Abstract
Large increases in concentration of particles smaller than 20 nm diameter are relatively common over the central Arctic Ocean in summer and have occasionally been observed over lower latitude oceans. These events often do not readily fit theoretical models of homogeneous nucleation from known precursor gases. It is shown that aggregates and gels of marine biological origin are often common over remote oceans and have a partially granular structure. Previous work in the central Arctic Ocean in summer has shown evidence of release of particles of the order of 40 nm diameter in the presence of evaporating fogs. It is suggested here that under some circumstances disintegration of the primary particles may be more complete, releasing particles smaller than 5 nm that would then be mistaken for recently nucleated particles. Examination of particles present during an apparent nucleation event at Cape Grim, Tasmania supports this interpretation. Correlation coefficients of concentrations of particles of different sizes during the period before apparent nucleation events suggests a distinct difference between vigorous true nucleation events and those over the central Arctic Ocean in summer where little subsequent growth is observed.
1. INTRODUCTION
Nucleation has been found to occur frequently in some coastal areas such as Mace Head, Ireland, the current theory being that oxidation of iodine compounds released from marine organisms exposed at low tide leads to the nucleation of aerosols (CitationO'Connor et al. 2008). There have also been cases of non-tidally related enhancements of nucleation mode particles (e.g., CitationLeck and Bigg 2008; Modini et al. 2009). Over remote low and mid-latitude oceans particles < 20 nm diameterFootnote* occur infrequently (CitationHeintzenberg et al. 2004) but over the central Arctic Ocean in summer many cases have been observed. CitationCovert et al. (1996) found a continuous presence of particles in the 2.7 to 5 nm size range and a sporadic occurrence of an “ultrafine” particle mode occurring anywhere between 3 and 20 nm diameter, separated from the 25–80 nm Aitken mode. CitationWiedensohler et al. (1996) concluded that the ultrafine mode was produced by nucleation in the upper layers of the marine boundary layer and mixed down and that the precursor gases were produced over the open ocean. It is now generally considered that formation of new particles from homogeneous nucleation, either binary sulphuric acid (H2SO4) and water vapor (H2O), or binary or tertiary involving methane sulphonic acid (MSA), H2O, H2SO4, or ammonia (NH3) is unlikely to be common over the ocean (CitationKulmala et al. 1998; Vehkamäki et al. 2002; CitationKreidenweis and Seinfeld 1988; CitationBall, et al. 1999; CitationKorhonen et al. 2003; CitationNapari et al. 2002). However, nucleation and growth continues to be the generally accepted mode of formation of ultrafine particles. A direct demonstration that this is the route has yet to be given not only over the pack-ice region of the Arctic Ocean, where no particles smaller than 50 nm showed a detectable presence of H2SO4 (CitationLeck and Bigg 1999), but also elsewhere over remote marine areas.
CitationLeck and Bigg (1999) reported a nucleation event not followed by growth. Their showed enhanced concentrations first of ∼ 30 nm particles, then of ∼ 7 nm and finally < 5 nm. This sequence of decreasing sizes with time was common. They used the boundary layer structure and the vertical distribution of particles during nucleation events to show that if the ultrafine and Aitken particles did come from above, it was from a level < 250 m above the surface and not from the free troposphere. The most vigorous episodes of < 5 nm particle enhancements were associated with rapid dissipation of intermittent fogs. A subsequent study by CitationHeintzenberg et al. (2006) using aerosol size distribution data taken during two expeditions to the high Arctic in the summers of 1996 (CitationLeck et al. 2001), and 2001 (CitationLeck et al. 2004) showed strong indications of fog-related aerosol production particularly in the Aitken mode size range. CitationLeck and Bigg (1999) speculated that electrical charge on fog drops could cause explosive ejection of included material as the fog drops evaporated. DeFelice and Cheng (1998) have provided another explanation of particle production following rapid evaporation of fog or cloud based on the way in which dissolved material crystallizes during evaporation.
FIG. 1 Granular structures having a broad size range linked by electron-transparent gel material in the surface microlayer of the central Arctic Ocean.
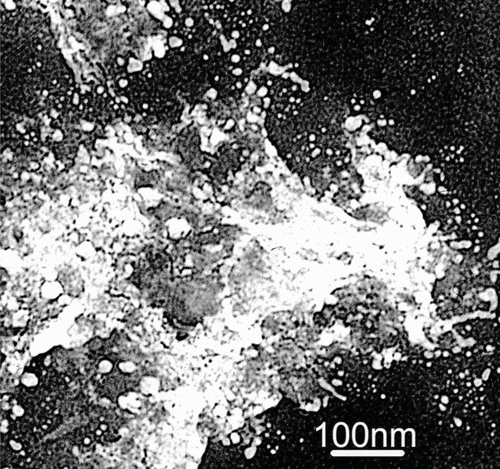
The possible significance of the very large numbers of granules a few nanometers in diameter within many marine organic particles was not fully realized by CitationLeck and Bigg (1999), who concentrated instead on the possibility of production and release from the particles of precursor gases for nucleation. Here a case will be presented that ejection of the granules or clumps of them, from the parent particles could lead to the observed production of Aitken, ultrafine and < 5 nm particles. Processes that might be involved will be considered.
2. METHODS
2.1. Aerosol Collection and Visualization
Direct collection of particles onto transmission electron microscope (TEM) 3 mm formvar-coated copper grids was used in all cases both in the Arctic and at lower latitudes. The collection efficiency of the electrostatic precipitator used was inter-compared with the TSI 3089 Nanometer Aerosol Sampler. Collection efficiency for particles smaller than 25 nm was low in both and such particles would only be readily detected when concentrations were high. Three different impactors were used, with nozzle diameters of 1 mm, 100 μ m, and 70 μ m. Estimated 50% collection efficiency cut-points were about 200 nm, 70 nm, and 50 nm. The characteristics of the impactors and electrostatic precipitators used have been described in many papers, the most recent being CitationLeck and Bigg (2008). Shadowing the specimens with platinum at an oblique angle was used in much of the early work in order to deduce three-dimensional shape and as accurate a size as possible. However, this procedure inevitably hides very fine structure in the particles and in recent collections specimens were deliberately left unshadowed to reveal their fine structure. In all TEM photographs in this paper, the brighter areas represent greater electron-density. The TEMs used were Sydney University's Electron Microscope Unit (EMU) Philips CM12 and Stockholm University's Chemistry Department JEOL-2000FX TEM. X-ray analyzers attached to each of these microscopes were used to detect inorganic components in selected specimens. Sydney University's EMU field transmission microscope JEOL-3000F was used for the smallest detectable particles (section 4) in conjunction with a high resolution X-ray spectrometer.
2.2. Number Size Distributions or Concentrations
Number size distributions at 10 min intervals were available for each of the sites mentioned except Cape Grim and used conventional differential mobility particle sizing (DMPS) systems. Details of the Arctic Ocean experiments DMPS for example have been given by CitationCovert et al. (1996), CitationBirmili (1999), CitationLeck et al. (2001), and CitationHeintzenberg et al. (2006). Those used on the Arctic Ocean expeditions and Hyytiälä Finland (CitationDal Maso et al. 2005) had lower limits of detection of about 3 nm. At Cape Grim, new particle events were detected by the difference between concentrations recorded by a TSI3025 counter (total concentration >3 nm) and a TSI 3010 counter (total >10 nm).
3. GRANULAR STRUCTURES WITHIN MARINE AND AIRBORNE BIOLOGICAL PARTICULATES
3.1. Marine Biological Particulates in the Surface Ocean
Wells and Goldberg (1991, 1993, 1994) were the first to note the presence of high concentrations, typically of the order of 109 ml –1, of particles predominantly < 50 nm in diameter, which they called “marine microcolloids.” Many of them appeared to be aggregates or granular structures of particles as small as 2 nm. A “mucus-like” or gel-like material was found to join the microcolloids. CitationDecho (1990) and CitationChin et al. (1998) recognized the microcolloids and their gels to be exopolymer secretions (EPS) produced by bacteria, algae and other organisms. EPS are water insoluble, thermally stable, highly surface-active, highly hydrated (99% water), and readily sequester dissolved organic matter. EPS and the gels derived from them consist mainly of polysaccharides that bind amino acids proteins and peptides, and range from tight aggregated capsules to the loose-slime matrix associated with the aggregates or granular structures. Divalent ions are thought to provide bridges between adjacent or different polysaccharide chains to give the gel-like consistency (CitationChin et al. 1998). According to CitationVerdugo et al. (2004), the marine gels span the whole size spectrum from colloidal-size nanogels containing single macromolecules entangled to form single-chain networks to micrometer-size gels that can aggregate to several 100s μ m; while most gel-forming biopolymers are macromolecular colloids.
CitationBigg et al. (2004) collected particles from the surface microlayer of the open leads of the Arctic pack ice area and shows a sample illustrating a granular structure including the presence of particles < 10 nm and the filmy material holding them together.
3.2. Airborne Marine Biological Particulates
Leck and Bigg (2005, 2008) and Bigg (2007) have illustrated the airborne gels with granular structures, which clearly originate from the surface microlayer of the ocean. Some additional examples having large amounts of gel enclosing smaller granules are shown in . At higher magnification, there are electron-dense patches down to about 2 nm in size. They were stable to heating and vacuum in the TEM.
FIG. 2 Gel covered granular structures or aggregates. Left to right: from the Arctic Ocean, 89°N, 2°W, Southern Ocean, 54°S, 90°E, Cape Grim, Tasmania, 41°S, 145°E, and Lizard Island, 15°S, 145°E.
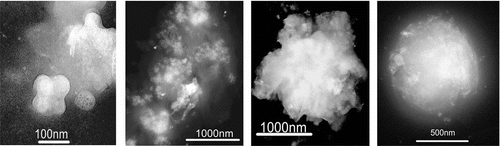
Granules within the gel material are most easily seen when the layer is thin. Bacteria recently injected into the atmosphere have a thin layer of this material on their surface that withstands the vacuum of the TEM, unlike those that have spent longer in the atmosphere which shrivel and then have no visible surface layer. shows such a bacterium and the granules are clearly visible in the enlargement of its head in .
FIG. 3 (a) a bacterium collected over the Southern Ocean and (b) enlargement of the head portions showing granules in the transparent material coating it.
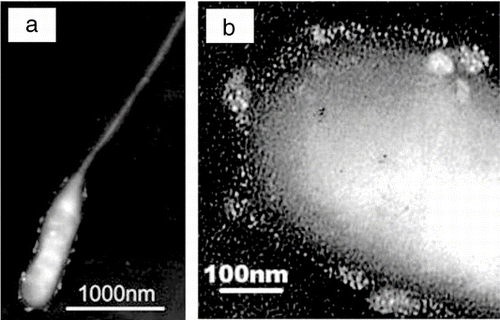
Self-assembled polymer gels disperse under the influence of ultraviolet light (CitationOrellana and Verdugo 2003) and undergo volume-phase transition upon acidification (CitationChin et al. 1998). Some examples of the latter are shown in , , , . These pictures appear to show the skeletons from which the trapped water and organic molecules has escaped the polymer networks linked by calcium ions. is a magnified portion of and shows the very large number of nanometer sized grains that have spilled from the parent particle. This was an unshadowed specimen. Where metal shadowing has been used the grain size of the shadowing material can disguise the fine structure within the gels. Collections made at the Maldives Climate Observatory Hanimaadhoo, during the summer monsoon (June through September) with onshore flow from the remote Indian Ocean, showed very large numbers of the condensed phase particles ( and , shadowed lightly), most of which were surrounded by halos of droplets typical of sulphuric acid. From the earliest days of the TEM technique this halo structure was recognized and identified (CitationBigg 1980). Other strong acids, notably phosphoric acid, also produce similar halos but the only one likely to be found in the atmosphere is methane sulphuric acid, which gives flatter droplets. Skeletal gels without any visible grains attached also occur, implying that the processes of separation under the microscope also occur in the atmosphere.
4. GELS ASSOCIATED WITH AN APPARENT NUCLEATION EVENT
During a field campaign aimed at understanding the properties of the aerosol at the Cape Grim atmospheric monitoring station several unusual events occurred simultaneously between 08 h and 16 h on December 8, 2007. A front had passed the station early on 7 December and baseline conditions (total particle concentrations < 700 cm–3) with westerly winds were established by 11 h UTC with wind speeds initially of 15 ms–1, dying away to about 5 ms–1 by midnight and to 3 ms–1 by 08 h on 8 December. The air trajectory at that time lay to the west and had not touched land for more than five days. The sea was calm with little swell and it was covered with large patches of persistent white foam visible as far as the horizon to an extent not seen even when wave action was extreme (). Note the low clouds that evaporated during the morning. The difference between two particle counters' readings, recording total particle concentrations >3 nm and >10 nm, respectively, rose to a maximum, then subsided as shown in indicating the presence of particles < 10 nm. Concentration of particles >10 nm were about 700 cm–3 at the beginning and end of the period and only reached above 1000 cm–3 for four separate 10 min intervals.
FIG. 5 (top) Extensive areas of persistent foam on the sea at Cape Grim, 08 h 8 December 2007. (bottom) Difference in concentrations recorded by TSI3025 (UCN) and TSI3010 (CN) particle counters between 08 and 16 h UTC on 8 December2007 at Cape Grim, Tasmania.
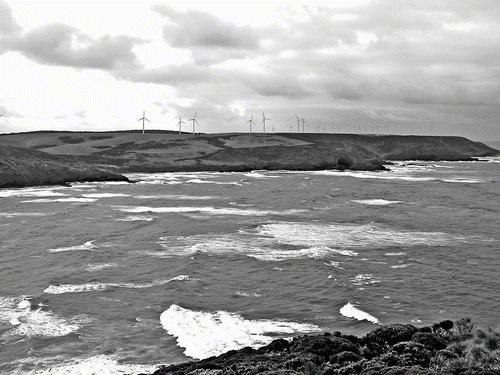
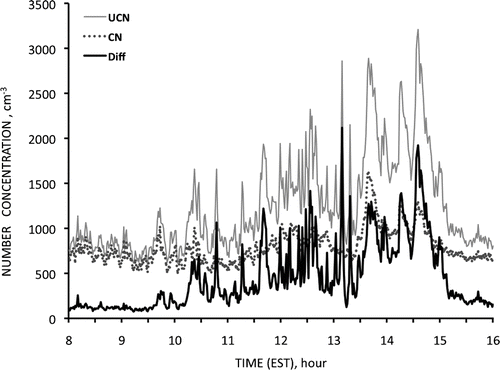
Throughout this period Z. Ristovski (Queensland University of Technology) was operating a volatility and hygroscopic tandem differential mobility analyzer (VH-TDMA). He reports (personal communication): “Particles with diameters of the order of 50 nm were an external mixture of two categories—those with thermal and hygroscopic properties consistent with sea salt hygroscopic growth factors (HGF) >2 and do not decompose up to 300°C) and those with an ammonium sulfate composition (HGF ∼ 1.6, decompose above 170°C).” At the same time two impactors (one operating at low pressure with a 70 μ m nozzle to collect small particles) and an electrostatic precipitator, were collecting aerosol particles.
TEM photographs of particles of the order of 50 nm did not appear consistent with any previously identified as sea salt and ammonium sulfate. However, they strongly resemble portions of the microlayer particle of . Those in show central portions consisting of aggregates of particles of about 5 nm held in a transparent matrix. The two on the right are surrounded by a multitude of individual nanometer-sized particles. Their central portions consisted of aggregates of particles of about 5 nm held in a transparent matrix.
The nature of particles like these (Aitken mode or smaller) was checked using a high resolution X-ray spectrometer attached to a powerful field emission TEM. lists the elements detected and the relative strengths of the elemental signatures.
TABLE 1 Peak heights of X-ray signal as a percentage of the carbon peak for 6 particles 40–80 nm diameter
Time limitations allowed only six particles to be analyzed. The formvar substrate provided a consistent carbon reference signal and peak heights of the X-ray responses are given as a percentage of it in . Calcium is clearly the dominant element detected, the remainder also being those that are consistent with a biological origin. Sea salt might have been expected to be present but sodium was not detected in any particle, although a weak signal may have been masked by its proximity to the copper K-α line due to the copper supporting grid. That chlorine was much more strongly represented than sodium is consistent with the osmotic function of the EPS and the affinity of the polysaccharides for chlorine resulting in a relatively large excess of chlorine compared with sea salt ratios of the ocean (CitationDecho 1990). The near-absence of sodium in the TEM-samples suggests that little, if any sea salt was present.
The important question is whether incorporation of particles like those of into the overlying low clouds and subsequent evaporation of the cloud drops could have led to the apparent nucleation event of . This will be considered further in section 6.
5. CORRELATION MATRICES AND NUCLEATION EVENTS
Suppose the correlation coefficients r of number concentration of particles with diameters d1 and d2 between times t a and t b are calculated for all d1 and d2 and presented in the form of color-coded contours of r on the d1–d2 plane. To see the correlation between specific diameters, look at the color code at the intersection of d1 and d2 with the diagonal. (Note that the portion below the diagonal is simply a mirror image of that above it and may be disregarded.) The time span used should be long enough to encompass about 20 measurements or more but not so long that changes in air mass are likely to have occurred and should only include periods of increasing total concentration because the growth phase where nucleation has occurred will affect the correlations.
Nucleation events are usually associated with a reduction in particle surface area, so the common form of the correlation matrix (CM) will be that of (Hyytiälä, Finland, 5 April 2002). Here particles having diameters within the accumulation (80–500 nm, Covert et al. 1996) and Aitken modes are well-correlated as are those within the nucleation mode, but the two regions are separate, showing separate sources. Anti-correlation with the nucleation mode is a maximum for diameters near 70 and 300 nm, which provided most of the particulate surface area. Total concentrations of particles rose rapidly from a minimum of 2700 cm–3 before the event to >20,000 cm–3 at the end of the selected period.
FIG. 6 Aggregates of nanometer-sized particles at the time of an apparent nucleation event at Cape Grim, Tasmania on the morning of 8 December 2007.
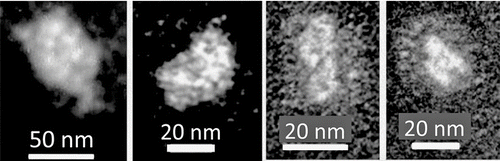
Concentrations of nucleation mode particles were far less in the pack ice region of the central Arctic Ocean, rarely exceeding 1000 cm–3 and there were few cases of obvious growth. The correlation matrices were never as simple as that of and showed complex regions of positive and negative correlations. These can be interpreted in terms of fragmentation of particles. Suppose for example that particles with predominant diameters of 200 nm split into 50 nm units. A negative correlation between concentrations of the two particle sizes will result. If the 50 nm particles further separate into 5 nm particles the correlation between concentrations of 50 and 5 nm particles may be either positive or negative, depending on whether the former continue to increase in concentration, or to decrease. If separation from the 200 nm particles into 5 nm particles is a continuous process, there will be on the CM strips of positive or negative correlations sloping from larger to smaller sizes. shows features consistent with both of these processes. Note the surprising fact that correlation coefficients are very strongly negative or positive within some very limited size ranges.
6. DISCUSSION
What we have shown is that there is a potential for particles of marine biological origin to separate into fragments having sizes within the nucleation mode. The nature of the Aitken mode particles seen in at the time of an apparent nucleation event and the correlation matrix of certainly suggest that this might happen but proof is lacking. The tendency noted in the Introduction for concentrations of larger particles to be enhanced before small ones in Arctic apparent nucleation events would be expected from a progressive stepwise fragmentation of large particles. It could be explained in terms of mixing of air from different levels or source regions having different aerosol size distributions but would require more complex assumptions. A similar hypothesis could be assumed to explain the patches of positive and negative correlation in but the correlation coefficients were so high in such limited size ranges that it seems unlikely. New particle formation in the Arctic associated with transient fogs could again be ascribed to mixing events that caused the fogs to dissipate but as in the other cases fragmentation represents a simpler solution.
CitationLeck and Bigg (1999) suggested that disruption of particles by electric charge might provide an appropriate fragmentation mechanism. It appears consistent with observation since it would be favored by evaporation of cloud or haze drops and needs further elaboration.
If the charge Q on a spherical drop of radius r at which electrical stress balances surface tension (known as the “Rayleigh limit”) is exceeded, the drop explosively ejects material and loses some charge. The Rayleigh limit is given by Q = 64π2ϵ σ r3 where ϵ is the permittivity of free space and σ is the surface tension. For a 20 μ m diameter cloud drop this is about 4.107 elementary charges (e) but for 200 nm it is only about 4000e. Electrical charge on cloud drops varies very widely and increases rapidly with drop size. Pruppacher and Klett (1998) summarize the observations (their figure 18-1) and give mean charge on 20 μ m drops as about 200 e for warm cloud, and more than a factor of 10 higher for cumulus clouds. During evaporation of a drop charge is not lost but the Rayleigh limit would not usually be reached until its size became smaller than that of a typical marine gel. Widmann et al. (1997) cite cases of disruption of organic liquids at 3% of the Rayleigh limit. Highly surface active gels embedded in a drop may not only yield an effective surface tension considerably less than that of pure water as the drop evaporates but may provide a shape factor that reduces stability. A drop containing a marine gel may also behave more like an organic liquid because of the high liquid content of EPS networks. The process is thus marginal but not impossible.
Measurement of the range of electrical charge on fog or cloud drops associated with apparent nucleation events could yield insights into the possibility that Rayleigh disintegrations were responsible.
If Rayleigh explosions occur at all, a prime place for them would be at the top or outflow from marine cumulus clouds, or in regions where evaporating cloud drops occur. There have been a number of observations of apparent particle production in such circumstances (e.g., CitationHegg et al. 1990; CitationPerry and Hobbs 1994; CitationClarke et al. 1998a, Citation1998b). The latter is a particularly relevant case because it occurred near the ocean surface in the presence of evaporating cloud drops and showed simultaneous increases in particle concentrations from the nucleation mode to about 20 nm and at about 50 nm. The Cape Grim case of an apparent nucleation event below low cloud discussed in section 4 seems to be similar to these and the nature of the Aitken mode particles seems to favor fragmentation as a cause. The discrepancy between the nature of the Aitken particles deduced from their thermal and hygroscopic properties and from their physical and chemical properties in that case is of considerable importance. It suggests that the fragments of marine gels when recently injected into the atmosphere are both highly hygroscopic and refractory. Their hydrophilic nature has long been known and the fact that they can withstand the vacuum of an electron microscope and the heating from the electron beam without visible alteration suggests a degree of thermal stability. The calcium-based structure of the network may contribute to this.
In addition to the hypothetical Rayleigh explosion process for fragmentation of marine gels, the physical and chemical changes that occur as a result of acidification, ultraviolet light and bacterial enzymes need to be considered as contributors to fragmentation. Acidification through liquid-phase sulphate production from dissolved sulphur dioxide will usually occur in fog drops. If it proceeds as far as the phase transition pH of 4.5, proteolysis that breaks protein structures into soluble products such as peptides and amino acids will follow and the granular structural integrity of aggregates would be destroyed. Cleavage of EPS molecules to short-chain polymers through the effects of multiple UV scattering in fog could aid the disintegration.
7. CONCLUSIONS
The fine structure of marine biological particles both in the water and air has been amply demonstrated. It gives rise to the possibility that in special circumstances, particularly their involvement in evaporating cloud or fog, their fragmentation could give rise to particles small enough to be confused with particles produced by nucleation of a supersaturated gas. The suggested causes of fragmentation appear possible but remain unproven and are an obvious but difficult target for further research.
Acknowledgments
Thanks are due to the Cape Grim Baseline Air Pollution Station staff, especially to the Director, J. Cainey for support at the Station and for financial support with the electron microscopy. We are very grateful to A. Öhrström for providing the analyses of the TEM-samples collected at the Maldives Climate Observatory Hanimaadhoo. I. Kaplin and S. Bulcock (Sydney University's Electron Microscope Unit) provided expert assistance with the two microscopes and associated X-ray equipment. I would also like to thank Z. Ristovski for permission to quote the results from his VH-TDMA.
Notes
*In this article, all particle sizes refer to diameters.
REFERENCES
- Ball , S. M. , Hanson , D. R. , Eisele , F. and McMurry , P. H. 1999 . Laboratory Studies of Particle Nucleation: Initial Results for H2SO4, H2O, and NH3 Vapors . J. Geophys. Res. , 104 : 23,709 – 23,718 .
- Bigg , E. K. 1980 . Comparison of Aerosol at Four Baseline Atmospheric Monitoring Stations . J. Appl. Meteorol. , 19 : 521 – 533 .
- Bigg , E. K. , Leck , C. and Tranvik , L. 2004 . Particulates of the Surface Microlayer of Open Water in the Central Arctic Ocean in Summer . Marine Chem. , 91 : 131 – 141 .
- Birmili , W. , Stratmann , F. and Wiedensohler , A. 1999 . Design of a DMA–Based Size Spectrometer for a Large Particle Size Range and Stable Operation. J . Aerosol. Sci. , 30 : 549 – 553 .
- Chin , W.-C. , Orellana , M. V. and Verdugo , P. 1998 . Spontaneous Assembly of Marine Dissolved Organic Matter into Polymer Gels . Nature , 391 : 568 – 572 .
- Clarke , A. D. , Varner , J. L. , Eisele , F. , Mauldin , R. L. , Tanner , D. and Litchy , M. 1998a . Particle Production in the Remote Marine Atmosphere: Cloud Outflow and Subsidence during ACE 1 . J. Geophys. Res , 103 ( D ) : 16397 – 16409 .
- Clarke , A. D. , Davis , D. , Kapustin , V. N. , Eisele , F. , Chen , G. , Paluch , I. , Lenschow , D. , Bandy , A. R. , Thornton , D. , Moore , K. , Mauldin , L. , Tanner , D. , Litchy , M. , Carroll , J. , Collins , M. A. and Albercook , G. 1998b . Particle Nucleation in the Tropical Boundary Layer and its Coupling to Marine Sulfur Sources . Science , 282 : 89 – 92 .
- Covert , D. S. , Wiedensohler , A. , Aalto , P. , Heintzenberg , J. , McMurry , P. H. and Leck , C. 1996 . Aerosol Number Size Distribution from 3 to 500 nm Diameter in the Arctic Marine Boundary Layer during Summer and Autumn . Tellus , 48B : 197 – 212 .
- Decho , A. W. 1990 . Microbial Exopolymer Secretions in Ocean Environments: Their Role(s) in Food Webs and Marine Processes . Oceanogr. Mar. Biol. Ann. Rev. , 28 : 73 – 153 .
- Dal Maso , M. , Kulmala , M. , Riipinen , I. , Wagner , R. , Hussein , T. , Aalto , P. P. and Lehtinen , K. E. J. 2005 . Formation and Growth of Fresh Atmospheric Aerosol: Eight Years of Aerosol Size Distribution Data from SMEAR II Hyytiälä Finland . Boreal Environ. Res. , 10 : 323 – 336 .
- Hegg , D. A. , Radke , L. F. and Hobbs , P. V. 1990 . Particle Production Associated with Marine Clouds . J. Geophys. Res , 95 : 13,917 – 13,926 .
- Heintzenberg , J. , Birmili , W. , Wiedensohler , A. , Nowak , A. and Tuch , T. 2004 . Structure, Variaility and Persistence of the Submicrometer Marine Aerosol . Tellus , 56B ( 4 ) : 357 – 267 .
- Heintzenberg , J. , Leck , C. , Birmili , W. , Wehner , B. , Tjernström , M. and Wiedensohler , A. 2006 . Aerosol Number-Size Distributions during Clear and Fog Periods in the Summer High Arctic 1991, 1996 and 2001 . Tellus , 58B : 41 – 59 .
- Korhonen , H. , Lehtinen , K. E. J. , Pirjola , L. , Napari , I. , Vehkamki , H. , Noppel , M. and Kulmala , M . 2003 . Simulation of Atmospheric Nucleation Mode: A Comparison of Nucleation Models and Size Distribution Representations . J. Geophys. Res. , 108 ( 15 ) : 4471
- Kreidenweis , S. and Seinfeld , J. 1988 . Nucleation of Sulfuric Acid–Water and Methanesulfonic Acid–Water Solution Particles: Implications for the Atmospheric Chemistry of Organosulfur Species . Atmos. Environ. , 22 : 283 – 296 .
- Kulmala , M. , Laaksonen , A. and Pirjola , L. 1998 . Parameterizations for Sulfuric Acid/Water Nucleation Rates . J. Geophys. Res. , 103 : 8,301 – 8,307 .
- Leck , C. and Bigg , E. K. 1999 . Aerosol Production Over Remote Marine Areas—A new Route . Geophys. Res. Letters , 26 : 3,577 – 3,581 .
- Leck , C. , Nilsson , E. D. , Bigg , E. K. and Bäcklin , L. 2001 . Atmospheric Program on the Arctic Ocean Expedition 1996 (AOE–96): An Overview of Scientific Goals, Experimental Approach, and Instruments . J. Geophys. Res. , 106 ( 23 ) : 32,051 – 32,067 .
- Leck , C. , Tjernström , M. , Matrai , P. , Swietlicki , E. and Bigg , K. 2004 . Can Marine Micro-Organisms Influence Melting of the Arctic Pack Ice? . EOS , 85 ( 3 ) : 25 – 36 .
- Leck , C. and Bigg , E. K. 2005 . Biogenic Particles in the Surface Microlayer and Overlaying Atmosphere in the Central Arctic Ocean during Summer . Tellus , 57B ( 4 ) : 305 – 316 .
- Leck , C. and Bigg , E. K. 2008 . Comparison of Sources and Nature of the Tropical Aerosol with the Summer High Arctic Aerosol . Tellus , 60B : 118 – 126 .
- Napari , I. , Noppel , M. , Vehkamaki , H. and Kulmala , M. 2002 . An Improved Model for Ternary Nucleation of Sulfuric Acidammoniawater . J. Chem. Phys. , 116 ( 10 ) : 4,221 – 4,227 .
- O'Connor , T. C. , Jennings , S. G. and O'Dowd , C. D. 2008 . Highlights from 50 Years of Aerosol Measurements at Mace Head . Atmos. Res , 90 : 338 – 355 .
- Orellana , M. V. and Verdugo , P. 2003 . Ultraviolet Light Blocks the Organic Carbon Exchange between the Dissolved Phase and the Gel Phase in the Ocean . Limnol. Oceanogr , 48 ( 4 ) : 1,618 – 1,623 .
- Perry , K. D. and Hobbs , P. V. 1994 . Further Evidence for Particle Nucleation in Clear Air Adjacent to a Marine Cumulus Cloud . J. Geophys Res. , 99 : 22,803 – 22,818 .
- Vehkamaki , H. , Kulmala , M. , Napari , I. , Lehtinen , K. E. J. , Timmreck , C. , Noppel , M. and Laaksonen , A. 2002 . An Improved Parameterization for Sulfuric Acidwater Nucleation Rates for Tropospheric and Stratospheric Conditions . J. Geophys. Res. , 107 : D22 4622
- Verdugo , P. , Alldredge , A. L. , Azam , F. , Kirchman , D. L. , Passow , U. and Santschi , P. H. 2004 . The Oceanic Gel Phase: A Bridge in the DOM–POM Continuum . Marine Chem. , 92 : 67 – 85 .
- Wells , M. L. and Goldberg , E. D. 1991 . Occurrence of Small Colloids in Sea Water . Nature , 353 : 342 – 344 .
- Wells , M. L. and Goldberg , E. D. 1993 . Colloid Aggregation in Seawater . Marine Chem. , 41 : 353 – 358 .
- Wells , M. L. and Goldberg , E. D. 1994 . The Distribution of Colloids in the North Atlantic and Southern Oceans . Limnol. Oceanogr , 39 : 286 – 302 .
- Wiedensohler , A. , Covert , D. S. , Swietlicki , E. , Aalto , P. , Heintzenberg , J. and Leck , C. 1996 . Occurrence of an Ultrafine Particle Mode Less than 20 nm in Diameter in the Marine Boundary Layer during Arctic Summer and Autumn . Tellus , 48B : 213 – 222 .