Abstract
The P-trak ultrafine particle counter is a portable version of a condensation particle counter (CPC). Both instruments detect particle number concentrations in real time but have different detection limits. The P-trak has been widely used for indoor air quality evaluation and aerosol research. However, there is very limited information about the reliability and precision of this instrument and its comparability with other similar instruments. The purpose of this study was to compare a P-trak ultrafine particle counter with a standard CPC and evaluate its applicability to ambient air monitoring.
This study was carried out near the Interstate 405 freeway (I-405) in Los Angeles. Measurements were made at increasing distances from the freeway on both sides at night as well as inside and outside of two 2-bedroom apartments located near the freeway. A CPC and a Scanning Mobility Particle Sizer (SMPS) were collocated with two P-traks and measurement results compared.
In general, higher correlations were observed between P-trak and CPC data for indoor measurements than outdoor. The highest P-trak and CPC correlation ( r 2 = 0.9385) was detected inside Apartment 2, which is located farther away from the freeway than Apartment 1. The poorest correlation occurred at 30 m downwind from the freeway. In that case, the P-trak reported about 25% of ultrafine particle concentration that CPC did. A sigmoid (S-shape) function was fitted to observed P-trak to CPC ratios and geometric mean diameters of the corresponding ultrafine particle size distributions. Overall, we concluded the P-trak worked reasonably well when sampled indoor air. However, it has significant limitations in detecting freshly emitted ultrafine particles from vehicles. The P-trak underestimated ultrafine particles especially for particles smaller than its activation size which was found to be approximately 25–30 nm. Caution must be given in interpreting data collected by P-trak monitors near combustion sources.
INTRODUCTION
Atmospheric particulate matter (PM) is of great concern due to its potential for causing respiratory and cardiac diseases in vulnerable people (CitationNemmar et al. 2002, Citation2004). There is evidence that the health hazard associated with particulate matter is more closely correlated with number concentration than mass concentration. Particle mass concentration is dominated by larger particles. Whereas most of the number of particles are in the ultrafine size range (Dp < 100 nm) and can reach and deposit in the alveoli region of the lung (CitationJaques and Kim 2000). Recent toxicological and epidemiological studies have shown that ultrafine particles are more important from a health perspective than larger particles (CitationPakkanen et al. 2001; CitationPeters et al. 1997; CitationWichmann and Peters 2000; CitationWichmann et al. 2000). It has been suggested that particle number concentration could be used to better reflect the adverse health effect of the PM exposure.
At present, growth by condensation is the most commonly used technique to detect ultrafine particles. Based on this technique, TSI manufactured a class of Condensation Particle Counters (CPC), which has then been used in research and industrial applications around the world for many years. The working principles for different versions of CPCs are more or less the same. Particles are drawn through the instrument by a pump. Upon entering the instrument, particles pass through a saturator tube where they mix with alcohol vapors. The particle/alcohol mixture is then drawn into a condenser tube, which cools the air/particle stream causing alcohol to condense on the particles and the particles grow into detectable droplets. The droplets then pass through a focused laser beam, producing flashes of scattered light. The scattered light flashes are sensed by photodetector and counted to determine particle number concentration.
Among all TSI CPCs, P-trak Ultrafine Particle Counter (UPC) (model 8525, TSI Inc., St. Paul, MN) is a battery operated, portable instrument. The P-trak UPC uses the same fundamental technology to detect and count ultrafine particles in real time. The working fluid, however, differs between instruments. Due to its portability and ease of use, the P-trak has wide application in indoor air quality evaluation and field aerosol research.
P-trak is known to have a larger minimum detectable particle size compared to a general-purpose CPC. However, there is very limited information about the reliability, precision, comparability, and the minimum detectable size of this instrument. Previous studies have shown that the concurrent measurement of ultrafine particles by a general-purpose CPC (model 3022, TSI Inc., St. Paul, MN) and a P-trak in a laboratory achieved very high correlations (CitationChan et al. 2004). In that study the CPC consistently reported approximately 30% more counts of particles than the P-trak. A recent study performed a field comparison between a P-trak and a portable CPC 3007 (CitationMatson et al. 2004). Their results revealed a good correlation between the two instruments. The differences between these two instruments were less than ±20%. The working fluids for both CPC 3007 and P-trak 8525 are isopropyl alcohol. There are no data available on field performance of P-trak monitors as compared to general-purpose CPCs especially in microenvironments where high ultrafine particle concentrations are expected, for example near major roadways where high concentrations have been reported (CitationReponen et al. 2003; CitationZhu et al. 2002a, Citation2002b). The purpose of this study is to compare P-trak performance with a regular CPC and evaluate their applicability to ambient air monitoring. We will use “P-trak” to refer to P-TRAK® Ultrafine Particle Counter, and “CPC” to refer to CPC 3022a from now on.
EXPERIMENTAL METHODS
P-trak field calibrations were performed in connection with two major field campaigns in the vicinity of the I-405 Freeway in Los Angeles, CA. Freeway 405 has nine to ten lanes and runs generally north and south near the sampling locations. A general wind pattern persists in the sampling area. A consistent onshore sea breeze (eastward, from the ocean) develops each day in the mid-morning, reaches its maximum early to mid-afternoon, and dies out in the early evening. A weaker offshore sea breeze (westward, to the ocean) usually occurs at night. The eastern side of the I-405 was the downwind site during the daytime and the upwind site during the night and vice versa for the western side.
A Scanning Mobility Particle Sizer (SMPS 3936L22, TSI Inc., St. Paul., MN), a Condensation Particle Counter (CPC 3022a, TSI Inc., St. Paul, MN) and two P-trak (model 8525, TSI Inc., St. Paul, MN) ultrafine particle counters were used for this study. The SMPS is the premier instrument for measuring high-resolution size distributions of ultrafine particles. Particles are classified with an Electrostatic Classifier and their concentrations are measured by a CPC and corrected by the Aerosol Instrument Manger software (AIM software, TSI Inc., St. Paul, MN). The sampling flow rate in this experiment was adjusted to 1.5 L/min to permit measuring particles as small as 7 nm and to minimize the diffusion losses of ultrafine particles during sampling. The 3022a CPC was treated as the standard particle counters in this study.
First campaign was conducted inside and outside of two 2-bedroom apartments located on the eastern side of I-405 freeway. Data were collected when windows were closed. When sampling lines were passed through the window, the window was sealed. Apartment 1 is about 15 m from a sound barrier wall at the edge of the freeway and apartment 2 is about 40 m from the wall. Both apartments are on the third floor with windows 5.5 m above the ground. In the first apartment (Apt No. 1), a SMPS, a second CPC and a P-trak were used to simultaneously measure indoor and outdoor ultrafine particle concentrations. In the second apartment (Apt No. 2), one CPC and two P-traks were used. The CPC measurements were made through a common switching manifold, which alternately sampled indoor and outdoor air, each for 10 minutes. The manifold consisted of two identical 1.5 m long sampling arms, one inside the sampling room with inlet located at breathing height (approximately 1.6 m) and the other extended outside through a window in the apartment. One P-trak measured indoor and a second P-trak measured outdoor ultrafine particle concentration. Sampling lines for both indoor and outdoor P-traks were kept at 1.5 m, similar to the CPC. For 20 nm particles, diffusion losses through these sampling lines were determined to be less than 5% (CitationHinds 1999). Detailed description of the apartments, sampling site and sampling protocol can be found in CitationZhu et al. (2005). The two P-trak Ultrafine Particle Counters were also tested side-by-side simultaneously under various conditions, which included inside apartments with and without cooking activities and outside apartments near and away from the freeway. In total, there are approximately 4300 data pairs obtained for both P-traks for this test.
The second campaign was conducted at nighttime (11 P.M. to 4 A.M.) on both sides of Freeway 405 for seven nights. The SMPS, CPC and P-trak were placed on an electric vehicle and were driven together to sample simultaneously at 30, 60, 90, 150, and 300 m upwind and downwind from the center of the I-405 freeway. It took about 6 minutes to complete sampling in one location and about 50 minutes to finish a set of five sampling points on one side of the freeway. Three to four sets were performed at the downwind side and one or two sets were performed at the upwind side. In total five sets were performed during each sampling night. Detailed experimental setup for this campaign can be found elsewhere (CitationZhu et al. 2006).
Throughout the measurements, P-trak ultrafine particle counters collected data at one-minute intervals, while the CPC and the SMPS took measurements at two-minute intervals. P-trak data were exported in the TrakPro software (version 3.33, TSI Inc., St. Paul, MN). SMPS and CPC output were exported in the Aerosol Instrument Manager software (version 5.1, TSI Inc., St. Paul, MN). P-trak data were converted to two-minute average for comparison with SMPS and CPC results in Statistical Analysis System (SAS version 8.01).
RESULTS AND DISCUSSION
Precision of P-Trak Ultrafine Particle Counter
The precision of the collocated P-trak ultrafine particle counters for measurement of particle number concentration was found to be very high. illustrates the degree of correlation found among the one-min average readings of the two P-trak instruments. Observed particle number concentrations ranged from 1,800 particles/cm3 to 280,000 particles/cm3. The readings were highly correlated with r 2 greater than 0.99 and slopes within ±3% of unity.
P-Trak and CPC Comparison
depicts a typical time series plot of P-trak and CPC measurements. In general, P-trak readings were almost always lower than CPC readings. P-trak readings seem to track CPC readings very well. This suggests that the P-trak can be used to provide information on relative particle number concentrations for exposure studies. However, caution must be used when interpreting P-trak data, especially near combustion sources as discussed below.
In our first field campaign, the best P-trak and CPC correlation was observed inside the second apartment (Apt No. 2) and was plotted in . The second apartment is about 40 m downwind from the edge of the freeway 405. Two P-traks and one CPC were used to measure particle number concentration at one-minute intervals. One of the P-trak sampled indoor air and the other one sampled outdoor air. The CPC inlet was connected to a timer-controlled valve, which was alternatively switched between indoor and outdoor air every 10 minutes. Indoor data were collected without indoor activities. In total more than 700 data pairs were obtained and used in . A slope close to 1 and a very high r 2 value were observed for indoor aerosols comparison. This suggests that P-trak works quite well in indoor environments without indoor activities.
FIG. 3 Indoor P-trak readings vs. CPC readings in the second apartment. The dash lines indicated the 95% confidence interval of the regression line. The dotted line indicates the 1:1 ratio.
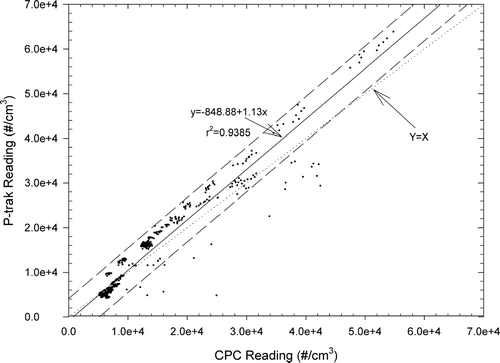
In our first field campaign, the weakest P-trak to CPC correlation was observed outside of the first apartment (Apt No.1) and is shown in . In the first apartment, which is about 15 m from the edge of the freeway 405, one P-trak was sampling side by side with a CPC and a SMPS either inside or outside of the apartment. Three full days of outdoor sampling and two days of indoor sampling were performed in this apartment. More than 1300 sets of outdoor data were used in . Each data set includes a pair of two-minute averaged P-trak and CPC readings and an associated SMPS scan. Two distinct bands were observed for data in . Previously, we have reported strong and consistent diurnal wind patterns for the sampling site (CitationZhu et al. 2005a, Citation2005b). This apartment was downwind of the freeway during daytime and upwind of the freeway at night. The data were then dichotomized into two groups: upwind background aerosol versus downwind freeway aerosol. Linear regression indicates that the P-trak can detect about 30% of freeway aerosols and 75% of background aerosols that the CPC detected. It is clear that type of aerosols and presumably the shape of their size distributions strongly influence the relative concentrations measured by the P-trak and the CPC.
FIG. 4 Outdoor P-trak readings vs. CPC readings in the first apartment when the apartment is upwind (gray symbol) and downwind (black symbol) of the Freeway 405.
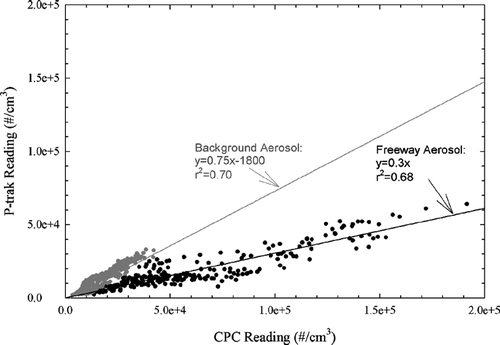
Comparing data collected in the indoor and outdoor environments, the r 2 value was always higher for indoor data than outdoors for both apartments, indicating that the P-trak and CPC data collected indoors had a better correlation than those collected outdoors. This may be due to the fact that outdoor particle concentrations changed much more dramatically than indoor concentrations. It appears that the P-trak could not respond to particle number concentration changes as fast as the CPC.
In our second field campaign, a P-trak ultrafine particle counter, a CPC and an SMPS were used to sample simultaneously at different distances upwind and downwind from the 405 freeway. During this nighttime study, the western side was the downwind side and the eastern side was the upwind side of the freeway. summarizes CPC and P-trak measured average particle number concentrations at different distances from the freeway. Standard deviations are also included in parenthesis in the table. P-trak measurements appear to track CPC measurements well on both sides of the freeway. The ratio of particle number concentration measured by the CPC and P-trak on the eastern side (upwind side) was fairly constant for all the sampling points except the closest point, which may have received some of the freeway exhaust due to turbulence. Both CPC and P-trak readings on the western side (downwind side) dropped 50–60% between 30 and 500 m downwind from the freeway. In general, the P-trak measured number concentration 25–30% of that for the CPC on the downwind side and more than 50% on the upwind side of the freeway. It should be noted that the P-trak to CPC ratio was lower when sampling at the downwind side of the freeways. Closer to the freeway, ultrafine particles are dominated by smaller particles usually below 50 nm. Those freshly emitted nanoparticle are known to have a large fraction of volatile components (CitationKittelson 1998), which may not be effectively detected by the P-trak instrument.
TABLE 1 Particle number concentrations measured by the CPC and P-trak. Standard deviation is given in parenthesis
and suggest that P-trak can be used to provide information on relative particle number concentrations for exposure studies. However, caution must be used when interpreting P-trak data. As seen in , there is a factor of 13 between CPC measured particle number concentration at 30 m downwind (western site) and 300 m upwind (eastern site) from the freeway. This factor of 13 became a factor of 4.3 if P-trak data were used. It is clear that P-trak underestimates particle number concentrations to a greater degree near sources. This suggests the performance of P-trak depends on particle size distributions.
Particle Size Effect
The effect of particle size distribution on P-trak performance during the first field campaign was examined in the following way. Two one-hour series of data were selected by examining particle size distribution graphs collected by the SMPS. The primary modes of the first one-hour series were between 30 and 40 nm, while the primary modes of the second one-hour series were between 10 and 20 nm. The first set of data represent larger particles than the second set of data. The manufacturer claims the P-trak is able to detect particles as small as 20 nm. Both CPC readings and CPC readings minus particles smaller than 20 nm diameter based on SMPS readings were compared with P-trak results. summarizes linear regressions results from this analysis. The slope for larger mode distribution was three times greater than smaller mode. The P-trak could only account for about 20% of CPC readings when sampling very small particles. The r 2 value for larger mode is also higher than for smaller mode. Subtracting SMPS readings for particles less than 20 nm only improved the r 2 for the larger mode group slightly and had little effect on the smaller mode group. These could possibly be due to two facts. First, the summation of particle number concentrations based on SMPS measurements was always lower than CPC readings. Although charging efficiencies and size dependent detection efficiencies inside the Electrostatic Classifier are corrected by the AIM software, there may be more losses that have not been accounted for and/or the charging may not be ideal Boltzmann equilibrium (CitationSioutas et al. 1999). In addition, the SMPS only measured a portion of particle size range (7–220 nm) that the CPC detects. Thus, the SMPS data do not account for all the particles larger than 220 nm that the CPC detected although these particles are unlikely to account for a large portion of the total CPC counts. Second, the indicated lower detection limit of P-trak, 20 nm, may be wrong. The P-trak may not be able to detect particles down to 20 nm, especially when those particles were freshly emitted from traffic sources.
TABLE 2 Linear regression results for different size distribution
Although the P-trak only measures a portion of particles that CPC does, the relatively high r 2 values (∼0.6 to ∼0.8) seem to suggest that proper correction factors could be applied to P-trak measurements to adjust for this discrepancy. presents P-trak to CPC ratios and geometric mean diameters (GMD) derived from SMPS measured particle size distributions (6 to 220 nm) as a function of distance from the freeway in the second field campaign. Error bars indicate one standard deviation. The P-trak to CPC ratios showed a consistent pattern versus geometric diameter on both sides of the freeway. Freshly emitted freeway aerosols have a much lower GMD compared to upwind aerosols. A factor of 0.25 seems to be appropriate to correct P-trak readings when sampling freshly emitted traffic aerosols. A factor of 0.75 may be used when sampling background aerosols. When sampling was conducted under mixed conditions, an intermediate value, 0.5, can be used to correct P-trak readings. Correction factors presented in could be used to correct P-trak readings if the distance to freeway is known.
The dependence of P-track to CPC ratios on GMD of the particle size distribution became even clearer when all the paired P-trak, CPC and SMPS data were pooled together and presented in . Error bars indicate one standard deviation. A sigmoid (S-shape) function was found to fit the observed data very well. The P-trak to CPC ratio levels off at 0.19 when the GMD was less than 20 nm and levels off at 0.96 when the GMD was greater than 80 nm. This represents the worst and best case in terms of P-trak performance. When particles are fresh and small, P-trak measures about 20% what CPC measures. When particles are aged and/or large, P-trak measures more than 90% what CPC measures. The fitted curve in could be used to correct for P-trak readings if the GMD of the aerosol is known.
FIG. 6 P-trak to CPC ratio vs. geometric mean diameter of the corresponding particle size distribution.
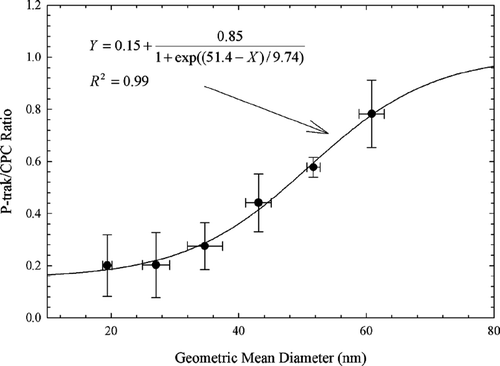
It is noted, that the P-trak may not be able to detect particles down to 20 nm as stated in its manual. The number of particles counted by a P-trak is the total number of particles larger than the minimum size to be activated, i.e.,
shows the relationship between determined P-trak activation size and the GMD of the corresponding aerosol. Error bars indicate one standard deviation. No strong function was observed. In general, the P-trak detects particles down to 25–30 nm regardless of the size distribution of the aerosol. It is clear from and , the sigmoid function between GMD and P-trak/CPC ratio is due to the fact that aerosols with smaller GMD have more particles below the P-trak's detection limit. Thus, P-trak is not recommended to sample aerosols where a large portion of particles is below 25–30 nm—fresh vehicular exhaust.
CONCLUSIONS
In general, P-trak works reasonably well for indoor environments. For outdoor environments, the P-trak and CPC instruments showed better agreement at background sites than at freeway influenced site. Higher correlations were observed between P-trak and CPC data for indoor measurements than outdoor. The highest P-trak and CPC correlation (r 2 = 0.9385) was detected inside the apartment that was located farther from the freeway than the other apartment. The poorest correlation occurred at 30 m downwind from the freeway. In that case, the P-trak reported about 25% of ultrafine particle concentration that the CPC did. Based on collected SMPS data, we identified two types of ultrafine particle size distributions, one with the dominant mode diameter at about 15 nm and the other at about 30 nm. Correlations between the P-trak and the CPC were better for the latter case. A sigmoidal function was found between P-trak to CPC ratios and geometric mean diameter derived from SMPS measured particle size distributions.
Overall, we concluded P-trak worked reasonably well when sampled indoor air. However, it has significant limitations in detecting freshly emitted ultrafine particles from vehicles. The P-traks underestimated total particle number concentrations especially for ultrafine particles with smaller geometric mean diameters. Caution must be given in interpreting data collected by P-traks near combustion sources.
The authors would like to thank professor Andrea M. Hricko and Ed Avol at University of Southern California for sharing the two P-traks. This work was supported by the Southern California Particle Center and Supersite: U.S. Environmental Protection Agency grant number R82735201, California Air Resources Board contract number 98-316, and the Southern California Environmental Health Center, National Institute of Environmental Health Sciences (NIEHS) Grant # 5 P30 ES07048-07.
REFERENCES
- Chan , C. C. , Chuang , K. J. , Shiao , G. M. and Lin , L. Y. 2004 . Personal Exposure to Submicrometer Particles and Heart Rate Variability in Human Subjects . Environ. Health. Perspect. , 112 : 1063 – 1067 . [INFOTRIEVE] [CSA]
- Hinds , W. C. 1999 . Aerosol Technology: Properties, Behavior, and Measurement of Airborne Particles, , 2nd Edition , New York : Wiley .
- Jaques , P. A. and Kim , C. S. 2000 . Measurement of Total Lung Deposition of Inhaled Ultrafine Particles in Healthy Men and Women . Inhal. Toxicol. , 12 : 715 – 731 . [INFOTRIEVE] [CROSSREF] [CSA]
- Kittelson , D. B. 1998 . Engines and Nanoparticles: A Review . J. Aerosol Sci. , 29 : 575 – 588 . [CROSSREF] [CSA]
- Matson , U. , Ekberg , L. E. and Afshari , A. 2004 . Measurement of Ultrafine Particles: A Comparison of Two Handheld Condensation Particle Counters . Aerosol Sci. Technol. , 38 : 487 – 495 . [CROSSREF] [CSA]
- Nemmar , A. , Hoet , P. H. , Vanquickenborne , B. , Dinsdale , D. , Thomeer , M. , Hoylaerts , M. F. , Vanbilloen , H. , Mortelmans , L. and Nemery , B. 2002 . Passage of Inhaled Particles into the Blood Circulation in Humans . Circulation , 105 : 411 – 414 . [INFOTRIEVE] [CROSSREF] [CSA]
- Nemmar , A. , Hoylaerts , M. F. , Hoet , P. H. M. and Nemery , B. 2004 . Possible Mechanisms of the Cardiovascular Effects of Inhaled Particles: Systemic Translocation and Prothrombotic Effects . Toxicol. Lett. , 149 : 243 – 253 . [INFOTRIEVE] [CROSSREF] [CSA]
- Pakkanen , T. A. , Kerminen , V. -M. , Korhonen , C. H. , Hillamo , R. E. , Aarnio , P. , Koskentalo , T. and Maenhaut , W. 2001 . Urban and Rural Ultrafine (PM0.1) Particles in the Helsinki Area . Atmos. Environ. , 35 : 4593 – 4607 . [CROSSREF] [CSA]
- Peters , A. , Wichmann , H. E. , Tuch , T. , Heinrich , J. and Heyder , J. 1997 . Respiratory Effects Are Associated with the Number of Ultrafine Particles . Am. J. Respir. Crit. Care Med. , 155 : 1376 – 1383 . [INFOTRIEVE] [CSA]
- Reponen , T. , Grinshpun , S. A. , Trakumas , S. , Martuzevicius , D. , Wang , Z. M. , LeMasters , G. , Lockey , J. E. and Biswas , P. 2003 . Concentration Gradient Patterns of Aerosol Particles near Interstate Highways in the Greater Cincinnati Airshed . J. Environ. Monit. , 5 : 557 – 562 . [INFOTRIEVE] [CROSSREF] [CSA]
- Sioutas , C. , Abt , E. , Wolfson , J. M. and Koutrakis , P. 1999 . Evaluation of the Measurement Performance of the Scanning Mobility Particle Sizer and Aerodynamic Particle Sizer . Aerosol Sci. Technol. , 30 : 84 – 92 . [CROSSREF] [CSA]
- Wichmann , H. E. and Peters , A. 2000 . Epidemiological Evidence of the Effects of Ultrafine Particle Exposure . Phil. Trans. R.Soc. Lond. A , : 2751 – 2769 . [CSA]
- Wichmann , H. E. , Spix , C. , Tuch , T. , Wolke , G. , Peters , A. , Herinrich , J. , Kreyling , W. G. and Heyder , J. 2000 . Daily Mortality and Fine and Ultrafine Particles in Erfurt, Germany, Part A: Role of Particle Number and Particle Mass: HEI report.
- Zhu , Y. , Hinds , W. C. , Kim , S. , Shen , S. and Sioutas , C. 2002a . Study of Ultrafine Particles near a Major Highway with Heavy-Duty Diesel Traffic . Atmos. Environ. , 36 : 4323 – 4335 . [CROSSREF] [CSA]
- Zhu , Y. , Hinds , W. C. , Kim , S. and Sioutas , C. 2002b . Concentration and Size Distribution of Ultrafine Particles near a Major Highway . J. Air Waste Manag. Assoc. , 52 : 1032 – 1042 . [INFOTRIEVE] [CSA]
- Zhu , Y. , Kuhn , T. , Mayo , P. and Hinds , W. C. 2006 . Comparison of Daytime and Nighttime Concentration Profiles and Size Distributions of Ultrafine Particles near a Major Highway . Environ. Sci. Technol. , [CSA]
- Zhu , Y. F. , Hinds , W. C. , Krudysz , M. , Kuhn , T. , Froines , J. and Sioutas , C. 2005 . Penetration of Freeway Ultrafine Particles into Indoor Environments . J. Aerosol Sci. , 36 : 303 – 322 . [CROSSREF] [CSA]