Abstract
Purpose
Highly dynamic oxygen gradients occur within tumors that can result in a hypoxic response, contributing to tumor progression and metastasis. Evidence in uveal melanoma (UM) suggests an upregulated hypoxia response in some poor prognosis UM characterized by HIF1α signaling. We aimed to investigate the effects of exposure to hypoxia on tumor growth and dissemination in the chick embryo chorioallantoic membrane (CAM) model.
Methods
UM cell lines (MP41, 92.1, MP46, and OMM1) were grown in two-dimensional culture and pre-exposed to hypoxic (1% O2) conditions for 72 h. The effects of this hypoxia pre-conditioning on cell number and clonogenicity as compared with 21% O2 (“normoxia”) were investigated prior to transplantation of the cells onto the CAM. Nodule-forming efficiency (NFE), nodule size, and the presence/absence of tumor cell dissemination were determined macroscopically and histologically.
Results
Exposure of UM cell lines to hypoxia upregulated HIF1α expression compared to cells cultured in normoxia. A 72-h pre-exposure to hypoxia significantly reduced cell number and clonogenicity in the MP41 and OMM1 cell lines while it had little effect in 92.1 and MP46 cells. When 72-h hypoxia pre-conditioned cells were grown in three-dimensions on the CAM, a reduction in NFE and nodule size was observed when compared with normoxic UM cells. All nodules were composed of proliferating (Ki-67+) Melan-A + cells and displayed chick blood vessel recruitment. Spread of UM cells into the adjacent CAM was observed; however, dissemination to the chick liver was only seen with 92.1 cells grown under normoxia.
Conclusions
Hypoxia pre-conditioning does not appear to drive a metastatic phenotype in UM; however, further understanding of how oxygen dynamics within the tumor microenvironment regulates HIF1 signaling is needed to determine whether inhibitors of HIF signaling represent a therapeutic option in metastatic UM.
Introduction
Despite effective treatment of primary uveal melanoma (UM), metastatic spread of UM cells from the eye to the liver is the leading cause of death in patients with UM.Citation1 Metastases arise predominantly in tumors that have particular clinical, histological and genetic characteristics. Indeed, loss of one copy of chromosome 3 (i.e. monosomy 3), gains in 8q, BAP1 mutations/loss of nuclear BAP1 expression, and a class 2 gene signature are all strongly associated with a high metastatic risk.Citation2–4 Several biomarkers have been associated with the class 2 gene expression signature potentially providing further insight into the functional changes regulating this UM cell dissemination. One of these factors is hypoxia inducible factor 1α (HIF1α), a hallmark of tumor hypoxia.Citation5 It is well-established that hypoxia generates intra-tumoral oxygen gradients, contributing to tumor plasticity and heterogeneity, and in turn promoting a more aggressive and metastatic phenotype.Citation6,Citation7 Cycles of hypoxia-re-oxygenation occur in solid tumors, happening rapidly over minutes or more slowly over days.Citation8–10 In neuroblastoma, pre-conditioning for 3 days with hypoxia demonstrated that the cells do not have to be hypoxic anymore to exhibit pro-metastatic capabilities suggesting retention of a “memory” of prior hypoxic exposure.Citation11 These hypoxic microenvironments can lead to alterations in tumor vasculature and cancer cell differentiation. This is supported in UM, such that HIF1α expression is observed in spindle cells lining structures associated with vasculogenic mimicry (VM).Citation12 Furthermore, it is associated with a de-differentiated embryonic-like phenotype in class 2 UM,Citation5 which is consistent with HIF1α signaling and an upregulated hypoxia response noted in a subset of poor prognosis UM by The Cancer Genome Atlas study.Citation13
The chick embryo chorioallantoic membrane (CAM) model has been utilized previously for the study of angiogenesisCitation14 and has more recently established itself as a quick and economical xenograft model for cancer research.Citation15–17 We have previously demonstrated and reported the successful utilization of the CAM to grow UM cell lines as three-dimensional (3D) tumor nodules over a short period of time.Citation17 In this study, we expand on our previous work, to further investigate the role of hypoxic pre-conditioning in UM in the CAM model, and to understand how this affects tumor growth and spread.
Materials and methods
Cell culture
Four UM cell lines derived from primary (MP41, MP46, and 92.1) and metastatic (OMM1) UM were used in this study.Citation18–20 Cell lines were free from mycoplasma at the time of study and had been authenticated by STR profiling according to the details in https://web.expasy.org/cellosaurus. All UM cell lines were GFP-labelled using lentiviral transduction methods as previously described.Citation11 Cells were maintained in 25 cm2, 75 cm2, and 175 cm2 Nunc EasYFlasks (Thermo Fisher Scientific, Loughborough, UK) under normoxic conditions (37 °C, 5% CO2) in RPMI 1640 with GlutaMAX (Gibco, Thermo Fisher Scientific) supplemented with 10% fetal bovine serum (Labtech, East Sussex, UK) and 1% Penicillin/Streptomycin (Gibco, Thermo Fisher Scientific). Cells were passaged using 0.05% Trypsin-EDTA (Gibco, Thermo Fisher Scientific). UM cells were plated and grown to ˜60% confluence before incubation in normoxic or hypoxic conditions (1% O2 and 5% CO2; Don Whitley Scientific, Shipley, UK; Hypoxystation-H35).
Western blot
UM cell lines were incubated for varying times up to 96 h in normoxic and hypoxic conditions. HIF-1α accumulates under hypoxic conditions and on re-oxygenation is rapidly degraded. Degradation is mediated through hydroxylation of prolines 402 and 564. For this reason, we examined the expression of hydroxylated HIF-1α. Cells were lysed by adding 200 µL RIPA buffer (Merck Life Science UK Limited, Dorset, UK) containing protease inhibitor cocktail (Merck Life Science UK Limited) on ice for 30 min. Lysates were spun at 10 000 g for 5 min at 4 °C and the supernatant was retained for western blot. Protein quantification was undertaken using Pierce BCA protein assay kit (Thermo Fisher Scientific) and absorbance was measured using the Spark Multimode Microplate Reader (Tecan, Männedorf, Switzerland) at 562 nm. Proteins were separated by SDS-PAGE using Any kD™ Mini-PROTEAN® TGX pre-cast gels (Bio-Rad, Watford, UK) and transferred using the Trans-Blot Turbo Transfer System (Bio-Rad) onto 0.2 µM PVDF membranes (Bio-Rad). Membranes were blocked using Tris-Buffered Saline, 0.1% Tween (TBS-T) with 5% milk and incubated overnight at 4 °C with rabbit anti-Hydroxy-HIF-1α antibody (Cell Signaling Technology, London, UK) diluted 1:1000 with TBS-T, 5% milk. Membranes were washed and incubated with goat anti-rabbit HRP antibody (DAKO, Agilent, Cheshire, UK) for 1 h. Membranes were further washed and incubated with SuperSignal West Pico PLUS (Thermo Fisher Scientific) HRP substrate and bands visualized with the GeneGnome XRQ imaging system (SYNGENE, Cambridge, UK). β-actin 1:5000 (Merck Life Science UK Limited) in TBS-T, 5% milk and a goat anti-mouse HRP secondary antibody (DAKO) was used as loading control. MP41 cells treated with the proteasome inhibitor MG132 were used as a positive control.
Sulforhodamine B assay of cell number
UM cell lines were incubated for 72 h in normoxic and hypoxic conditions before harvesting and plating into flat bottom 96-well plates (Nunclon delta-treated; Thermo Fisher Scientific) at densities of either 4000 (MP41, 92.1, OMM1) or 8000 cells (MP46) per 100 µL/well. After plating, cells were incubated for up to 96 h in normoxic conditions. At 24-h time points, media was discarded and cells were fixed by adding 100 µL 10% Trichloroacetic acid (TCA) (Merck Life Science UK Limited) to each well and incubating at 4 °C for 1 h. TCA was discarded by applying running tap water and plates were allowed to air dry. 100 µL of 0.4% Sulforhodamine B (SRB) (Merck Life Science UK Limited) in 1% acetic acid was then added to each well and the plates incubated at room temperature for 1 h. SRB solution was discarded and wells underwent several washes with 1% acetic acid to remove excess dye before leaving the plates to dry. Stained cells were solubilized by adding 10 mM Tris buffer and rocking for 10 min. Absorbance was measured at 595 nm using Spark Multimode Microplate Reader (Tecan), and background absorbance at 690 nm was subtracted from these readings.
Clonogenic assay
UM cell lines were incubated for 72 h in normoxic and hypoxic conditions before harvesting and plating into six-well plates (Nunclon delta-treated; Thermo Fisher Scientific) at a cell density of 500 cells/well in 2 mL of media. Plates were incubated in normoxic conditions until colonies of ≥50 cells were visible (14–16 days). At this time point, both normoxic and hypoxic conditioned UM cells were fixed and stained with 1% Gentian violet (Merck Life Science UK Limited) in 70% ethanol at room temperature for 10 min. Gentian violet was discarded and excess dye removed by submerging plates in water. Colonies of ≥50 cells were counted using a stereomicroscope.
CAM implantation
CAM implantation was carried out as previously described.Citation17 In brief, fertilized chicken eggs were incubated at 37 °C and then “windowed” at embryonic day 3 (E3). UM cell lines incubated in either normoxic or hypoxic conditions for 72 h were harvested at E7, counted and spun to produce 10 µL aliquots containing 2 × 106 cells in media for implantation onto the CAM. Previously windowed eggs were opened and the surface of the CAM was dried by applying sections of sterilized lens cleaning tissue (Thermo Fisher Scientific). The “wet” cell pellets were gently pipetted onto the surface of the CAM, eggs were resealed and incubated for 7 days until E14. At E14 eggs were opened, examined using a M165 FC dissecting microscope (Leica Microsystems, Wetzlar, Germany) and GFP-expressing tumor nodules present on or beneath the surface of the CAM were photographed and measured. Embryos were then dissected for internal examination of metastasis. Nodules present on the CAM and livers of all embryos with CAM nodules were fixed in 10% Neutral Buffered Formalin (NBF) for subsequent processing, embedding and histological examination.
Histology & immunohistochemistry
Formalin fixed paraffin embedded (FFPE) tissues were cut at 4 µm and mounted on X-tra™ Adhesive slides (Leica Biosystems UK Ltd, Milton Keynes, UK). Hematoxylin and eosin (H&E) stains were performed to confirm presence of tumor nodules on the CAM. Immunohistochemistry (IHC) was performed using the Bond RXm Automated Stainer with the Bond polymer refine detection systems in either red or brown, according to the manufacturers’ instructions (Leica Biosystems UK Ltd). Tissue sections were stained for Melan-A (A103, 1;100, DAKO), Ki67 (MM1-L-CE, 1:200, Leica Biosystems) & α-SMA (1A4, 1:400, DAKO).
Statistics
Statistical analyses were performed using SPSS, version 27.0 (SPSS, Chicago, USA) and GraphPad Prism (GraphPad, San Diego, CA). Statistical significance was considered at p < .05.
Results
In our studies, UM cell lines exposed to 1% O2 for 72 h demonstrated expression of hydroxylated HIF1α, which was absent in cells cultured under normoxic conditions (Supplementary Figure 1). All experiments were thus performed with 72-h normoxic and hypoxic pre-incubated cells.
Pre-conditioning of cells in hypoxic conditions reduces cell number and colony formation
Three UM cell lines, MP41, MP46, and OMM1, demonstrated a significant reduction (p < .05) in cell number following a 72-h pre-exposure to 1% O2 and then growth in normoxia for up to 96 h as compared with cells cultured in normoxia alone. This was greatest for OMM1 and MP41 cells with 60% and 38% reduction at 96 h respectively. While for 92.1 cells, pre-exposure to hypoxia had no significant effect on cell number as compared with normoxia alone ().
Figure 1. Proliferation of UM cells exposed to hypoxic conditions for 72 h. UM cells were cultured in normoxic or hypoxic conditions for 72 h prior to harvesting and plating in 96 well plates. Subsequent changes in cell number in normoxic conditions was measured over 96 h. The graphs show data from a representative experiment n = 5 technical repeats (mean ± SEM). (A) MP41, (B) MP46, (C) 92.1, (D) OMM1. The table details the percentage reduction in cell number between hypoxic and normoxic conditions at 96 h (n = 3 biological repeats). Significant differences were determined by t-test.
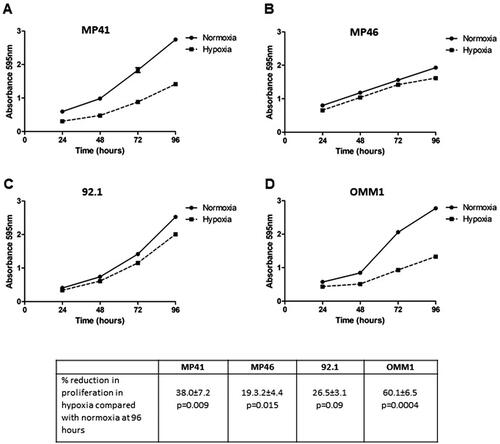
Hypoxia has previously been reported to re-programme cancer cells toward a cancer stem-like cell (CSC) phenotype.Citation21 To investigate whether pre-exposure to hypoxia may select for increased CSC populations in UM, we investigated clonogenicity of the UM cell lines following a 72-h pre-exposure to 1% O2 and then growth in normoxia, as compared with cells cultured in normoxia alone. Hypoxia significantly reduced clonogenicity in OMM1 (p < .0001) and MP41 (p < .0162) cells only ().
Figure 2. Clonogenicity of UM cells exposed to hypoxic conditions for 72 h. UM cells were cultured in normoxic or hypoxic conditions for 72 h prior to harvesting and plating in six well plates. Subsequent clonogenicity in normoxic conditions was measured. The graphs show data from a representative biological repeat (mean ± SEM, n = 6). (A) MP41, (B) MP46, (C) 92.1, and (D) OMM1. Significant differences were determined by t-test; ns: not significant, *p < .05, **p < .005, ***p < .0005.
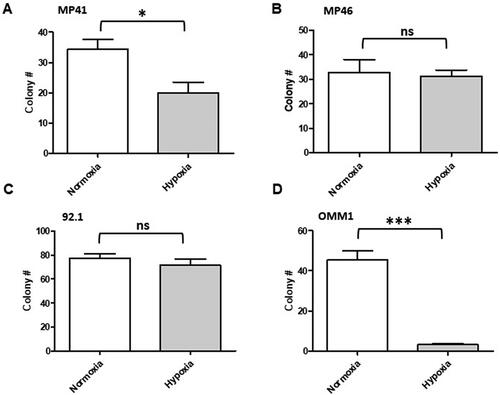
Pre-conditioning of cells in hypoxic conditions reduces nodule-forming efficiency and nodule size when UM cells are implanted on the CAM
Previous studies of neuroblastoma cells grown on the chick embryo CAM demonstrated that the metastatic phenotype can be controlled by neuroblastoma cell hypoxic pre-conditioning (3 days at 1% O2).Citation11 Given the effects of hypoxic pre-conditioning on colony forming efficiency and cell number, we investigated the metastatic phenotype of the UM cell lines when pre-exposed to hypoxic or normoxic conditions before transplantation onto the CAM. UM cell lines demonstrated variable tumor nodule-forming efficiencies (NFE) when transplanted onto the CAM as has been reported for cell lines of other cancer types (reviewed in Ref.Citation22) Under normoxic conditions, NFE was highest for the 92.1 cell line (55%) and lowest for the MP46 cell line (28%) (). This was affected by a 72-h pre-exposure of the cells to 1% O2 resulting in a reduction of NFE by around 50% in 92.1, OMM1 and MP41 UM cell lines. No nodules formed with MP46 cells following 72-h pre-exposure of the cells to 1% O2. However, the reduction in NFE by hypoxia failed to reach statistical significance (p < .05) in all UM cell lines examined (). With regard to tumor nodule size formed by normoxic UM cells on the CAM over the 7-day period, MP41 cells formed the largest nodules and OMM1 cells formed the smallest nodules (). For 92.1 and MP41 cells, when compared with normoxia, nodule size was reduced following 72-h pre-exposure of the cells to 1% O2, although this was not statistically significant likely due to the small number of nodules formed overall. OMM1 cells showed little change in nodule size following 72-h pre-exposure of the cells to 1% O2 as compared with normoxia.
Table 1. Nodule-forming efficiency and nodule size following the transplantation of hypoxic or normoxic pre-conditioned UM cells onto the CAM.
Macroscopic and histological features of UM nodules growing on the CAM
All UM nodules formed on the CAM stained positively with Melan-A and Ki67 demonstrating actively growing tumor cells (). αSMA positivity indicated recruitment of chick blood vessels into the tumor nodule, although metastatic spread to the chick liver was only observed in a single xenograft of 92.1 cells grown under normoxic conditions and transplanted on the chick embryo CAM (). Although tumor NFE and nodule size were reduced following pre-conditioning of UM cells in hypoxia, tumor nodules were similarly positive for Melan-A, Ki67 and retained recruitment of αSMA positive chick blood vessels. In a number of tumor nodules either grown in normoxic or hypoxic conditions, spread of the UM cells into the adjacent CAM tissues, a short distance from the xenograft site, could be seen ()).
Figure 3. Representative images of UM xenografts on the chick CAM harvested at E14. 92.1 (A and B) and MP41 (C and D) cells were cultured in normoxia (A and C) and hypoxia (B and D) for 72 h prior to harvesting and grafting onto the chick CAM. Nodules were removed and fixed in 10% NBF prior to histological and immunohistochemical examination. Top middle panels are H&E; bottom middle are Melan-A; top right are αSMA and bottom right are Ki67.
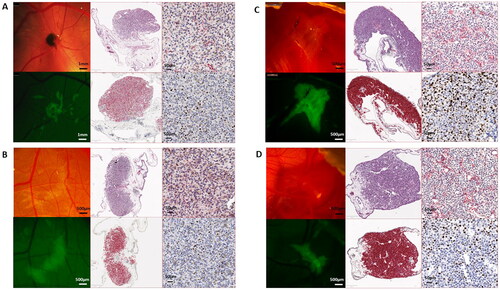
Discussion
In this study we have shown that pre-conditioning of UM cells with hypoxia for 72 h reduces cell number and clonogenicity in 2D culture, and that this is consistent with a reduced capacity of these cells to form tumor nodules on the CAM. There was no evidence in this study that hypoxia drives a metastatic phenotype. We provide further evidence to support the use of the chick embryo CAM model for the growth of 3D UM nodules.
Tumors experience a highly dynamic range of oxygen gradients.Citation23 As tumors grow in size, diffusion distances increase and there is localized outstripping of the blood supply, resulting in a mixed population of cells at differing oxygen tensions at any one time.Citation23 Hypoxia therefore, has been broadly separated into three types namely acute, chronic and cycling. In chronic hypoxia, cells experience low oxygen tensions for prolonged periods of time as tumors grow and outstrip the local blood supply while acute (short periods of time) and cycling (intermittent hypoxia) hypoxia occur as the tumor adapts to ongoing changes in the microenvironment, resulting in cellular responses, such as accumulation of reactive oxygen species, changes in metabolism, radioresistance and spontaneous metastasis.Citation10,Citation24 Transcriptional responses to hypoxia are primarily mediated by HIF with expression of the HIF1α subunit increasing dramatically when cells are exposed to low oxygen levels.Citation25 Previous studies have demonstrated that hypoxic pre-conditioning of neuroblastoma cells for 3 days drives a change to a more aggressive phenotype that is mediated by HIF1α and continues following cellular re-oxygenation.Citation11
High levels of HIFα have been observed in UM tissueCitation26,Citation27 and, indeed, increased HIF-1α expression was shown to be significantly associated with proliferative and vascular markers; although there was no correlation between HIF-1α expression levels and patient survival.Citation26,Citation27 Importantly in these studies, HIF1α expression was observed throughout the whole tumor and not necessarily restricted to hypoxic areas. In the study by Jha et al. HIF1α expression was significantly associated with highly pigmented UM cases.Citation26 It has been reported that melanin serves as a scavenger of oxygen and creates hypoxic conditions during its synthesis.Citation28 This has led to the suggestion by several authors that an oxygen-independent mechanism also regulates HIF1α expression, which is consistent with reports of HIF1α expression in UM cell lines grown under both normoxic and hypoxic conditions.Citation27,Citation29 In our study, however, HIFα expression was highest in UM cell lines cultured at 1% O2 as compared with cells grown at 21% O2, consistent with data from Hu et al. who also reported increased HIF1α when 92.1 and OMM1 cells were exposed to hypoxia for 24 h as compared with cells in normoxia.Citation30 We further demonstrated that OMM1 and MP41 cells showed the highest sensitivity in culture to hypoxic pre-exposure for 72 h with significant decreases in cell number and clonogenicity compared with cells grown in normoxia. El Filali et al. similarly demonstrated variable effects of hypoxia on proliferation in six UM cell lines exposed for up to 11 days.Citation31 Of note in these experiments was a significant reduction in the proliferation of 92.1 cells grown in hypoxia as compared with no effect in our studies using this cell line. This is likely due to chronic exposure to hypoxia in the studies by El FilaliCitation31 compared with acute hypoxic pre-conditioning in our experiments again highlighting the importance of understanding the contributions of acute, chronic and cycling hypoxia to tumor growth and metastasis.
When the UM cell lines were transplanted onto the chick embryo CAM, there was no significant effect of hypoxia on tumor nodule formation and size compared with normoxia for three of the cell lines; the MP46 cell line failed to form tumor nodules following hypoxia pre-conditioning. Importantly, a single metastatic lesion in the chick liver following the incubation of 92.1 cells in normoxia was identified throughout the experiments demonstrating spontaneous metastasis of these cells. We hypothesize that additional spontaneous metastatic lesions could be identified if longer incubation times were possible in this model, as we have previously reported metastatic foci in the chick liver by 92.1 and OMM1 cells when injected directly into the chick circulation.Citation17 Furthermore, although local invasion of UM cells into the CAM tissue was observed in some of the nodules formed for all UM cell lines, this was not dependent on oxygen concentrations. This is consistent with data to support the functional importance of HIF-1α signaling in promoting the invasive capacity of UM cells in both hypoxia and normoxia.Citation29 Interestingly, targeting hypoxia-induced gene expression with KCN1, a hypoxia inducible factor-1 (HIF-1) pathway inhibitor, has been shown to reduce tumor size and prolong survival in an orthotopic mouse model of UM using the 92.1 and Mel290 cell lines.Citation32 At the molecular level, KCN1 antagonized hypoxia-induced expression of two genes, P4HA1 and P4HA2, which regulate collagen maturation and deposition resulting in a disordered collagen VI structure, and reduced UM cell invasion. Similarly, Voropaev et al. demonstrated that shRNA knockdown of CREB or HIF-1 in Mel270 UM subcutaneous xenografts in SCID mice, dramatically decreased UM progression.Citation33
The use of the chick embryo CAM model is a strength of this study as it allows the UM cells to grow in 3D, with blood vessel recruitment and hence variations in oxygen tensions across the nodule, as compared with cells in 2D culture. A weakness of this study, however, is that it examined UM cell lines only. We have recently demonstrated the importance of the immune tumor microenvironment in metastatic UM,Citation34–36 and interestingly Bronkhorst et al. reported that conditioned media from cultured UM cell lines induced chemotaxis of monocytes, which was independent of the normoxic or hypoxic state.Citation37 We are currently working with 3D co-cultures of UM cells that incorporate immune infiltrates and other features of the tumor microenvironment of metastatic UM. The ability to xenograft such nodules onto the chick embryo CAM will enable more representative pre-clinical models for drug testing.
In conclusion, the chick embryo model continues to represent a valuable short-term animal model for pre-clinical cancer research. Further understanding of the role of differential hypoxia dynamics and HIF1 signaling in UM is needed to determine whether inhibitors of HIF signaling represent truly viable therapeutic options in this devastating disease.
Supplemental Material
Download JPEG Image (66.1 KB)Disclosure statement
No potential conflict of interest was reported by the author(s).
Data availability statement
The data supporting the findings of this study are available within the article and its supplementary materials.
Correction Statement
This article was originally published with errors, which have now been corrected in the online version. Please see Correction (http://dx.doi.org/10.1080/02713683.2023.2192394)
Additional information
Funding
References
- Carvajal RD, Schwartz GK, Tezel T, Marr B, Francis JH, Nathan PD. Metastatic disease from uveal melanoma: treatment options and future prospects. Br J Ophthalmol. 2017;101(1):38–44. doi:10.1136/bjophthalmol-2016-309034
- Eleuteri A, Taktak AFG, Coupland SE, Heimann H, Kalirai H, Damato B. Prognostication of metastatic death in uveal melanoma patients: a Markov multi-state model. Comput Biol Med. 2018;102:151–156. doi:10.1016/j.compbiomed.2018.09.024
- Onken MD, Worley LA, Ehlers JP, Harbour JW. Gene expression profiling in uveal melanoma reveals two molecular classes and predicts metastatic death. Cancer Res. 2004;64(20):7205–7209. doi:10.1158/0008-5472.CAN-04-1750
- Smit KN, Jager MJ, de Klein A, Kiliҫ E. Uveal melanoma: towards a molecular understanding. Prog Retin Eye Res. 2020;75:100800. doi:10.1016/j.preteyeres.2019.100800
- Chang SH, Worley LA, Onken MD, Harbour JW. Prognostic biomarkers in uveal melanoma: evidence for a stem cell-like phenotype associated with metastasis. Melanoma Res. 2008;18(3):191–200. doi:10.1097/CMR.0b013e3283005270
- Eales KL, Hollinshead KE, Tennant DA. Hypoxia and metabolic adaptation of cancer cells. Oncogenesis. 2016;5(1):e190. doi:10.1038/oncsis.2015.50
- Schito L, Semenza GL. Hypoxia-inducible factors: master regulators of cancer progression. Trends Cancer. 2016;2(12):758–770. doi:10.1016/j.trecan.2016.10.016
- Almendros I, Gozal D. Intermittent hypoxia and cancer: undesirable bed partners? Respir Physiol Neurobiol. 2018;256:79–86. doi:10.1016/j.resp.2017.08.008
- Martinive P, Defresne F, Bouzin C, Saliez J, Lair F, Grégoire V, Michiels C, Dessy C, Feron O. Preconditioning of the tumor vasculature and tumor cells by intermittent hypoxia: implications for anticancer therapies. Cancer Res. 2006;66(24):11736–11744. doi:10.1158/0008-5472.CAN-06-2056
- Saxena K, Jolly MK. Acute vs. chronic vs. cyclic hypoxia: their differential dynamics, molecular mechanisms, and effects on tumor progression. Biomolecules. 2019;9(8):339. doi:10.3390/biom9080339
- Herrmann A, Rice M, Lévy R, Pizer BL, Losty PD, Moss D, Sée V. Cellular memory of hypoxia elicits neuroblastoma metastasis and enables invasion by non-aggressive neighbouring cells. Oncogenesis. 2015;4(2):e138. doi:10.1038/oncsis.2014.52
- Folberg R, Hendrix MJ, Maniotis AJ. Vasculogenic mimicry and tumor angiogenesis. Am J Pathol. 2000;156(2):361–381. doi:10.1016/S0002-9440(10)64739-6
- Robertson AG, Shih J, Yau C, Gibb EA, Oba J, Mungall KL, Hess JM, Uzunangelov V, Walter V, Danilova L, et al. Integrative analysis identifies four molecular and clinical subsets in uveal melanoma. Cancer Cell. 2017;32(2):204.e15–220.e15. doi:10.1016/j.ccell.2017.07.003
- Ribatti D. Chicken chorioallantoic membrane angiogenesis model. Methods Mol Biol. 2012; 843:47–57. doi:10.1007/978-1-61779-523-7_5
- Kain KH, Miller JW, Jones-Paris CR, Thomason RT, Lewis JD, Bader DM, Barnett JV, Zijlstra A. The chick embryo as an expanding experimental model for cancer and cardiovascular research. Dev Dyn. 2014;243(2):216–228. doi:10.1002/dvdy.24093
- Deryugina EI, Quigley JP. Chick embryo chorioallantoic membrane model systems to study and visualize human tumor cell metastasis. Histochem Cell Biol. 2008;130(6):1119–1130. doi:10.1007/s00418-008-0536-2
- Kalirai H, Shahidipour H, Coupland SE, Luyten G. Use of the chick embryo model in uveal melanoma. Ocul Oncol Pathol. 2015;1(3):133–140. doi:10.1159/000370151
- Luyten GP, Naus NC, Mooy CM, Hagemeijer A, Kan-Mitchell J, Van Drunen E, Vuzevski V, De Jong PT, Luider TM. Establishment and characterization of primary and metastatic uveal melanoma cell lines. Int J Cancer. 1996;66(3):380–387. doi:10.1002/(SICI)1097-0215(19960503)66:3<380::AID-IJC19>3.0.CO;2-F
- Jager MJ, Magner JA, Ksander BR, Dubovy SR. Uveal melanoma cell lines: where do they come from? (An American Ophthalmological Society Thesis). Trans Am Ophthalmol Soc. 2016;114:T5.
- Amirouchene-Angelozzi N, Nemati F, Gentien D, Nicolas A, Dumont A, Carita G, Camonis J, Desjardins L, Cassoux N, Piperno-Neumann S, et al. Establishment of novel cell lines recapitulating the genetic landscape of uveal melanoma and preclinical validation of mTOR as a therapeutic target. Mol Oncol. 2014;8(8):1508–1520. doi:10.1016/j.molonc.2014.06.004
- Carnero A, Lleonart M. The hypoxic microenvironment: a determinant of cancer stem cell evolution. Bioessays. 2016;38 Suppl 1(Suppl 1):S65–S74. doi:10.1002/bies.201670911
- Chu PY, Koh AP, Antony J, Huang RY. Applications of the chick chorioallantoic membrane as an alternative model for cancer studies. Cells Tissues Organs. 2022; 211(2):222–237. doi:10.1159/000513039
- Campillo N, Falcones B, Otero J, Colina R, Gozal D, Navajas D, Farré R, Almendros I. Differential oxygenation in tumor microenvironment modulates macrophage and cancer cell crosstalk: novel experimental setting and proof of concept. Front Oncol. 2019;9:43.
- Muz B, de la Puente P, Azab F, Azab AK. The role of hypoxia in cancer progression, angiogenesis, metastasis, and resistance to therapy. Hypoxia. 2015;3:83–92. doi:10.2147/HP.S93413
- Semenza GL. HIF-1 and mechanisms of hypoxia sensing. Curr Opin Cell Biol. 2001;13(2):167–171. doi:10.1016/s0955-0674(00)00194-0
- Jha J, Singh MK, Singh L, Pushker N, Lomi N, Meel R, Chosdol K, Sen S, Bakhshi S, Kashyap S. Association of TYRP1 with hypoxia and its correlation with patient outcome in uveal melanoma. Clin Transl Oncol. 2021;23(9):1874–1884. doi:10.1007/s12094-021-02597-7
- Mouriaux F, Sanschagrin F, Diorio C, Landreville S, Comoz F, Petit E, Bernaudin M, Rousseau AP, Bergeron D, Morcos M. Increased HIF-1α expression correlates with cell proliferation and vascular markers CD31 and VEGF-A in uveal melanoma. Invest Ophthalmol Vis Sci. 2014;55(3):1277–1283. doi:10.1167/iovs.13-13345
- Brożyna AA, Jóźwicki W, Roszkowski K, Filipiak J, Slominski AT. Melanin content in melanoma metastases affects the outcome of radiotherapy. Oncotarget. 2016;7(14):17844–17853. doi:10.18632/oncotarget.7528
- Asnaghi L, Lin MH, Lim KS, Lim KJ, Tripathy A, Wendeborn M, Merbs SL, Handa JT, Sodhi A, Bar EE, Eberhart CG. Hypoxia promotes uveal melanoma invasion through enhanced Notch and MAPK activation. PLoS One. 2014;9(8):e105372. doi:10.1371/journal.pone.0105372
- Hu K, Babapoor-Farrokhran S, Rodrigues M, Deshpande M, Puchner B, Kashiwabuchi F, Hassan SJ, Asnaghi L, Handa JT, Merbs S, et al. Hypoxia-inducible factor 1 upregulation of both VEGF and ANGPTL4 is required to promote the angiogenic phenotype in uveal melanoma. Oncotarget. 2016;7(7):7816–7828. doi:10.18632/oncotarget.6868
- el Filali M, Missotten GS, Maat W, Ly LV, Luyten GP, van der Velden PA, Jager MJ. Regulation of VEGF-A in uveal melanoma. Invest Ophthalmol Vis Sci. 2010;51(5):2329–2337. doi:10.1167/iovs.09-4739
- Kaluz S, Zhang Q, Kuranaga Y, Yang H, Osuka S, Bhattacharya D, Devi NS, Mun J, Wang W, Zhang R, et al. Targeting HIF-activated collagen prolyl 4-hydroxylase expression disrupts collagen deposition and blocks primary and metastatic uveal melanoma growth. Oncogene. 2021;40(33):5182–5191. doi:10.1038/s41388-021-01919-x
- Voropaev H, Gimmelshein Vatkin M, Shneor D, Luski S, Honigman A, Frenkel S. Infectious knockdown of CREB and HIF-1 for the treatment of metastatic uveal melanoma. Cancers. 2019;11(8):1056. doi:10.3390/cancers11081056
- Figueiredo CR, Kalirai H, Sacco JJ, Azevedo RA, Duckworth A, Slupsky JR, Coulson JM, Coupland SE. Loss of BAP1 expression is associated with an immunosuppressive microenvironment in uveal melanoma, with implications for immunotherapy development. J Pathol. 2020;250(4):420–439. doi:10.1002/path.5384
- Krishna Y, Acha-Sagredo A, Sabat-Pośpiech D, Kipling N, Clarke K, Figueiredo CR, Kalirai H, Coupland SE. Transcriptome profiling reveals new insights into the immune microenvironment and upregulation of novel biomarkers in metastatic uveal melanoma. Cancers. 2020;12(10):2832. doi:10.3390/cancers12102832
- Krishna Y, McCarthy C, Kalirai H, Coupland SE. Inflammatory cell infiltrates in advanced metastatic uveal melanoma. Hum Pathol. 2017;66:159–166. doi:10.1016/j.humpath.2017.06.005
- Bronkhorst IH, Jehs TM, Dijkgraaf EM, Luyten GP, van der Velden PA, van der Burg SH, Jager MJ. Effect of hypoxic stress on migration and characteristics of monocytes in uveal melanoma. JAMA Ophthalmol. 2014 May;132(5):614–621. doi:10.1001/jamaophthalmol.2014.43