Abstract
An approach based on the calculation of the probabilities of the random displacements of particles around their regular trajectories enables us to derive analytical expressions for the normalized apparent mobility spectrum. A particular derivation is carried out for the case of a DMA with one collecting electrode, with inclined electric field, and variable electric field strength. Calculations show that an inclined electric field actually results in a sharper apparent spectrum, i.e., in a higher resolution than a transverse electric field does. A way to improve resolution by calculations is outlined.
INTRODUCTION
Differential Mobility Analyzer (DMA) has a wide use in the measurements of aerosol particle size distributions and, also, air ion mobility distributions. A comprehensive historical overview of this apparatus was composed by CitationFlagan (1998). A systematical approach to DMA can be found in CitationTammet (1970). An overview of contemporary electrical aerosol size spectrometers was published by Intra and Tippayawong (Citation2007, Citation2008). In general lines, a DMA has air inlet(s), air outlet(s), electrodes, and collector(s) of electric current or aerosol particles. Following the terminology of CitationTammet (1970), a DMA with a narrow inlet for aerosol and a narrow collector for aerosol particles can be called a second-order DMA. It is possible to design many different structures of DMA. For example, the DMA by CitationKnutson and Whitby (1975) has a divided air inlet and one collector at the air outlet. The electrical aerosol spectrometer of the University of Tartu has a divided air inlet and multiple collecting electrodes (CitationMirme et al. 1984; CitationTammet et al. 2002). CitationSalm (1995) proposed a DMA with a divided air inlet and multiple collectors at the air outlet; an analogous DMA was built by CitationBox and Collings (2007).
The Brownian motion of particles and turbulence are two of the factors that cause distortions in the measurements of mobility distributions by means of DMA. These processes may also be called molecular diffusion and turbulent diffusion, respectively. The diffusion distortions can be divided into two groups: particle losses in the entrance region of the analyzer, and the distortion of the shape of the mobility distribution. We will confine ourselves to the second phenomenon. The most essential diffusion distortion is the smoothing of the mobility distribution and the relevant reduction of resolution. The historical survey of the problem up to 2000 is given in CitationSalm (2000). Additional information to the survey can be found in the Introduction of the paper (CitationFlagan 1999). The diffusion of charged particles in nonuniform force field and the corresponding DMA resolution have been studied in CitationAlonso and Endo (2001) and CitationAlonso (2002). CitationMamakos et al. (2007) by numerical calculations investigated the validity of the semianalytical theory of DMA resolution by CitationStolzenburg (1988). CitationStolzenburg and McMurry (2008) have presented a summary of equations governing the responses of single DMA and TDMA systems including diffusion broadening of the DMA transfer function. Diffusion distortions in a simple DMA have systematically been studied also in CitationSalm (2000). Here we will demonstrate that the method developed in the above paper is applicable also for a more complicated DMA. In particular, we consider the design of a DMA with an inclined electric field, which has certain advantages with respect to the resolution (CitationLoscertales 1998; CitationTammet 1998; Citation1999). The principle of inclined velocities was developed in an analyzer IGMA by Tammet (Citation2002, Citation2003). In general, we will follow the methodology used in CitationSalm (2000).
APPARENT MOBILITY SPECTRUM
In an ideal DMA, a charged particle with fixed mobility moves along a regular trajectory, and reaches a certain end point. In reality, the Brownian motion of the particles exists, turbulence may take place, and the actual trajectories disperse randomly around the ideal trajectory; charged particles of single mobility reach various dispersed end points, as if a continuous mobility spectrum of finite width exists. Then an ordinary data processing algorithm calculated for ideal conditions yields an apparent mobility spectrum of finite width in place of a discrete line.
If diffusion is lacking, then the current strength through one collector or the characteristic of the analyzer is expressed by the integral
The characteristic I(ψ) is measured and a proper operator H ψ is applied to the characteristic to solve the Equation (Equation1) and to find the mobility spectrum ρ(Z):
The structure of the operator H ψ depends on the particular type of the mobility analyzer and on the choice of the variable parameter ψ. The subscript ψ →Z denotes that the variable parameter should be expressed by mobility.
Without restricting generality, the particles of a single mobility Z 1 (unimobile particles) may be considered only (the subscript 1 or the symbol Z 1 denotes this presumption). The current through a collector is then I 1. In the case of diffusion, Equations (1) and (2) can be expressed:
DMA WITH ONE COLLECTING ELECTRODE, WITH AN INCLINED ELECTRIC FIELD AND VARIABLE ELECTRIC FIELD STRENGTH
Description of the DMA
Let us consider a simplified second-order DMA, which is similar to that in CitationSalm (2000). The main difference is that the traverse electric field strength E is replaced by two components E x and E y, where E x is directed against the airflow u (). represents the DMA schematically; the technical structure can be designed in several shapes and is not discussed here.
The regular velocity of a charged particle is in general
The horizontal velocity of a charged particle in our DMA is
We will consider here the case E x∝E y. Let us express E x = kE y, where k is the coefficient of proportionality. Equation for the characteristic (limiting) mobility is replaced by a modified equation
Charged particles get to the collector, if the characteristic (limiting) mobility equals to the particle mobility Z 1.
Current Strength and Solving Algorithm
The following derivation of equations is quite similar to that in CitationSalm (2000), with understandable replacements. Let the electric field strength E y be the variable parameter. At first, the current strength through the collector I*1(E y) is to be calculated, and then the algorithm (4) is to be applied:
As in the above paper, the current strength I*1(Ey ) through the collector, which is proportional to the current density through the same collector, is calculated by kinematical approach based on the consideration of the regular trajectories and of the random walk of particles.
Let us consider unipolarly charged particles entering the DMA at a point (0,0) at a moment t = 0, and drifting to the collector, in average (see ). The current strength of the entering particles:
In general, the current density of particles is composed of a conduction component and of a diffusion component (CitationSalm 2000). The current density of the particles through the collector is determined by the y-components of corresponding vectors and by the charge density of particles in the vicinity of the collector:
The relative importance of the regular and random components of fluid motion may be expressed by the Peclet number
The Peclet number is an essential factor that determines the resolution of the DMA. We are considering the case of weak diffusion, i.e., the range of large Peclet numbers, Pe ≫ 1. In the case of weak diffusion, the deposition of particles on electrodes obviously has little effect upon the results of calculation (CitationSalm 2000). In the theory of Brownian motion, the deposition of particles is called absorption. In the present paper, we will neglect the effect of absorption.
The two-dimensional density of probability for finding an entered particle at a point (x, y) at a later moment t is expressed by two-dimensional Gaussian law, if we neglect the absorption on electrodes:
If we take into account only the regular term Z 1 E y ρ c in Equation (Equation10) in the vicinity of the collecting electrode, then the probability that an entered particle hits upon the collector at any time is
The current strength through the collecting electrode:
In order to specify the general algorithm (2) for the present DMA, we use the same way as in CitationSalm (2000). As a result, in the absence of diffusion, the algorithm (2) can be expressed as follows:
Normalized Apparent Spectrum
The normalized apparent spectrum can be expressed on the basis of Equations (8) and (16) as follows:
Substituting of I*1(Ey ) from Equation (Equation15) into Equation (Equation17) gives the normalized apparent spectrum explicitly:
The shape of the normalized apparent mobility spectrum is illustrated in . As it is seen in , an inclined electric field actually results in a sharper apparent spectrum, i.e., in a higher resolution according to the concept by CitationLoscertales (1998). The width of the spectrum essentially depends on the Peclet number. also clearly shows that the apparent spectrum is asymmetrical and that the peak is shifted towards lower mobility comparing with the actual mobility; this result was discovered by CitationSalm (1970).
FIG. 2 The solid line represents the function w*(Z, Z 1) in the case of Z 1 = 1.0; d = L; Pe = 20 and Ex = Ey . The dashed line shows the function with the exception Ex = 0, as in CitationSalm (2000).
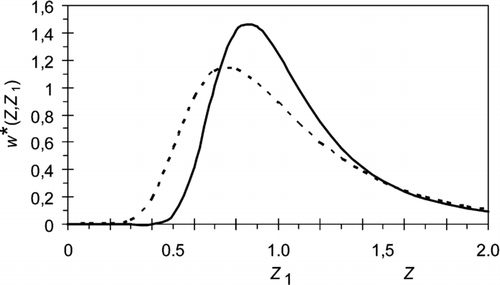
Simplification of Equations
At weak diffusion, the argument ζ of the function K0(ζ) is large, and we can use the asymptotic equation of this function:
Equation (Equation19) is sufficiently accurate also at moderate diffusion: the error is less than 3%, if ζ>5. Equation (Equation18) can asymptotically be expressed as follows:
Equation (Equation20) represents an explicit analytical function composed of elementary functions. At the limit of weak diffusion, a subsequent simplification of the equation is possible. As in CitationSalm (2000), the expression under square root symbol and the same under fourth root symbol can be transformed algebraically, and Equation (Equation20) is then expressed as follows:
Equation (Equation21) has the same shape as Equation (30) in CitationSalm (2000). However, here the variable a has somewhat different expression, depending on the ratio k. In the limit k→0, the expression approaches to that in CitationSalm (2000).
At weak diffusion only small deviations of Z around Z 1 are essential. Then b≪a and by means of geometrical considerations as related to in (CitationSalm 2000) it is possible to prove that
Then Equation (Equation21) can be transformed as
Thus we have obtained a quite simple analytical equation for the description of the effect of diffusion on mobility spectrum measurements by a particular DMA. The normalized apparent spectrum according to Equation (Equation24) has a certain affinity to the Gaussian curve, but shows asymmetry. The mode of mobility is shifted toward lower mobilities. The affinity to the Gaussian curve becomes better noticeable, if a new variable 1/Z is introduced. The exact Gaussian curve with respect to 1/Z is obtained, if we consider only small deviations of Z in the vicinity of Z 1, i.e., Z≈Z 1. Then
The mean value of the variable 1/Z is then 1/Z 1, and the standard deviation is
The factor has appeared here in comparison with Equation (38) in CitationSalm (2000). This factor expresses the effect of inclined electric field.
The standard deviation of the variable 1/Z can be used for the expression of the resolution of mobility spectrometers (CitationSalm 2000). The resolution parameter can in general be expressed as follows Salm (Citation1983, Citation2000) and CitationZhang and Flagan (1996):
Equations (26) and (28) are in accordance with relevant estimations in CitationLoscertales (1998) and CitationTammet (1999), which were derived on the basis of a relatively rough diffusion model. Here we have seen that several steps of simplification are necessary in order to obtain the estimations.
The knowledge of the analytical apparent spectrum opens a way to the improvement of resolution by calculations. This idea was first published by CitationSalm (1970) and is summarized as follows. If the normalized apparent spectrum w*(Z,Z 1) is known, then the apparent spectrum ρ*(Z) for any general case is expressed as
Here we consider only diffusion distortions. In many cases the apparent spectrum w*(Z,Z 1) depends on the ratio Z/Z 1. This is the case also in Equations (18), (20), and (24). Then, using a simple exponential transformation, it is possible to express Equation (Equation29) in the shape of convolution, and to solve the convolution equation by means of the Fourier transform. Thus the mobility spectrum ρ(Z) can be found with an enhanced resolution.
CONCLUSIONS
An approach based on the calculation of the probabilities of the random displacements of particles around their regular trajectories enables to derive analytical expressions for the normalized apparent spectrum. A particular derivation is carried out for the case of a second-order DMA with one collecting electrode, an inclined electric field and variable electric field strength. Equation (Equation18) represents the most exact explicit equation for the normalized apparent spectrum in this study. Analogously as in CitationSalm (2000), a simplification of Equation (Equation18), and the derivation of explicit analytical expressions of various approximation degrees for the normalized apparent spectrum are possible. Sufficiently simplified equations are in accordance with relevant estimations in CitationLoscertales (1998) and CitationTammet (1999), which were derived on the basis of a relatively rough diffusion model. The knowledge of the analytical apparent spectrum opens a way to the improvement of resolution by calculations.
Acknowledgments
This research was in part supported by the Estonian Science Foundation through grants 6223 and 6988, and the Estonian Research Council Targeted Financing Project SF0180043s08.
REFERENCES
- Alonso , M. 2002 . Reducing the Diffusional Spreading Rate of a Brownian Particle by an Appropriate Non-uniform External Force Field . J. Aerosol Sci. , 33 : 439 – 450 .
- Alonso , M. and Endo , Y. 2001 . Dispersion of Aerosol Particles Undergoing Brownian Motion . J. Phys. A: Math. Gen , 34 : 10745 – 10755 .
- Box , S. and Collings , N. 2007 . A Multi-Channel Electrical Mobility Spectrometer with Wedge Geometry—Design and First Evaluation . J. Aerosol Sci. , 38 : 656 – 673 .
- Flagan , R. C. 1998 . History of Electrical Aerosol Measurements . Aerosol Sci. Technol. , 28 : 301 – 380 .
- Flagan , R. C. 1999 . On Differential Mobility Analyzer Resolution . Aerosol Sci. Technol. , 30 : 556 – 570 .
- Intra , P. and Tippayawong , N. 2007 . An Overview of Aerosol Particle Sensors for Size Distribution Measurement . Maejo J. Sci. Tech. , 01 ( 02 ) : 120 – 136 .
- Intra , P. and Tippayawong , N. 2008 . An Overview of Differential Mobility Analyzers for Size Classification of Nanometer-Sized Aerosol Particles . Songklanakarin J. Sci. Technol. , 30 ( 2 ) : 243 – 256 .
- Knutson , E. O. and Whitby , K. T. 1975 . Aerosol Classification by Electric Mobility Apparatus, Theory and Applications . J. Aerosol Sci. , 6 : 443 – 451 .
- Loscertales . 1998 . Drift Differential Mobility Analyzer . J. Aerosol Sci. , 29 : 1117 – 1139 .
- Mamakos , A. , Ntziachristos , L. and Samaras , Z. 2007 . Diffusion Broadening of DMA Transfer Functions. Numerical Validation of Stolzenburg Model . J. Aerosol Sci. , 38 : 747 – 763 .
- Mirme , A. , Noppel , M. , Peil , I. , Salm , J. , Tamm , E. and Tammet , H. 1984 . Multi-Channel Electric Aerosol Spectrometer . 11th Int. Conf. on Atmospheric Aerosols, Condensation and Ice Nuclei, Budapest , 2 : 155 – 159 .
- Salm , J. 1970 . The Apparent Spectrum of Air Ions Due to Heat Diffusion (in Russian; summary in English) . Acta et comm. Universitatis Tartuensis , 240 : 164 – 173 .
- Salm , J. 1983 . “ The Effect of Turbulent and Molecular Diffusion in the Spectrometer of Air Ions ” . In Proceedings in Atmospheric Electricity , Edited by: Ruhnke , L. H. and Latham , J.A. 61 – 64 . Hampton : Deepak Publishing .
- Salm , J. 1995 . A Modification of the Electrical Analyzer of Charged Aerosol Particles . Aerosols, Moscow , 1 : 12 – 12 .
- Salm , J. 2000 . Diffusion Distortions in a Differential Mobility Analyzer: The Shape of Apparent Mobility Spectrum . Aerosol Sci. Technol. , 32 : 602 – 612 .
- Stolzenburg , M. 1988 . An Ultrafine Aerosol Size Distribution Measuring System , MN : University of Minnesota . Ph.D. Thesis
- Stolzenburg , M. and McMurry , P. H. 2008 . Equations Covering Single and Tandem DMA Configurations and a New Lognormal Approximation in the Transfer Function . Aerosol Sci. Technol. , 42 : 421 – 432 .
- Tammet , H. 1970 . The Aspiration Method for the Determination of Atmospheric-Ion Spectra , Jerusalem : Israel Program for Scientific Translations .
- Tammet , H. 1998 . Optimal Length of the Plain Loscertales Mobility Analyzer. . J. Aerosol Sci. , 29 : S63 – S64 .
- Tammet , H. 1999 . The Limits of Air Ion Mobility Resolution . Proc. 11th Int. Conf. Atmos. Electr. , : 626 – 629 . Alabama (NASA, MSFC)
- Tammet , H. 2002 . Inclined Grid Mobility Analyzer: The Plain Model. . Abstracts of Sixth International Aerosol Conference , 2 : 647 – 648 .
- Tammet , H. 2003 . Method of Inclined Velocities in the Air Ion Mobility Analysis . Proc. 12th Int. Conf. Atmos. Electr., Versailles , : 399 – 402 .
- Tammet , H. , Mirme , A. and Tamm , E. 2002 . Electrical Aerosol Spectrometer of Tartu University . Atmospheric Research , 62 : 315 – 324 .
- Zhang , Shou-Hua and Flagan , R. C. 1996 . Resolution of the Radial Differential Mobility Analyzer for Ultrafine Particles . J. Aerosol Sci. , 27 : 1179 – 1200 .