The Thermo Electron Model 5020 Sulfate Particulate Analyzer is a recently commercialized instrument that provides continuous measurements of the sulfate component of ambient particulate matter. The technique uses a stainless steel rod placed inside a quartz oven to reduce the particle sulfate to sulfur dioxide; followed by pulsed fluorescence detection of the sulfur dioxide. Field and laboratory evaluations of a pre-production version of the analyzer are described as well as laboratory evaluations of the pre-production version and two production units. Laboratory tests concentrated on challenging the instruments with ammonium sulfate aerosol, but tests with sodium, potassium, and calcium sulfate are reported as well. The instrument performed very well in field and laboratory settings, reporting values that were highly correlated with continuous mass measurements in the lab, and 24-hour filters in the field. Conversion/detection efficiencies for ammonium sulfate in the laboratory, and for ambient sulfate aerosol at our rural site in Addison, New York, were both very close to 80%. Laboratory conversion efficiencies for calcium, sodium, and potassium sulfate salts ranged from 4% to 63%. These lower efficiencies for mineral-type sulfates will be an important consideration in areas with significant concentrations of sea salt or mineral dust sulfate, and less important for the high sulfate Eastern US which is dominated by ammonium sulfate.
INTRODUCTION
Adverse health effects associated with exposure to fine particulate matter (PM2.5, atmospheric particles with aerodynamic diameter less than 2.5 micrometers) was the driving factor for the 1997 issuance of the revised fine particulate matter National Ambient Air Quality Standard (NAAQS) (CitationUSEPA, 1997). In recognition of the need for improved understanding of all aspects of PM2.5, EPA embarked on ambient monitoring research program commonly referred to as the Particulate Matter Supersites Program (CitationSolomon and Allen, 2004). This program, among other things, aimed to improve our understanding of (1) key sources of PM; (2) methods for the development of the most cost effective control strategies; and (3) the health risks associated with PM2.5 and its physical and chemical components.
Interest in high time resolution (one-hour or less) measurements of key components of ambient PM2.5 has increased significantly since the publication of the revised NAAQS for airborne particulate matter in 1997 (CitationUSEPA, 1997). McMurry (2001) reviews aerosol measurements and notes that prior to the mid-1990s, the major “online” method for aerosol sulfate measurement involved Flame Photometric Detection in a hydrogen-rich flame (CitationApel et al. 1988; CitationTanner et al. 1980; CitationSpengler et al. 1986). Since that time a number of measurement methods, and different implementations of similar methods, have been put forward. These include, but are not limited to, the humidified impaction strip/flash volatilization/pulsed fluorescence of SO2 method (CitationHering et al. 2003); the aerosol mass spectrometer (CitationJayne et al. 2000); the particle-into-liquid sampler followed by ion chromatography (CitationWeber et al. 2001; Orsini et al. 2003); the steam jet aerosol collector (CitationKhlystov et al. 1995; CitationSlanina et al. 2001); and wetted filter collector (CitationAl-Horr et al. 2003).
One major objective of the PM Supersites Program in general, and of the Particulate Matter Technology Assessment and Characterization Study—New York (PMTACS–NY) Supersite Program in particular has been performance testing of PM measurement methods and instrumentation (CitationDrewnick et al. 2003; CitationSchwab et al. 2004a; CitationHogrefe et al. 2004a). The measurement of sulfate is of great importance to much of the Eastern half of the United States, and in many places around the world, because it and carbon are typically the two greatest contributors to PM2.5 mass loadings in most of these areas. (CitationSchwab et al. 2004b, CitationNARSTO 2003) In particular, continuous or semi-continuous measurements of PM2.5 sulfate are of great interest for a wide variety of purposes, including (1) air quality forecasting; (2) near-real-time public health reporting; (3) model and State Implementation Plan (SIP) evaluation, including analysis of transport vs. local production; (4) visibility and regional haze; (5) trends analysis; and (6) analysis of intense short-time scale exposures. CitationDrewnick et al. (2003) and CitationHogrefe et al. (2004a) have reported on our earlier efforts where we tested and evaluated a number of semi-continuous PM sulfate instruments.
This study focuses on the commercial version of the reduction oven–pulsed fluorescence method patented by the Harvard School of Public Health and manufactured by Thermo Electron, Inc. (Franklin, MA) The pre-production instrument was deployed for a four month period from July to November of 2004 at the ASRC research station in Addison, NY at Pinnacle State Park. Following the field deployment, this instrument was operated in our aerosol laboratory (CitationHogrefe et al. 2004b), where its measurements were compared to those from a TEOM. (TEOM stands for Tapered Element Oscillating Microbalance, a continuous mass concentration monitor (CitationPatashnick and Rupprect 1991).) The final evaluations involved the collocated operation of the pre-production instrument and two regular production instruments in our aerosol laboratory. In these tests, the instrument responses were compared to those from filter samples analyzed for sulfate, as well as those from the continuous TEOM monitors. This was followed by a three week period during which the three sulfate instruments sampled ambient air from a common manifold.
EXPERIMENTAL METHODS AND SITE DESCRIPTION
Thermo Electron 5020 Sulfate Particulate Analyzer—Description of the Pre-Production Prototype and Production Versions
The Thermo Electron 5020 utilizes a new measurement method for sulfate aerosol. The key step in this method is the transformation of particle sulfate to gaseous sulfur dioxide in a thermal reduction oven operating in the temperature range of 800–1000°C. The commercial version operates at 1000°C to optimize the sulfate to SO2 conversion. In an earlier research implementation of this method the aerosol passed through a stainless steel tube heated in tube furnace to a temperature of 900°C (CitationDrewnick et al., 2003). The pre-production and production versions of the instrument used in this work use a quartz tube containing a stainless steel rod (approx. 6 mm diameter and 100 mm long). The heated stainless steel is critical to the reduction reactions which convert the particle sulfate to sulfur dioxide gas.
We include here a short description of the pre-production instrument as it was deployed for the four months of field measurements. Ambient air is drawn through a sharp cut cyclone (BGI) with a 2.5 μ m cut point at a flow rate of 5 L/min. Inside the shelter this sample flow is split into a roughly 1.1 L/min sample/filter flow and a roughly 3.9 L/min bypass or make-up flow. The bypass flow passes through a rotameter flow meter and is exhausted through a dedicated bypass pump. The flow schematic for this version of the instrument is illustrated in . The sample/filter flow passes through a sodium carbonate coated annular denuder to remove ambient SO2 (Sunset Labs) and is subsequently split at a tee into independent sample and filter flows. The sample portion (∼ 450 cc/min) flows directly into the quartz tube, the center portion of which contains the stainless steel rod maintained at 1000°C. Downstream of the reduction oven, the sample flow passes through a PTFE filter membrane and into a trace-level SO2 pulsed fluorescence analyzer. The analyzer is largely identical to the 43C-TL SO2 analyzer (Thermo Electron Co., Franklin, MA) with two notable differences. First, the operating software is specific to the 5020 instead of the 43C-TL; and second, the “hydrocarbon kicker” (a concentric flow device with the inner tube consisting of a membrane through which hydrocarbons, and not SO2, will permeate) is not installed as part of the 5020.
FIG. 1 (a) Schematic of sample and filter flows for the pre-production version of the 5020 analyzer used in these experiments. (b) Schematic of sample and filter flows for the final production versions of the 5020 analyzer.
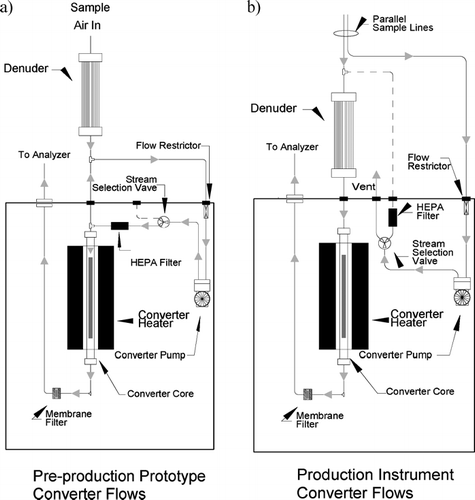
As noted above, the filter flow for this version of the instrument is split from the sample flow after the denuder. This filter flow (∼ 700 cc/min, maintained by a capillary flow restrictor) passes through a capsule filter, a three-way valve, then the capillary restrictor and a diaphragm pump, and finally through another three-way valve and a PTFE membrane filter before being introduced (during the filter period of the operating cycle) at a “tee” immediately upstream of the quartz tube and the oven. The filter flow is maintained at a rate higher than the sample flow. This assures that all the air flowing into the oven and the analyzer during filter periods has passed through the filter. The filtered air nominally contains the same gaseous SO2 as the sample air, but none of the particle sulfate.
The most notable difference between the prototype version of the analyzer that was deployed for field measurements and used for the first series of laboratory experiments, and the production version analyzers used in the second series of laboratory experiments, is a change in the plumbing of the filter flow. The prototype flow scheme is described above. In the production version (illustrated in ), the sample and filter flows are split before the sodium carbonate denuder. During filter periods, the filter flow is introduced upstream of the denuder. The manufacturer instituted this change to stabilize any slight flow and pressure differentials present in a switched mode instrument, with the goal of attaining a lower and more stable zero response from the instrument.
Sample and filter periods are user selectable. During the first month of field deployment these parameters were set to 50 min for the sample period and 10 min for the filter period. These are the same periods used for our deployments of the research version of the instrument used in 2001 and 2002 (CitationDrewnick et al. 2003; CitationHogrefe et al. 2004a). This choice gives a greater fraction of sample period data, but may sacrifice a little in terms of sensitivity or noise because the baseline is not measured as frequently, or for as large a fraction of the time. For the last three months or so we set the sample and filter periods to 10 min and 5 min, respectively.
Routine and field calibration of the 5020 consists only of gaseous calibration of the pulsed fluorescence analyzer. The production and characterization of test aerosols is a major research effort (CitationHogrefe et al. 2004b), and routine calibration of field instruments with aerosols is not yet practical. We calibrated the SO2 analyzer with a NIST-traceable gaseous standard from Scott-Marrin (Riverside, CA). The cylinder concentration was recertified by the New York State Department of Environmental Conservation Quality Assurance laboratory in March 2005 and the recertification concentration was within 1.1% of the December 2001 original value. The analyzer calibration was checked three times during the field campaign, and at four times during the course of the laboratory evaluation period. Deviations of measured versus expected values were less than 10% for all calibration checks.
ACCU
The Automated Cartridge Collection Unit (ACCU) is an optional accessory to the Rupprecht & Patashnick Model 1400 TEOM monitor. 24-hour filter samples (midnight to midnight) were collected daily using this sampler. The ACCU sampler shares the inlet with a TEOM and collects the particles in its 13.67 L/min bypass flow. The TEOM/ACCU inlet consists of a PM10 inlet followed by a PM2.5 sharp-cut cyclone, both supplied by Rupprecht & Patashnick. No denuder is used to remove gaseous interferents. The ACCU sampler has a valve controlled manifold that feeds one of eight filter channels, each of which contained a 47-mm diameter Zefluor filter (Pall Gelman) mounted in a plastic filter holder. A blank filter is collected weekly by placing an ACCU filter holder and filter inside the sampler (but not exposed to sample flow) during sample collection. Analysis for SO4 2− was carried out using ion chromatography at the Wadsworth Center laboratories of the New York State Department of Health (NYSDOH) (CitationDutkiewicz et al. 2004).
STN
New York State and ASRC jointly operate a Rupprecht & Patashnick Co., Inc. (R&P) Partisol Model 2300 Chemical Speciation Sampler according to the Speciation Trends Network (STN) protocol at the Addison site. 24-hour integrated samples are collected following the 1-in-3 day schedule distributed by EPA and shipped to RTI for analysis. The sampler has been described by CitationSolomon et al. (2003). In the STN protocol installations the instrument consists of three sampling modules (Chem-Comb™ cartridges) connected to individual flow controllers and a single vacuum pump. The inlets are impactors with PM2.5 cutpoints described by Demokritou et al. (2001). Channel 1 contains a Teflon filter that is analyzed gravimetrically for mass and for elements by XRF. The flow through this channel is 16.7 L/min (liters per minute). Channel 2 contains a single quartz filter, without denuder, which is analyzed for carbon (EC, OC, TC, Carb, OCX, Peak1-4, PyrolC) using the modified NIOSH 5040 method adopted by the EPA (CitationBirch 1999; CitationBirch and Cary 1996). The flow through this channel is 10 L/min. Channel 3 includes a Na2CO3 coated honeycomb denuder to remove acid gases followed by a nylon filter, which is analyzed for nitrate, sulfate, ammonium, sodium, and potassium using IC (ion chromatography). The flow through this channel is 10 L/min.
ASRC Aerosol Laboratory
The aerosol generation and characterization laboratory has been described in some detail by CitationHogrefe et al. (2004b). For these experiments we generated chemically pure sulfate salt aerosols using an atomizer from TSI. Inc. (Model 3076). Salt solutions with concentrations of approximately 1 g/L were used to generate aerosols. Mass concentrations in the slow-flow chamber were adjusted to our working range of 10–100 μ g/m3 through a combination of capillary dilution and dry or humid air dilution to attain a total chamber flow in the range of 30–40 L/min. For a total chamber flow of 40 L/min the aerosol equilibrates for about 10 minutes before reaching the sample ports where it is drawn off to the various sampling instruments.
Mass concentrations of the generated aerosols were measured using a standard TEOM monitor with a Nafion dryer (an SES TEOM), a differential TEOM with an electrostatic precipitator (ESP) (CitationSchwab et al., 2004c), and an R&P Partisol (Model 2000) integrated filter sampler. The TEOM monitors were operated with a mass transducer and filter temperature of 30°C for the first set of laboratory experiments, and 50°C for the second set of laboratory experiments. The higher temperatures were used for the second set of experiments to further minimize the effect of particle bound water on the measured aerosol mass. Filter samples are handled according to EPA Federal Reference Method 40 CFR Parts 50, 53, and 58 (CitationFederal Register 1997). The filter samples were collected on PTFE filter membranes, and after post-weighing, were analyzed for sulfate by IC using the same analysis protocol used for the ACCU filter samples.
Site Description
Pinnacle State Park (PSP) (42.09°N, 77.21°W, 505 m above MSL) in Addison, New York, is a rural site operated by ASRC located in the “transport corridor” between the industrial Midwest and the Eastern seaboard. The air monitoring shelter is located on the eastern side of Orr Hill, part of the Allegheny Plateau of southern New York State and northern Pennsylvania. The surrounding areas include a 9-hole golf course, a picnic area, undeveloped state forest lands, abandoned pastures, a 20-hectare pond, and a handful of homes. The village of Addison (pop. ∼ 1800) is about 4 km to the northwest and the town of Corning (pop. ∼ 12,000) is 15 km to the northeast.
RESULTS
Field Evaluation
The 5020 was set up and operational at the Addison site on July 13, 2004. Operation and data collection continued until November 2, 2004. Data collection and summary statistics for the period July 14 to November 1, 2004 are given in . Three valid 15-minute cycle data values are required to obtain a valid 1-hour averaged data point, and 18 valid hour-averaged data points are required to obtain a valid 24-hour averaged value for the 5020.
TABLE 1 Summary statistics for field deployment of 5020 Sulfate Particulate analyzer
The zero response of the instrument was measured by replacing the cyclone with a Millipore Opticap XL2 filter for a 24-hour period. During this period the instrument response was 0.062 ± 0.100 μg/m3 (uncertainty expressed as 1*SD). This zero response signal was used to adjust the final data set. The minimum detection limit (MDL) or lower detection limit (LDL) of the instrument is estimated to be 0.3 μg/m3, obtained as three times the standard deviation of this series of dynamic blank values.
The time series of hour-averaged sulfate concentrations is shown in . As is evident from the figure, there were several short periods of high sulfate concentrations, three of which exceeded 20 μ g/m3. Many of these sulfate events lasted 24 hours or less, and none lasted more than about two days. This behavior was undoubtedly due, at least in part, to the unusually cool and wet summer experienced at the site. The rainfall totals for the summer months were July (28.0 cm or 11.03″); August (17.0 cm or 6.7″); and September (20.3 cm or 8.0″). These are two to three times greater than normal values. The maximum temperature did not exceed 30°C (85°F) during this period, another unusual occurrence. About half of the periods of very low sulfate concentration correspond to significant rainfall events. For example, the low values measured on 8/5–8/7 do not correspond to precipitation events, while the low values measured 7/27 and 8/21 do correspond to precipitation events. As noted above, the STN filter samples were collected every third day, while the ACCU filter samples were collected daily. These filter measurements exhibit a high degree of correlation and agreement, giving us a high degree of confidence in both sets of filter measurements.
FIG. 2 Time series of hour-averaged PM2.5 sulfate measurements at Addison, NY from mid-July to early November 2004.
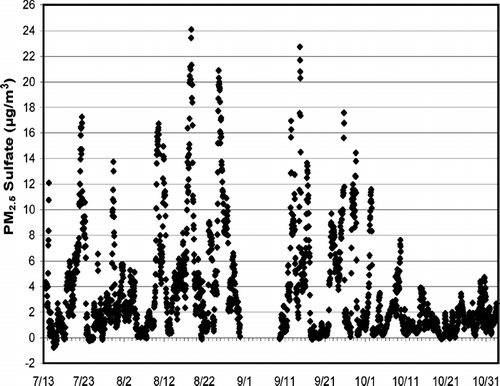
There are 77 valid ACCU samples and 30 valid STN samples during the measurement period, so we will use mainly the ACCU data for comparison with the 24-hour averages of the 5020 data. There were 5 days in late July and early August where ACCU samples were not available due to a TEOM malfunction but STN samples were available, and two additional days during the study where STN samples were available, but ACCU samples were not. There is excellent agreement between the data sets, and STN values were used for these seven sample days. shows the time series comparison of the 5020 24-hour averaged values with the filter sulfate measurements from the combined ACCU/STN data set. These data sets show a good degree of agreement, in many cases falling right on top of each other. Generally speaking, however, the averaged continuous measurements from the 5020 are lower than the filter sulfate measurements, in some cases (7/23/04 and 8/1/04) by as much as a factor of two. presents the correlation plot of these data, along with the results of a linear regression fit to the data. The points fall consistently near, but below the 1:1 line and cluster around a ratio of 0.8, implying an 80% conversion/detection efficiency for this configuration of the 5020 analyzer. If 5 outlier points are removed from the regression analysis, each at a distance from the regression line of at least 12 times the standard error, the slope increases to 0.81 and the R2 increases to nearly 0.97.
FIG. 3 Time series plot of the 24-hour averaged 5020 sulfate and filter sulfate from the combined ACCU/STN data set collected at the Addison field site.
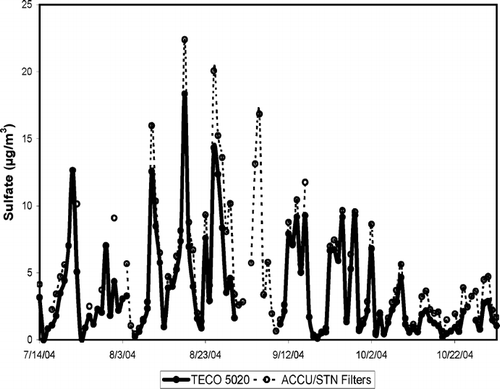
FIG. 4 Correlation plot of the 24-hour data from Addison, along with the 1:1 line for reference, and the linear regression line and equation.
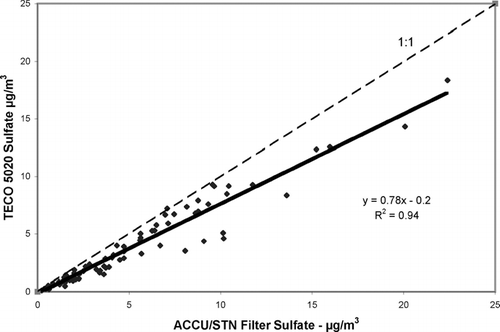
This result is different from that obtained by the Washington University group and reported in Brad Goodwin's Master Thesis (CitationGoodwin 2005). This group compared 5020 pre-production and production models to STN and other filter sulfate measurements in the St. Louis, Missouri area. Considering only 24-hour averaged values, they obtain correlation regression slopes of 1.04 and 0.983, with R2 values of 0.95 and 0.96, for the more extensive data set comparing the pre-production version with the filter measurements. From a much more limited data set (7 and 14 points, respectively) comparing two production version 5020's to STN filter sulfate, they obtain correlation regression slopes of 0.94 and 0.89. While our results overlap with those of Goodwin when uncertainty is considered, each group sees high precision in the measurements and the difference is puzzling. Two possible explanations include: (1) one or both groups has a small systematic error in their measurement procedure; or (2) that the sulfate-containing aerosol in St. Louis is more readily catalytically converted to SO2 than is the laboratory synthetically generated aerosol or the sulfate containing aerosol in the Northeast. We intend to pursue this second possible explanation of the difference by deploying the production version 5020 instruments for long-term measurements at our rural site in Addison and in New York City. Preliminary results from two months of data indicate that the 5020 sulfate conversion efficiency in New York City is also around 80%. If there is a difference in catalytic conversion efficiency due to composition of the aerosol, long term urban/rural comparisons may provide our best chance to resolve this apparent 15–20% difference between our results and those from the Midwest.
Laboratory Evaluations
After the field deployment, the production prototype 5020 was operated in the ASRC aerosol laboratory described above. The instrument was challenged with mass concentrations of generated sulfate salt aerosols in the 30–50 μ g/m3 range. The reference instruments for comparison were two TEOM monitors. The second set of experiments, described below, tested two production versions of the instrument and also used filter samples for comparison. As in previous work (CitationSchwab et al. 2004c), the TEOM measurements agreed very well with the filter measurements, confirming the use of the TEOMs as useful “transfer standard” measurement methods.
Results from this first set of laboratory measurements are summarized in . The test aerosol for the first three days of experiments was ammonium sulfate. The TEOM data shows larger excursions than the 5020, which we take to be indicative of instrument noise and not changing aerosol in the chamber. The 5020 data for ammonium sulfate was adjusted up by a factor of 1.375 to account for the ammonium mass. If the data for all three days of experiments using ammonium sulfate are averaged, the ratio is 0.78 ± 0.12. This value is identical to the regression slope for the field data shown in .
TABLE 2 Laboratory measurements of inorganic sulfate aerosols using the pre-production 5020 and a TEOM monitor
Experiments carried out using potassium, sodium and calcium sulfate test aerosols confirmed that the reduction oven was less efficient at converting these species to SO2. The ratios of aerosol mass detected by the 5020 (again corrected for cation mass) to aerosol mass detected by the TEOM were 0.63 ± 0.05 for potassium sulfate, 0.20 ± 0.04 for sodium sulfate and 0.04 ± 0.02 for calcium sulfate. These lower efficiencies are important to consider for locations with high concentrations of sea salt or mineral dust sulfate, and also for the use of the 5020 for coarse mode sulfate. That is, the 5020 is not equally efficient at converting all types of sulfate, so it cannot be 100% accurate. However, in the Northeast U.S., sodium, potassium, and calcium make up less than 2% of the aerosol mass, and their combined average mole concentration is less than 4% of the ammonium mole concentration (CitationSchwab et al. 2004b). This means that in high sulfate regions the error introduced due to the failure to quantitatively detect metal ion sulfate salts is significantly less than the measurement uncertainty.
The second set of laboratory evaluations included two new production 5020 instruments and filter samples collected with the Partisol sampler and analyzed for mass and sulfate. Experiments were run over five days, this time with total aerosol mass concentrations in the chamber between 15 and 55 μ g/m3. Two different values of relative humidity were used; very dry (RH < 2%), and RH ∼ 50%. Prior to the evaluations with generated aerosol, the new analyzers and the pre-production analyzer were first calibrated and tested for dynamic zero response. As noted above, the SO2 standard gas and the dynamic dilution calibrator was recertified immediately prior to these calibrations. Laboratory filtered air was used to determine the dynamic zero response of the instruments, a very useful diagnostic of proper instrument set-up and operation. The zero responses of the new instruments for an approximately 20-hour period were −0.02 ± 0.08 and −0.03 ± 0.06 (1 sigma). The zero response of the prototype instrument was −0.31 ± 0.16, larger in magnitude, but still in an acceptable range. We are not sure why the zero response in the laboratory is so different from that measured in the field. One of the reasons the manufacturer changed the plumbing between the pre-production and the production units was to achieve a zero response closer to zero. This appears to have been successful.
Comparisons of the filter determined sulfate, and filter determined sulfate plus calculated ammonium are plotted in versus the corresponding sulfate measured from the 5020, and the aerosol mass measured by the TEOM monitor, respectively. Six filter samples were collected and analyzed as part of the study; three samples were collected over a period of four hours, and three samples were collected over a period of eight hours. The results of the linear regressions of these six points are included in . The points are well correlated, and the regression slopes are consistent with previous results. In particular, the 5020 conversion/detection efficiency is slightly higher than for earlier tests, but the two values are well within the combined error limits. The regression y-intercepts are noticeably different than zero for each regression fit, but in each case the values are significantly less than the standard error value for the fitted constant. If the 5020 versus filter sulfate regression is constrained to pass through the origin, the fitted slope increases to 0.85, and the regression R2 is essentially unchanged.
FIG. 5 Comparisons of continuous TECO 5020 sulfate and TEOM mass concentration measurements with integrated filter sulfate measurements. The 5020 measurements are the average of the two production instruments (S/N's 1037 and 1038).
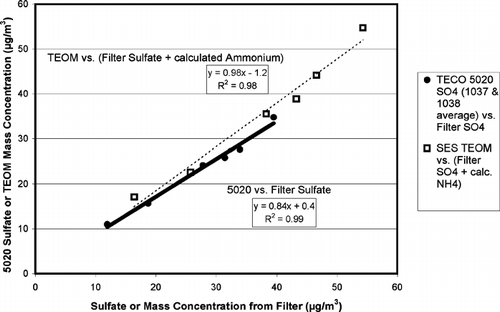
The correlation plot of sulfate from the 5020 plus calculated ammonium versus measured total aerosol mass from the TEOM using data from all five days of this set of laboratory evaluations is given as . Consistent with the filter results, and with the results from the first set of lab experiments, the slope of this line is also very close to 0.8. If the nine most obvious outliers are removed from the regression (four above the linear fit line and five below), the R2 parameter increases to nearly 0.98, but the slope increases by less than 2% and the intercept decreases by only 15%. The y-intercept of the linear fit in is almost five times the standard error of the fitted constant. This is a significant offset, and does seem to indicate some sort of systematic error. Still given the range of values displayed in the plot, a roughly 3 μ g/m3 offset is only capable of generating a relatively small absolute error. If the linear regression fit is constrained to pass through the origin, sometimes called the “recovery,” the slope coefficient is 0.87 and the R2 coefficient decreases to 0.93.
FIG. 6 Correlation plot of continuous sulfate (plus calculated ammonium) versus continuous mass concentration (MC) from the TEOM monitor for the second set of laboratory experiments. As in , the 5020 values are the average of the two production instruments.
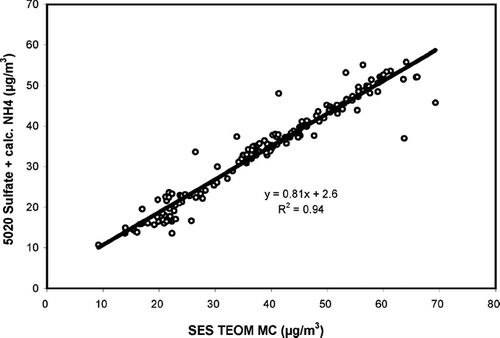
The sulfate concentrations measured by the two production version 5020 analyzers during this series of tests are illustrated in . The instruments show a high degree of precision, as evidenced by the R2 value, but there appears to be a bias of about 4–5%. This apparent bias is within the estimated uncertainty of the instruments.
FIG. 7 Measured sulfate values from the two 5020 continuous sulfate analyzers during the laboratory experiments. Each data point corresponds to a 15-minute sample/filter cycle of the instrument.
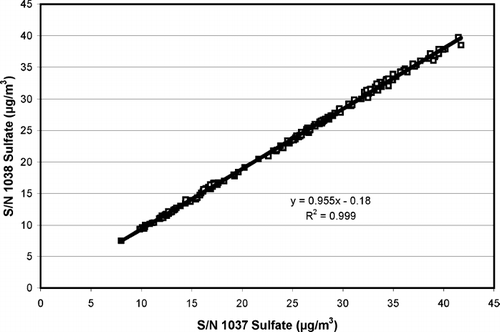
The final laboratory tests were conducted to test for interferences from organic aerosols or nitrate aerosols. The concern with the organic aerosols is due to the removal of the hydrocarbon kicker in the analyzer; and with the nitrate aerosols is due to the close proximity of spectral features of NO and NO2 to those of SO2. Experiments testing the 5020 with succinic acid aerosol concentrations up to 50 μ g/m3 and potassium nitrate aerosol concentrations up to almost 40 μ g/m3 yielded undetectable amounts of sulfate as measured by the 5020. Based on the standard deviations of the 5020 fifteen minute data points during these tests, we estimate the upper limits for the interference from succinic acid and potassium nitrate at 0.8% and 1.1%, respectively.
Side-by-Side Measurement of Ambient Aerosol
A follow-up to the laboratory precision comparison was performed by connecting all three instruments to a common inlet sampling ambient air for three weeks from a duct into our laboratory in Albany, NY. Also sampling from the duct was an R&P TEOM with a Nafion dryer. The three 5020's and the TEOM sampled air through a common cyclone, a BGI SCC1.829ARA with a 2.5 μ m cut-point at 5 L/min.
displays the simultaneous measurements, averaged to one hour, from the two production version 5020 instruments for the full three-week period. The similarity to is striking, with the regression slope being essentially identical. If the two highest points in the plot are removed the slope and intercept are identical (to three decimal places) and the R2 value decreases by less than 0.3%. The nearly 5% difference, while within the experimental error, deserves some discussion. It may well be due to a small bias introduced during the calibration of the analyzers. Our span checks of the analyzers was done at 20 ppb, and a 1 ppb error at this concentration is 5%. While it is quite possible to calibrate identical analyzers to within 5%, we believe the bias shown in and is most likely due to calibration bias.
SUMMARY
The Thermo Electron 5020 Sulfate Particulate Analyzer is a new commercial instrument based on pulsed fluorescence of sulfur dioxide gas following catalytic thermal reduction of sulfate aerosol in a 1000°C quartz oven containing a stainless steel rod as the catalyst. A number of research versions of this method, and pre-production versions of the instrument from Thermo Electron, have been deployed by SEARCH, Tennessee Valley Authority, the EPA St. Louis Supersite, and others (CitationGoodwin 2005). To our knowledge, this is the first laboratory evaluation of the commercial version of the instrument. The instrument was challenged with synthetic laboratory aerosols (mainly ammonium sulfate, but also sodium, potassium, and calcium sulfate) and compared to continuous mass measurements from two versions of the TEOM mass monitor; and to sulfate collected on filters and analyzed by ion chromatography. In addition, a pre-production version of the commercial instrument was deployed for more than three and a half months at a field station in Addison, New York. The field measurements are compared to daily sulfate measurements from filter samples, and to every third day sulfate measurements from STN filter samples.
The 5020 Sulfate Monitor performed quite well in the tests and field deployment described in this paper. Maintenance and calibration demands are relatively low, and certainly much lower than other continuous sulfate measurement systems like the PILS or the R&P 8400S. In both laboratory and field deployment tests we find that the 5020 is biased low compared to a TEOM, or to filters, by approximately 20%. Two separate sets of laboratory experiments, and a three and a half month field deployment all converge on the same value. Laboratory conversion efficiencies for calcium, sodium, and potassium sulfate salts ranged from 4% to 63%. These lower efficiencies for mineral-type sulfates will be an important consideration in areas with significant concentrations of sea salt or mineral dust sulfate, and less important for the high sulfate Eastern U.S., which is dominated by ammonium sulfate.
This work was supported in part by the New York State Energy Research and Development Authority (NYSERDA), contract # 4918ERTERES99, the U.S. Environmental Protection Agency (EPA) cooperative agreement # R828060010 and New York State Department of Environmental Conservation (NYS DEC), contract # C004210. Although the research described in this article has been funded in part by the U.S. Environmental Protection Agency, it has not been subjected to the Agency's required peer and policy review and therefore does not necessary reflect the views of the Agency and no official endorsement should be inferred. The authors wish to thank John Spicer for instrument operation and filter collection at the Addison site. Helpful guidance from and discussions with George Allen and Jay Turner are acknowledged.
REFERENCES
- Al-Horr , R. , Samanta , G. and Dasgupta , P. K. 2003 . A Continuous Analyzer for Soluble Anionic Constituents and Ammonium in Atmospheric Particulate Matter . Environ. Sci. Technol. , 37 : 5711 – 20 . [INFOTRIEVE] [CSA]
- Apel , B. R. , Tanner , R. L. , Adams , D. P. , Dasgupta , P. K. , Knapp , K. T. , Kok , G. L. , Pierson , W. R. and Reiszner , K. D. 1988 . “ Semi-Continuous Determination of Atmospheric Particulate Sulfur, Sulfuric Acid, and Ammonium Sulfates ” . In Methods of Air Sampling and Analysis, , Third Edition , Edited by: Lodge , James P. Jr. Boca Raton, FL : Lewis Publishers, Inc., CRC Press . Method 713
- Birch , M. E. 1999 . “ Elemental Carbon (Diesel Particulate): Method 5040 ” . In NIOSH Manual of Analytical Methods (NMAM), , Fourth Edition , Edited by: Cassinelli , M. E. and O'Connor , P. F. Washington, DC : National Institute for Occupational Health and Safety. Government Printing Office .
- Birch , M. E. and Cary , R. A. 1996 . Elemental Carbon-based Method for Monitoring Occupational Exposures to Particulate Diesel Exhaust . Aerosol Sci. Technol. , 25 : 221 – 241 . [CSA]
- Demoktitou , P. , Kavouras , I. G. , Harrison , D. and Koutrakis , P. 2001 . Development and Evaluation of an Impactor for a PM2.5 Speciation Sampler . J. Air & Waste Manage. Assoc. , 51 : 514 – 523 . [CSA]
- Drewnick , F. , Schwab , J. J. , Hogrefe , O. , Peters , S. , Husain , L. , Diamond , D. , Weber , R. and Demerjian , K. L. 2003 . Intercomparison and Evaluation of Four Semi-Continuous PM2.5 Sulfate Instruments . Atmos. Environ. , 37 : 3335 – 3350 . [CROSSREF] [CSA]
- Drewnick , F. , Schwab , J. J. , Jayne , J. T. , Canagaratna , M. , Worsnop , D. R. and Demerjian , K. L. 2004 . Measurement of Ambient Aerosol Composition During the PMTACS-NY 2001 Using an Aerosol Mass Spectrometer. Part I: Mass Concentrations . Aerosol Sci. Technol. , 38 ( S1 ) : 92 – 103 . [CROSSREF] [CSA]
- Dutkiewicz , V. A. , Qureshi , S. , Khan , A. R. , Ferraro , V. , Schwab , J. J. , Demerjian , K. L. and Husain , L. 2004 . Sources of Fine Particulate Sulfate in New York . Atmos. Environ. , 38 : 3179 – 3189 . [CROSSREF] [CSA]
- Federal Register . 1997 . Federal Reference Method—Appendix L to Part 50 , Reference Method for Determination of Fine Particulate Matter as PM2.5 in the Atmosphere Environmental Protection Agency . Federal Register, Friday, July 18, 1997, 40 CFR Part 50; http://www.epa.gov/ttn/oarpg/t1/fr_notices/pmnaaqs.pdf
- Goodwin , B. 2005 . Measurement of Ambient Black Carbon and Sulfate Aerosols at High Time Resolution , Master's Thesis St. Louis, MO : Environmental Engineering, Washington University .
- Hering , S. V. , Stolzenburg , M. R. , Hand , J. L. , Kreidenweis , S. M. , Lee , T. , Collett , J. L. Jr. , Dietrich , D. and Tigges , M. 2003 . Hourly Concentrations and Light Scattering Cross Sections for Fine Particle Sulfate at Big Bend National Park . Atmos. Environ. , 37 : 1175 – 1183 . [CROSSREF] [CSA]
- Hogrefe , O. , Schwab , J. J. , Drewnick , F. , Lala , G. G. , Peters , S. , Demerjian , K. L. , Rhoads , K. , Felton , H. D. , Rattigan , O. V. , Husain , L. and Dutkiewicz , V. A. 2004a . Semicontinuous PM2.5 Sulfate and Nitrate Measurements at an Urban and a Rural Location in New York: PMTACS-NY Summer 2001 and 2002 Campaigns . J. Air & Waste Manage. Assoc. , 54 : 1040 – 1060 . [CSA]
- Hogrefe , O. , Drewnick , F. , Lala , G. G. , Schwab , J. J. and Demerjian , K. L. 2004b . Development, Operation and Applications of an Aerosol Generation, Calibration and Research Facility . Aerosol Sci. Technol. , 38 ( S1 ) : 196 – 214 . [CROSSREF] [CSA]
- Jayne , J. T. , Leard , D. C. , Zhang , X. , Davidovits , P. , Smith , K. A. , Kolb , C. E. and Worsnop , D. R. 2000 . Development of an Aerosol Mass Spectrometer for Size and Composition Analysis of Submicron Particles . Aerosol Sci. Technol. , 33 : 49 – 70 . [CROSSREF] [CSA]
- Khlystov , A. , Wyers , G. P. and Slanina , J. 1995 . The Steam-Jet Aerosol Collector . Atmos. Environ. , 29 : 2229 – 2234 . [CROSSREF] [CSA]
- McMurry , P. H. 2000 . A Review of Atmospheric Aerosol Measurements . Atmos. Environ. , 34 : 1959 – 1999 . [CROSSREF] [CSA]
- NARSTO . 2003 . “ Particulate Matter Science for Policy Makers: A NARSTO Assessment ” . Part 2, Chaper 6–Spatial and Temporal Characterization of Particulate Matter; primary author C. L. Blanchard
- Orsinsi , D. A. , Ma , Y. , Sullivan , A. , Sierau , B. , Baumann , K. and Weber , R. J. 2003 . Refinements to the Particle-into-Liquid Sampler (PILS) for Ground and Airborne Measurements of Water Soluble Aerosol Composition . Atmos. Environ. , 37 : 1243 – 1259 . [CROSSREF] [CSA]
- Patashnick , H. and Rupprecht , E. G. 1991 . Continuous PM-10 Measurements Using the Tapered Element Oscillating Microbalance . J. Air & Waste Manage. Assoc. , 41 : 1079 – 1083 . [CSA]
- Schwab , J. J. , Spicer , J. , Demerjian , K. L. , Ambs , J. L. and Felton , H. D. 2004a . Long-Term Field Characterization of TEOM and Modified TEOM Samplers in Urban and Rural New York State Locations . J. Air & Waste Manage. Assoc. , 54 : 1264 – 1280 . [CSA]
- Schwab , J. J. , Felton , H. D. and Demerjian , K. L. 2004b . Aerosol Chemical Composition in New York State from Integrated Filter Samples: Urban/Rural and Seasonal Contrasts . J. Geophys. Res. , 109 : D16S05 [CROSSREF] [CSA]
- Schwab , J. J. , Hogrefe , O. , Demerjian , K. L. and Ambs , J. L. 2004c . Laboratory Characterization of Modified TEOM Samplers . J. Air & Waste Manage. , 54 : 1254 – 1263 . [CSA]
- Slanina , J. , ten Brink , H. M. , Otjes , R. P. , Even , A. , Jongejan , P. , Khlystov , A. , Waijers-Ijpelaan , A. , Hu , M. and Lu , Y. 2001 . The Continuous Analysis of Nitrate and Ammonium in Aerosols by the Steam Jet Aerosol Collector (SJAC): Extension and Validation of the Methodology . Atmos. Environ. , 35 : 2319 – 2330 . [CROSSREF] [CSA]
- Solomon , P. A. 2003 . Comparison of Integrated Samplers for Mass and Composition during the 1999 Atlanta Supersite Project . J. Geophys. Res. , 108 ( D7 ) : 8423 [CROSSREF] [CSA]
- Solomon , P. A. and Allen , D. 2004 . Special Issue of Aerosol Science and Technology on Findings from the Fine Particulate Matter Supersites Program . Aerosol Sci. Technol. , 38 ( S1 ) : 1 – 4 . [CROSSREF] [CSA]
- Spengler , J. D. , Allen , G. A. , Foster , S. , Severance , P. and Ferris , B. Jr. Sulfuric Acid and Sulfate Aerosol Events in Two U.S. Cities . Aerosols: Research, Risk Assessment, and Control Strategies—Proceedings of the Second U.S.-Dutch International Symposium . May 1985 , Williamsburg, VA. Edited by: Lee , S. D. , Schneider , T. , Grant , L. D. and Verkerk , P. J. Chelsea, Michigan : Lewis Publishers . Chapter 7
- Tanner , R. L. , D'Ottavio , T. , Garber , R. and Newman , L. 1980 . Determination of Ambient Aerosol Sulfur Using a Continuous Flame Photometric Detection System. 1. Sampling System for Aerosol Sulfate and Sulfuric Acid . Atmos. Environ. , 14 : 121 – 127 . [CROSSREF] [CSA]
- USEPA . 1997 . 40 CFR Part 50; National Ambient Air Quality Standards for Particulate Matter. Final Rule . Fed. Regist. , 62 ( 138 ) : 2 – 102 . [CSA]
- Weber , R. J. , Orsini , D. , Daun , Y. , Lee , Y. -N. , Klotz , P. J. and Brechtel , F. 2001 . A Particle-into-Liquid Collector for Rapid Measurment of Aerosol Bulk Chemical Composition . Aerosol Sci. Technol. , 35 : 718 – 727 . [CROSSREF] [CSA]