhrt H, Ulbricht E, Korner K, Bringmann A, Reichenbach A. Cone-to-Muller cell ratio in the mammalian retina: a survey of seven mammals with different lifestyle. Exp Eye Res. 2019;181:38–48. doi:https://doi.org/10.1016/j.exer.2019.01.012.