Abstract
The aerosol optical depth (AOD), Angstrom coefficients (α and β), and the second-order Angstrom exponent (α′) obtained by Microtops-II sun photometer have been analyzed in the spectral range 0.34–0.87 μm over the urban polluted city of Delhi, India for the period 2007–2008, aiming at investigating the physical and optical properties of aerosols. The average values of AOD at 500 nm, α and β (in the range 340–870 nm) are found to be 0.78 ± 0.32, 0.78 ± 0.28, and 0.45 ± 0.21, respectively, for the entire period of observations. The AOD data show significant curvature in the lnτ versus lnλ relationship suggesting different dominant aerosol types depending on season. In order to analyze further the curvature effect and the relative dominance of aerosol size, α has been calculated in three wavelength bands, i.e., shorter (0.34–0.50 μm), longer (0.675–0.87 μm), and broad (0.34–0.87 μm) during four seasons, summer (April–June), monsoon (July–September), winter (October–January), and spring (February–March) accompanied with calculations of α′, which quantifies the deviation of logarithmic behavior of AOD with lnλ. The α′ values are found to be positive and higher in the months of October–December and mostly negative in February and March, while close to zero values of α′ are found in April–August. These results indicate that winter season exhibits dominance of fine-mode aerosols while summer relatively higher concentration of coarse-mode particles. On the other hand, monsoon and spring seasons revealed the presence of mixed type, both fine- and coarse-mode aerosols over Delhi.
1. INTRODUCTION
Atmospheric aerosols play a significant role in affecting the earth's climate by altering the solar radiation balance through scattering and absorption of solar radiation and by modifying the cloud properties (Twomey Citation1991; Schwartz et al. Citation1995; Rosenfeld et al. Citation2008). The optical and physical properties of aerosols, such as aerosol optical depth (AOD), single scattering albedo (SSA), and aerosol size distribution are important parameters that control the aerosol radiative forcing at the surface and at the top of the atmosphere. All these properties are highly variable in space and time and several factors contribute to this variability. Among them, we emphasize the sources of aerosols (e.g., volcanic eruptions, sea-salt, biomass burning, fossil fuel burning, biogenic sources, or dust outflows), the mixing states and processes (e.g., coagulation, condensation, hydration, and gas-to-particle conversion) leading to changes in size as well as the reduction in aerosol concentration due to gravitational settling and washout due to precipitation and scavenging. Thus, examining spectral AOD and aerosol size distribution is important for understanding the aerosol types, their source strength, and climate implications (Kaskaoutis et al. Citation2007, Ghan and Schwartz Citation2007).
The Angstrom wavelength exponent (α) is a commonly used parameter to illustrate the wavelength dependence of AOD and to obtain basic information on the aerosol size distribution. For example, Singh et al. (Citation2006) have studied the variation of α and the aerosol size distribution in the central Indian region starting from Delhi to Hyderabad and have shown that in the forest location when α is close to unity, the coarse- and fine-mode aerosol concentrations are nearly equal and it gradually increases as the contribution of fine-mode aerosol concentration increases when one moves toward the more polluted areas. The Angstrom exponent calculated using the spectral variation of AOD has been used in various other previous studies as a tool to estimate particle size distribution and for extrapolating AOD throughout the broad spectral region as well as to distinguish the different aerosol types (Eck et al. Citation1999, 2001; O’Neill et al. Citation2001; Schuster et al. Citation2006; Kalapureddy and Devara Citation2008; Kedia and Ramachandran Citation2009). It is also an indicator of the average aerosol particle size in the atmosphere. Thus, α ≤ 1 indicates aerosol size distribution mainly dominated by coarse-mode aerosols of effective radius usually greater than 0.5 μm. These aerosols mainly originate from dust outflows or sea-spray. On the other hand, α ≥ 1 usually indicates a size distribution dominated by fine-mode aerosols of effective radius smaller than 0.5 μm, usually associated with urban pollution and biomass burning (Eck et al. Citation1999). However, the Angstrom exponent has been found to strongly depend on wavelength. This dependence leads to the spectral curvature of the AOD, which may provide crucial information about the aerosol size distribution (Eck et al. Citation1999, 2001, 2003; Holben et al. 2001; O’Neill et al. Citation2001; Ramachandran Citation2005; Schuster et al. Citation2006; Kaskaoutis et al. Citation2006a, Citation2006b, Citation2007, Citation2009, 2010, Gobbi et al. Citation2007; Kalapureddy and Devara Citation2008, Citation2010; Kambezidis and Kaskaoutis 2008; Komppula et al. Citation2009). As a consequence, it has been used to characterize different aerosol components, such as maritime aerosols (Kaufman et al. Citation2001; Smirnov et al. Citation2002; Vinoj et al. Citation2008), biomass-burning aerosols (Dubovik et al. Citation1998; Reid et al. Citation1999; Eck et al. Citation2001), and rural and urban aerosols (Cachorro et al. Citation2001; Remer and Kaufman 1998).
The main scope of the present work is to examine the seasonal variation of AOD, Angstrom exponent, and curvature of AOD and to associate the results with the presence of fine- and coarse-mode aerosols over the urban polluted location of Delhi, India. Although similar analysis has been performed over other locations in Indian subcontinent, like Hyderabad (Kaskaoutis et al. Citation2009), Ahmedabad (Kedia and Ramachandran 2011), and adjoining oceanic regions of Arabian Sea and Bay of Bengal (Kalapureddy and Devara Citation2008, Citation2010; Kedia and Ramachandran Citation2009; Kalapureddy et al. 2009; Kaskaoutis et al. 2010), to the best of our knowledge it is the first time that such an analysis is being performed over Delhi. This constitutes a real challenge since the urban pollution gets mixed with the desert-dust aerosols during premonsoon and summer seasons over Delhi (Singh et al. Citation2005; Prasad et al. Citation2007), while the winters are highly polluted with large concentrations of black carbon (BC) aerosols from vehicular and other anthropogenic pollution sources leading to the formation of foggy and haze conditions over the area (Ganguly et al. Citation2006, Singh et al. Citation2010).
2. OBSERVATION DETAILS AND METEOROLOGICAL CONDITIONS
The data used in this study were collected at the National Physical Laboratory located in the Delhi downtown (28.38 N, 77.10E, 235masl) area. The AOD data were obtained from January 2007 to December 2008 using Microtops-II (MT) (microprocessor-controlled total ozone portable spectrometer) sun photometer, model no. 4078 manufactured by Solar Light, Inc. This is a hand-held radiometer, which is used to measure aerosol optical thickness at five wavelengths, i.e., 340 nm, 500 nm, 675 nm, 870 nm, and 1020 nm and water vapor at 936 nm with a full width at half maximum (FWHM) of ±2–10 nm. The field of view (FOV) of the MT is 2.5o and typical error in AOD is of the order of ±0.03 in the UV wavelength and ±0.02 in the visible wavelengths. Long-term stability of the filter is better than 0.1 nm year−1. More details about the instrument and the accuracy of measurements for precision and consistency are discussed in detail elsewhere (Morys et al. Citation2001; Srivastava et al. Citation2006; Singh et al. (Citation2010).
A sun target and pointing assembly are permanently attached to the optical block of MT and laser-aligned to ensure accurate alignment with the optical channels. The pointing accuracy of the instrument is better than 0.1o. All the observations were done during clear sky conditions, which imply the cloud-free days or the intervals of the day that were cloud-free. The data were taken nearly every half an hour during the daytime 09:00 to 16:00 h on cloud-free days. Care was also taken so that the FOV remained cloud-free during the observations.
The whole data set has been classified into four seasons on the basis of existing meteorological conditions, namely summer (April–June), monsoon (July–September), winter (October–January), and spring (February to March). Delhi exhibits a semiarid climate with extreme weather conditions. The rainfall pattern usually follows the southwest monsoon, with July–September to be the normal rainy season, while the rainfall in winter is rather infrequent. Thus, due to cloudy conditions and rainfall, the observations are limited during July–September, while the rest of the year is characterized by dry weather with plenty of sunlight.
The meteorological parameters, like wind speed (WS), wind direction (WD), temperature (Temp.), relative humidity (RH%), and accumulated rainfall (RF), were measured by an automatic weather station at the same site. The monthly averaged meteorological features (WS, WD, Temp, RH%) and monthly accumulated rainfall for the years 2007 and 2008 are shown in . The average WS over Delhi is higher during summer season (max. 2.8 m/s) and it reduces during monsoon, while in winter the winds are usually calm. The summer season (April–June) over Delhi is characterized by strong winds, high temperature (30–45°C), and low relative humidity (35–65%). These weather patterns usually carry dust particles from the western desert regions of Thar Desert, Middle East, as well as the Arabian peninsula. The monsoon season shows high RH (75–90%) and low wind speeds (∼1 m/s) with moderately high temperature (average 25–30°C). During this season, the winds are generally south- southeasterly. The winter season is characterized by low temperature (10–20°C), RH between 45 and 75%, and low wind speed (<1.0 m/s), mainly from south-southwesterly directions. The spring season has RH between 60 and 75%, south- westerly winds with speed 0.5–1.0 m/s, and temperature varying between 15 and 25°C. The seasonal variations in meteorological parameters are almost similar during both the years.
3. METHODOLOGY
The AODs measured at four wavelengths (340, 500, 675, and 870 nm) were used to calculate the Angstrom parameters using the Angstrom's empirical expression, given as
The Angstrom wavelength exponent (α) can be calculated using the AODs (τ) at two different wavelengths by applying the Volz method (Equation 1):
It is clear from Equation (2) that α is the negative of the slope (or negative of the first derivative) of τ with respect to wavelength in logarithmic scale. This approach is fully described by Knobelspiesse et al. (Citation2004). Using this method, the errors in α and β arise from the errors in τ which may be very large because of the sensitivity of this method to the selected wavelength interval. The Angstrom parameters can also be derived by applying the least-squares fit to Equation (1) after discarding the strong absorption bands of water vapor and mixed gases. A more detailed analysis of different methods of estimating α and β and the errors associated with them may be found in Kaskaoutis and Kambezidis (Citation2008).
In order to compute the curvature of the ln τ versus ln λ curves, we make use of the second derivative of ln τ versus ln λ, or the derivative of α with respect to the logarithm of wavelength (Eck et al. Citation1999). The second derivative is a measure of the rate of change of the slope with respect to wavelength and, therefore, is a complement to the Angstrom exponent, which is the negative of the slope (first derivative) of ln τ versus ln λ (Eck et al. Citation2001). We can calculate the second derivative of τ as follows:
The α′ values were derived using the observed AODs at 0.34, 0.50, 0.675, and 0.87 μm wavelengths. The aerosol size distribution is rarely unimodal in the atmosphere due to different sources of origin and the mixing processes. The unimodal aerosol size distribution is the Junge size distribution. Once the aerosol size distribution is multimodal, the wavelength dependence of AOD does not follow Equation (1) accurately. Consequently, a deviation from the linear behavior of ln τ versus ln λ is expected and has been reported by several studies (Eck et al. Citation1999; O’Neill et al. Citation2001; Kaskaoutis and Kambezidis Citation2006; Kedia and Ramachandran Citation2009).
The curvature in the AOD spectra could be expressed as a second-order polynomial fit, written as
If α 2 < 0 and α′ > 0, then the curvature will be concave indicating aerosol size distributions dominated by the fine mode and if α2 > 0 and α′ < 0, then the curvature will be convex indicating size distributions with a significant contribution by the coarse mode (Eck et al. Citation1999, 2001; Reid et al. Citation1999; Schuster et al. Citation2006). According to Kaskaoutis et al. (2006b), the polynomial fit to Equation (4) is more precise than the linear fit to logarithms of Equation (1), while for low turbidity conditions large deviations in the above fits usually occur.
4. RESULTS AND DISCUSSION
4.1. Daily and Seasonal Variation of AOD and Angstrom Parameters
AOD is an indicator of the aerosol loading in the vertical column of the atmosphere and constitutes the main parameter to assess the aerosol radiative forcing and its effects on climate. The Angstrom turbidity coefficient (β) is related to the amount of aerosols present in the atmosphere whereas the Angstrom exponent (α) yields information on the predominant size of suspended particles and it is also an indicator of the relative fine-mode strength (Sapkota and Ranjan 2002; Zakey et al. Citation2004). The second-order Angstrom exponent (α′) further quantifies the deviation from linearity of the spectral AOD variation in logarithmic coordinates.
In this section, the average daily as well as the average monthly variations of the AOD at 500 nm, Angstrom parameters α, β, and α′ have been reported. represents the average daily variation of all these parameters for the entire period of observations. During April–May (summer season), the AOD values are high and are also associated with very low α values due to abundance of dust aerosols. In contrast, the high AOD in winter (November–December) is associated with high α values, indicating abundance of fine-mode smoke and anthropogenic particles that dominate during this period due to several open fires for heating and bursting of firecrackers during festival season (Attri et al. 2001). The average value of α calculated in the range 340–870 nm is 0.78 ± 0.28 with turbidity parameter (β) of 0.45 ± 0.21 and average α′ of 0.20 ± 0.57. Such large values of AOD and β indicate a very high aerosol loading over Delhi from both anthropogenic and natural sources that change their dominance seasonally. A value of β > 0.2 usually indicates heavy pollution while β > 0.4 indicates extremely heavy pollution (Iqbal 1983). In the present study, nearly 45% of the daily averaged values of β were found to lie between 0.2 and 0.4 while 55% lied above 0.4, showing abundance of heavy pollution over Delhi. also reveals positive and higher values of α′ in the period October–December in both the years 2007 and 2008, while the values are more negative in March–June in 2007 than those in 2008. According to Eck et al. (Citation2001) and O’Neill et al. (Citation2001), large positive values of α′ are attributed to fine-mode-dominated aerosol size distribution whereas close to zero and negative values of α′ are characteristic of size distributions with a dominant coarse mode.
As the α and α′ calculations have been done for the wavelengths between 340 and 870 nm, the errors in the calculations may be obtained using Equation (4) by estimating the errors in α 1 and α 2 in the same wavelength range. These errors have been estimated and plotted in as a variation with AOD500. There are large errors in the estimated coefficients at lower values of AOD (<0.5), while as the AOD increases (>0.5) the errors decrease significantly. This is because of the better fit of the linear and second-order polynomial to the spectral variability of AOD. A similar pattern in variation of errors has also been found by Kaskaoutis et al. (2010) using MT over the Arabian Sea.
Figure 3 The errors for coefficients a 1 and a 2 estimated in the wavelength range 340–870 nm as a variation with AOD500 values. (Color figure available online.)
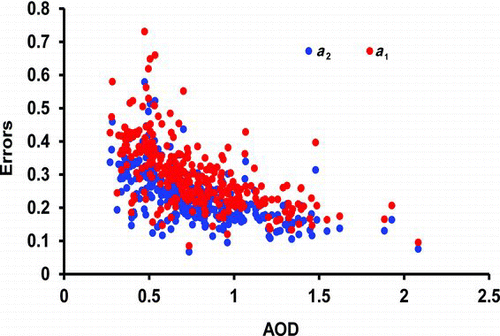
The average monthly variation of the AOD (500 nm), α, and α′ is shown in . The monthly AOD values are found to be maximum during the month of November (AOD ∼ 1.21), which may be due to the festival season of Diwali, when lots of crackers are burst. Except that, the AOD peaks during the months of May and June due to the enhancement in premonsoon dust storms. Usually, the AOD is minimum during the monsoon (August, AOD ∼ 0.46). The average AOD500 during the whole observation period (Jan 2007–Dec 2008) was found to be 0.78 ± 0.32. In a previous study, Singh et al. (Citation2010) found that the average value of AOD500 during 2006 was 0.86 ± 0.42. Another urban location, Kanpur in the Indo-Gangetic Plain also shows high AOD values in the range of 0.6–0.9 (Singh et al. Citation2004; Tripathi et al. Citation2005, 2006). The high AOD values over Delhi are attributed to the very high concentration of local pollution, mainly contributed by the vehicular pollution (∼80%), industry (∼12%), and domestic use (8%) in the city (Singh et al. Citation2010). According to Economic Survey Report of Delhi 2007–2008, the vehicular density is more than 10 times the average vehicular density of the country with more than 5.5 million vehicles, which is further increasing at an average annual rate of 8–10%.
Figure 4 Monthly averaged variation of AOD500, α, and α′ during the period of observation (2007 and 2008).
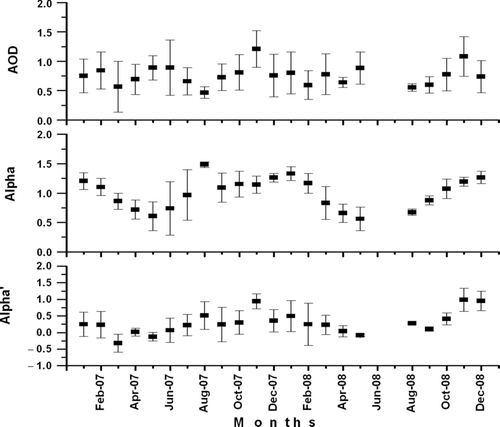
The Angstrom exponent α is found to be minimum during the summer month of May and it gradually increases to the maximum values during winter period (December and January). This indicates a gradual decrease in the coarse particle concentration from summer to winter months. The average monthly α′ values showed a maximum during November (for both years ∼0.99) and minimum during March 2007 (−0.32). α′ values are found to be increasing from October to February indicating quickly changing slope of AOD spectra and domination of fine-mode aerosols in the bimodal aerosol size distribution, while near to zero α′ values are found in the March–June months, which indicates constant slope of AOD spectra and a mixing of fine- and coarse-mode particles (Eck et al. Citation1999).
For analyzing the seasonal variation of AOD (500 nm), each year has been divided into the four dominant seasons described earlier. The average AOD (500 nm) was found to be 0.81 ± 0.32, 0.64 ± 0.22, 0.88 ± 0.36, 0.70 ± 0.40 for summer, monsoon, winter, and spring seasons, respectively, for the year 2007. For the year 2008, the values were 0.70 ± 0.18 (summer), 0.59 ± 0.12 (monsoon), 0.85 ± 0.33 (winter), 0.68 ± 0.30 (spring). The average values for β were 0.53 ± 0.29, 0.30 ± 0.14, 0.39 ± 0.16, 0.36 ± 0.23 for year 2007 and 0.45 ± 0.14, 0.33 ± 0.02 0.38 ± 0.16, 0.33 ± 0.15 for the year 2008 during summer, monsoon, winter, and spring seasons, respectively. The high β values during summer and winter indicate the dominance of severe turbid atmospheres over Delhi. The high AOD during winter is mainly due to local meteorological conditions developed over Delhi when the boundary layer is low and, due to relatively low wind speed, the ventilation coefficient is also very low (Bano et al. Citationin press) resulting in the accumulation of pollutants and aerosol particles. Moreover, the black carbon concentrations in winter become the highest throughout the year due to numerous open fires for heating purposes during the cold period in combination with the increase in fossil fuel combustion (CitationSoni et al. 2010). No significant change in the hourly average α was observed from morning to evening except some marginal increase in the α values during noontime (not shown). On the other hand, considerable seasonal variations were observed in α during the different seasons. The seasonal mean values of α were found to be 0.68 ± 0.29, 1.16 ± 0.34, 1.19 ± 0.16, 0.98 ± 0.18 in year 2007 and 0.64 ± 0.17, 0.84 ± 0.11, 1.21 ± 0.15, 1.02 ± 0.28 in year 2008 for summer, monsoon, winter, and spring seasons, respectively. Higher values of α during winter and spring are related to the biomass/biofuel burning enhancement during these seasons, which produces higher quantities of fine mode and absorbing aerosols in the atmosphere. On the other hand, in both years, the lowest values of α are shown in summer season due to frequency in dust activity as Delhi is in the downwind direction of the dust outflows during this season (Prasad et al. Citation2007; Gautam et al. 2009).
4.2. Angstrom Exponent Variations in Different Wavelength Bands During Different Seasons
In order to characterize the wavelength dependence of α, it has been calculated for three different wavelength bands, shorter (0.34–0.50 μm), longer (0.675–0.87 μm) and broad/total wavelengths (0.34.–0.87 μm). A comparison between α values obtained at shorter and longer wavelengths is shown in regarding the whole set of observations. The straight line with slope unity denotes the line where the two α values (α 0.34–0.50 and α 0.675–0.87) would be equal. It is also the line corresponding to zero curvature (α 2 = 0), as represented by Equations (4) and (5). indicates that over Delhi, 60% of the AOD spectra lie in α 2 > 0 (i.e., concave-type curves, positive curvature) and 40% lie in α 2 < 0 (i.e., convex-type curve, negative curvature) for the years 2007 and 2008. In the case of positive curvature, the rate of change of α is more significant at shorter wavelengths whereas in the case of negative curvature the rate of change of α is more significant at longer wavelengths (as also seen by Kaskaoutis et al. Citation2007). In order to further investigate the seasonal behavior of aerosols, similar plots have been made for the four seasons (). The results show that in summer and monsoon seasons, the values of α 2 are positive in 99% and 97% of the cases, respectively, and the curvature is mostly positive, signifying domination by the coarse-mode aerosols; these aerosols are of dust origin in the majority of the cases (Prasad et al. Citation2007). Further, some negative values of α (675–870) in summer are indicative of dust storm events during summer, since at longer wavelengths the AOD spectral variation is neutral or even slightly increase with wavelength. Similarly, in spring season, about 70% of AOD spectra lie in α 2 > 0 zone (i.e., in about 70% of total cases the curvature is positive) and 30% in α 2 < 0 zone, suggesting the presence of higher concentration of coarse-mode than fine-mode aerosols. However, the winter season shows opposite behavior than the other three seasons. Thus, in winter, the large fraction (about 70% of total) in α 2 < 0 region indicates negative curvature, implying that there is relatively higher concentration of fine-mode aerosols in the size distribution. This may be due to the enhanced concentration of fine-mode vehicular and biomass-burning aerosols during winter due to low ventilation coefficients of the atmosphere. The positive curvature (α 2 > 0) for an atmosphere in which coarse-mode aerosol concentration is high owes to a bimodal distribution with higher coarse-mode contribution, while the opposite exists for negative curvature (Eck et al. Citation1999; Schuster et al. Citation2006; Kaskaoutis et al. Citation2007, 2010).
Figure 6 Variation of differences in α values (α 0.34–0.50–α 0.675–0.87) with AOD500 (a) during January 2007–December 2008 and (b) during different seasons.
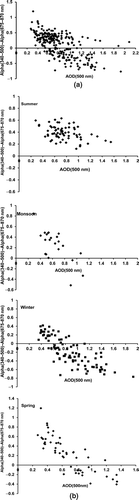
In order to investigate how AOD influences the spectral variation of α through its difference between the values at shorter and longer wavelengths (α 0.34-0.50–α 0.675-0.87), we plotted . The results obtained from (a) do not allow a safe conclusion regarding the differences in α as AOD increases, since the scatter plot is rather complicated with positive and negative dα for both low and high AOD values. Nevertheless, in general, for increasing AOD, the dα becomes mostly negative. Positive and negative differences are indicative of positive and negative curvatures, respectively, whereas differences close to zero show the absence of spectral variability in the Angstrom exponent (Kaskoutis et al. 2007). This rather complicated situation observed for the whole set of observations presents more clear characteristics on seasonal basis (). Thus, during winter, as the AOD500 increases the differences become more negative, i.e., α 0.675–0.87 is greater than α 0.34–0.50. Similar results have been also observed by Kaskaoutis et al. (Citation2007) at Alta Floresta, Brazil, and Ispra, Italy, which indicates enhanced presence of fine-mode particles under turbid atmospheres resulting in negative curvature (Eck et al. Citation1999). Similarly, in spring, the differences (α 0.34–0.50–α 0.675–0.87) become mostly negative at higher AOD500 ≥ 0.8, even though they are less negative than those observed during winter. The three negative dα values for AOD > 0.8 in monsoon cannot be considered as representative of this season due to limited number of observations. On the contrary, during summer as AOD500 increases, the differences tend to approach zero, even though they are positive (α 0.675–0.87 lower than α 0.34–0.50) for all the cases. The always-positive difference in α during summer suggests always-positive curvature of the spectral variability in the Angstrom exponent during this season, which is characteristic of desert-dust aerosols suggesting dominance of the coarse-mode. The scatter plot shows that for coarse-mode particles, the curvature becomes less positive under turbid conditions and the spectral variation of α becomes lesser. Similar variations regarding the coarse-mode aerosols have been reported in previous studies (Eck et al. Citation1999; Kaskaoutis et al. Citation2007) suggesting that bimodal aerosol size distributions with similar contribution of fine- and coarse mode result in negligible curvature. Interestingly, in our study, there are not many points around the zero y-axis indicating that the curvature effect in the spectral AOD variation in logarithmic coordinates is significant over Delhi. Synoptically, the results indicate contrasting seasonal aerosol characteristics with high aerosol loading and predominance of fine-mode aerosols suggesting increased pollution by anthropogenic sources in winter and increased presence of natural coarse mode (e.g., desert dust) aerosols in summer.
4.3. Curvatures in Aerosol Optical Depth Spectra During Different Seasons
Figure 8 Scatter plot of α obtained in different wavelength intervals versus AOD500 (a) during the period 2007–2008 and (b) in different seasons. (Color figure available online.)
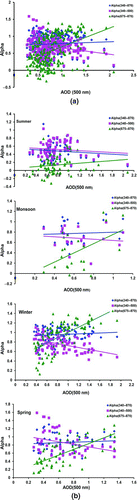
The curvature effects in spectral distribution of AOD are quantified by deriving the second-order Angstrom exponent. shows the variation of α′ as a function of AOD at 500 nm over Delhi for the whole set of measurements. The results show that ∼60% of the AOD spectra have positive α′ consequently signifying a concave curvature and a bimodal aerosol size distribution. Large positive α′ indicates mostly fine-mode particle dominance, which is true for Delhi due to local anthropogenic emissions, while coarse mode dominates mainly during premonsoon season when α becomes close to zero and α′ negative. Nevertheless, for most of the year, the fine mode dominates with moderate to high α values as also reported previously for this location (Singh et al. Citation2010). A general increasing trend in α′ is observed with increasing AOD, while for lower AODs α′ exhibits quite a high variation, ranging from –1.5 up to 1.0. As a result, for lower AODs, both fine- and coarse-mode particles are present in the atmosphere, which are difficult to be discriminated considering the whole period. In contrast, when the AOD is high (>1.0), a single type of aerosol becomes evident either for fine (large positive α′) or coarse (α′ close to zero). Therefore, at a higher AOD value, the curvature is less and α′ approaches zero. shows the scatter plots of the α′ against AOD (500 nm) during different seasons. In winter and monsoon seasons and partly in spring, there are large positive values as AOD500 increases owing to the strong optical influence of fine-mode particles at higher optical depths. In contrast, in summer season, close to zero or mostly negative values of α′ are found, indicating the presence of coarse-mode particles. During spring season, there is a large range of values, both negative and positive. This may be an indication of the existence of bimodal size distributions at comparatively low optical depths (Kaskaoutis et al. Citation2006b, 2007). The large negative values for small AODs point out a remarkable departure from linearity under clean atmospheric conditions. Negative values at the high AODs are related mainly with coarse mode (desert dust) particles (Kaskoutis et al. 2007). Our results are different with the results obtained for Hyderabad by Kaskaoutis et al. (Citation2009), where in winter and post-monsoon the coefficients a 2 showed high positive values for low AOD500 decreasing as AOD increases. shows the correlation between AOD and the Angstrom exponent obtained for three distinct wavelength intervals, shorter (0.34–0.50 μm), longer (0.675–0.87 μm), and total (0.34.–0.87 μm), regarding the whole set of measurements. A decreasing trend or negative correlation can be seen for a shorter wavelength interval (0.34–0.50 μm) throughout the season. In the longer wavelength interval (0.675–0.87 μm), α exhibits an increasing trend or positive correlation with increasing AOD500. Values of α at shorter wavelengths give information regarding the changes in the size of the fine-mode aerosols, while α at longer wavelengths is more sensitive to variations in fine-to-coarse mode ratio (Schuster et al. Citation2006). Schuster et al. (Citation2006) have also found a negative correlation between shorter wavelength α 0.34–0.50 and AOD500, which shows an increase in the fine-mode particle radius with increase in AOD. Furthermore, a positive correlation between α 0.675–0.87 and AOD500 indicates increase in fine-mode fraction under turbid conditions. Similar results have also been observed in other studies by Reid et al. (Citation1999), Eck et al. (2001, 2003), and Kaskaoutis et al. (Citation2006b).
shows the variation of Angstrom exponent derived at three wavelength intervals with respect to AOD500 during the four seasons. In all the seasons, alpha values at longer wavelength interval (675–870 nm) show an increasing trend whereas at a shorter wavelength interval (340–500 nm), these show a decreasing trend with increasing AOD in winter and spring seasons while summer and monsoon seasons show almost constant trend. However, α 0.34–0.87 does not show any correlation with the increasing AOD500, indicating a neutral trend during all the seasons. At the same time, at the shorter wavelengths, the aerosol size distribution tends to shift toward higher particle size radius (decrease in α 0.340–0.500 with AOD).
5. CONCLUSIONS
The monthly and seasonal variation of AOD, the Angstrom exponent, and the curvature of the AOD were investigated over Delhi during the period January 2007–December 2008. The α values were derived from AOD at shorter (0.34–0.50 nm), longer (0.675–0.87 nm), and total/broad (0.34–0.87 nm) wavelength intervals. The α′ values were estimated in the wavelength range 340–870 nm. In the same range, the errors in the coefficients a 1 and a 2 were also estimated. These errors are found to be high at lower AOD500 values and decrease sharply as AOD becomes >0.5. The average AOD500 during the whole observation period (Jan 2007–Dec 2008) was found to be 0.78 ± 0.32. The Angstrom exponent α values varied between 0.39 ± 0.19 and 1.09 ± 0.09 with an average value of 0.78 ± 0.28, while the turbidity parameter (β) was found to be 0.45 ± 0.21. The α′ values showed large fluctuation during the observation period with an average value of 0.20 ± 0.57.
During the summer (April–May) season, AOD values are high and are associated with very low α values due to abundance of dust aerosols. In winter (November–December), AOD is again high but coupled with high α values, indicating abundance of fine-mode particles that dominate during this period. A comparison between α at shorter wavelength (α 0.34–0.50) and longer wavelength (α 0.675–0.87) revealed that the AOD spectra turn more concave leading to a positive curvature, indicating relatively higher concentration of coarse-mode aerosols than fine-mode aerosols, particularly during the summer. Furthermore, in summer and monsoon seasons, the curvature is rarely negative, indicating strong dominance by coarse-mode aerosols, which are of dust storm origin. However, winter season shows mostly negative curvature than all three seasons and relatively higher concentration of fine-mode aerosol in size distribution.
The α′ which was estimated in the wavelength range 340–870 nm shows that it is largely positive during the year indicating mostly fine-mode particle dominance. The positive values are mostly observed in winter due to enhanced black carbon and fine aerosol emissions in this season over Delhi. In contrast, in summer season, close to zero or mostly negative values of α′ were observed indicating the presence of coarse-mode particles. During spring season, there is a large range of values, both negative and positive. This may be an indication for the existence of bimodal size distributions at comparatively low optical depths.
Acknowledgments
The authors are highly grateful to the two referees for providing valuable suggestions and comments toward improving this manuscript.
REFERENCES
- Attri , A. K. , Kumar , U. and Jain , V. K. 2001 . Formation of Ozone by Fireworks . Nature , 411 : 1015
- Bano , T. , Singh , S. , Gupta , N. C. , Soni , K. , Tanwar , R. S. and Nath , S. In press. . Variation of Aerosol Black Carbon Concentration and Its Emission Estimates at the Mega City Delhi . Int. J. Remote Sens. , in press
- Cachorro , V. E. , Vergaz , R. and de Fruto , A. M. 2001 . A Quantitative Comparison of α-Å Turbidity Parameter Retrieved in Different Spectral Ranges Based on Spectroradiometer Solar Radiation Measurements . Atmos. Environ. , 35 ( 30 ) : 5117 – 5124 .
- Dubovik , O. , Holben , B. N. , Kaufman , Y. J. , Yamasoe , M. , Sminov , A. , Tanre , D. and Slutsker , I. 1998 . Single Scattering Albedo of Smoke Retrieved from the Sky Radiance and Solar Transmittance Measured from Ground . J. Geo- Phys. Res. , 103 ( D24 ) 31, 903-31, 923
- Eck , T. F. , Holben , B. N. , Reid , J. S. , Dubovik , O. , Smirnov , A. , O’Neill , N. T. , Slutsker , I. and Kinne , S. 1999 . Wavelength Dependence of the Optical Dept of Bio Mass Burning, Urban, and Desert Dust Aerosols . J. Geophys. Res. , 104 ( D-24 ) : 31333 – 31349 .
- Eck , T. F. , Holben , B. N. , Ward , D. E. , Dubovik , O. , Reid , J. S. , Smirnov , A. , Mukelavi , M. M. , Hsu , N. C. , O’Neill , N. T. and Slutsker , I. 2001 . Characterization of the Optical Properties of Biomass Burning Aerosols in Zambia During the 1997 ZIBBEE Field Campaign . J. Geophys. Res. , 106 : 3425 – 3448 .
- Eck , T. F. , Holben , B. N. , Ward , O. , Reid , J. S. , O’Neill , N. T. , Schafer , D. E. , Dubovik , J. S. , Smirnov , A. , Yamasoe , M. A. and Artaxo , P. 2003 . High Aerosol Optical Depth Biomass Burning Events: A Comparison of Optical Properties for Different Source Regions . Geophys. Res. Lett. , 30 ( 20 ) : 2035
- Ganguly , D. , Jayaraman , A. , Rajesh , T. A. and Gadhavi , H. 2006 . Wintertime Aerosol Properties During Foggy and Nonfoggy Days Over Urban Center Delhi and Their Implications for Shortwave Radiative Forcing . J. Geophys. Res. , 111 : D15217 doi:10.1029/2005JD007029
- Gautam , R. , Liu , Z. , Singh , R. P. and Hsu , N. C. 2009 . Two Contracting Dust-Dominant Periods Over India Observed From MODIS and CALIPSO Data . Geophys. Res. Lett. , 36 : 1–5
- Ghan , S. J. and Schwartz , S. E. 2007 . Aerosol Properties and Processes: A Path from Field and Laboratory Measurements to Global Climate Models . Bull. Am. Meteorol. Soc. , 88 : 1059 – 1083 . doi:10.1175/BAMS-88-7-1059
- Gobbi , G. B. , Kaufman , Y. J. , Koren , I. and Eck , T. F. 2007 . Classification of Aerosol Properties Derived from AERONET Direct Sun Data . Atmos. Chem. Phys. , 7 : 453 – 458 .
- Holben , B. N. 2001 . An Emerging Ground-Based Aerosol Climatology: Aerosol Optical Depth From AERONET . J. Geophys. Res. , 106 : 12067 – 12097 .
- Iqbal , M. 1983 . An Introduction to Solar Radiation , 107 – 128 . New York : Academic Press .
- Kalapureddy , M. C. R. and Devara , P. C. S. 2008 . Characterization of Aerosols Over Oceanic Regions Around India During Pre-Monsoon . Atmos. Environ. , 42 : 6816 – 6827 . doi:10.1016/j.atmosenv.2008.05.022
- Kalapureddy , M. C. R. and Devara , P. C. S. 2010 . Pre-Monsoon Aerosol Optical Properties and Spatial Distribution Over the Arabian Sea During 2006 . Atmos. Res. , 95 : 186 – 196 .
- Kalapureddy , M. C. R. , Kaskaoutis , D. G. , Raj , P. E. , Devera , P. C. S. , Kambezidis , H. D. , Kosmopalos , P. G. and Nostos , P. T. 2009 . Identification of Aerosol Type Over the Arabian Sea in the Pre-Monsoon Season During the Integrated Campaign for Aerosols, Gases, and Radiation Budget (ICARB) . J. Geophys. Res. , 114 : D17203
- Kambezidis , H. D. and Kaskaoutis , D. G. 2008 . Aerosol Climatology Over Four AERONET Sites: An Overview . Atmos. Environ. , 42 ( 8 ) : 1892 – 1906 .
- Kaskaoutis , D. G. , Badarinath , K. V. S. , Kumar Kharol , S. , Rani Sharma , A. and Kambezidis , H. D. 2009 . Variations in the Aerosol Optical Properties and Types Over the Tropical Urban Site of Hyderabad, India . J. Geophys. Res. , 114 : D22204
- Kaskaoutis , D. G. , Kalpureddy , M. C. R. , Moorthy , K. K. , Devera , P. C. S. , Nactos , P. T. and Kosmopoulos , P. G. 2010 . Heterogeneity in Pre-Monsoon Aerosol Types over the Arabian Sea Deduced from Ship-Borne Measurements of Spectral AODs . Atmospheric Chemistry and Physics , 10 : 4893 – 4908 .
- Kaskaoutis , D. G. and Kambezidis , H. D. 2006 . Investigation into the Wavelength Dependence of the Aerosol Optical Depth in the Athens Area . Quart. J. Roy. Meteor. Soc. , 132 : 2217 – 2234 . doi: 10.1256/Qj.05.183
- Kaskaoutis , D. G. and Kambezidis , H. D. 2008 . Comparison of the Ångström Parameters Retrieval in Different Spectral Ranges with the use of Different Techniques . Meteorol. Atmos. Phys. , 99 : 233 – 246 . doi:10.1007/s00703-007-0279-y
- Kaskaoutis , D. G. , Kambezidis , H. D. , Adamopoulos , A. D. and Kassomenos , P. A. 2006a . Comparison Between Experimental Data and Modeling Estimates of Atmospheric Optical Depth Over Athens, Greece . J. Atmos. Sol-Terr. Phys. , 68 : 1167 – 1178 . doi:10.1016/j.jastp.2006.02.011
- Kaskaoutis , D. G. , Kambezidis , H. D. , Adamopoulos , A. D. and Kassomenos , P. A. 2006b . On the Characterization of Aerosols Using the Ångström Exponent in the Athens Area . J. Atmos. Sol-Terr. Phys. , 68 : 2147 – 2163 .
- Kaskaoutis , D. G. , Kambezidis , H. D. , Hatzianastassiou , N. , Kosmopoulos , P. and Badarinath , K. V. S. 2007 . Aerosol Climatology: On the Discrimination of the Aerosol Types Over Four AERONET Sites . Atmos. Chem. Phys. Discuss. , 7 : 6357 – 6411 .
- Kaufman , Y. J. , Smirnov , A. , Holben , B. N. and Dubovik , O. 2001 . Baseline Maritime Aerosol: Methodology to Derive the Optical Thickness and Scattering Properties . Geophys. Res. Lett. , 28 ( 17 ) : 3251 – 3254 . doi:10.1029/2001GL013312
- Kedia , S. and Ramachandran , S. 2009 . Variability in Aerosol Optical and Physical Characteristics Over the Bay of Bengal and the Arabian Sea Deduced from Ångström Exponents . J. Geophys. Res. , 114 : D14207
- Knobelspiesse , K. D. , Pietras , C. , Fargion , G. S. , Wang , M. , Frouin , R. , Miller , M. A. , Subramaniam , A. and Balch , W. M. 2004 . Maritime Aerosol Optical Thickness Measured by Handheld Sun Photometers . Remote Sens. Environ. , 93 : 87 – 106 .
- Komppula , M. , Lihavainen , H. , Hyvärinen , A.-P. , Kerminen , V.-M. , Panwar , T. S. , Sharma , V. P. and Viisanen , Y. 2009 . Physical Properties of Aerosol Particles at a Himalayan Background Site in India . J. Geophys. Res. , 114 : D12202
- Morys , M. , Mims , F. M. III , Hagerup , S. , Anderson , S. E. , Baker , A. , Kia , J. and Walkup , T. 2001 . Design, Calibration, and Performance of MICROTOPS II Handheld Ozone Monitor and Sun Photometer . J. Geophys. Res. , 106 ( 14 ) : 573 – 582 . doi:10.1029/2001JD900103
- O’Neill , N. T. , Eck , T. F. , Holben , B. N. , Smirnov , A. , Dubovik , O. and Royer , A. 2001 . Bimodal Size Distribution Influences on the Variation of Angstrom Derivatives in Spectral and Optical Depth Space . J. Geophys. Res. , 106 : 9787 – 9806 .
- Prasad , A. K. , Singh , S. , Chauhan , S. S. , Srivastava , M. K. , Singh , R. P. and Singh , R. 2007 . Aerosol Radiative Forcing Over the Indo-Gangetic Plains During Major Dust Storms . Atmos. Environ. , 41 : 6289 – 6301 . 2007
- Ramachandran , S. 2005 . PM2.5 Mass Concentrations in Comparison with Aerosol Optical Depths Over the Arabian Sea and Indian Ocean During Winter Monsoon . Atmos. Environ. , 39 ( 10 ) : 1879 – 1890 .
- Reid , J. S. , Eck , T. F. , Christopher , S. A. , Hobbs , P. V. and Holben , B. N. 1999 . Use of the Angstrom Exponent to Estimate the Variability of Optical and Physical Properties of Aging Smoke Particles in Brazil . J. Geophys. Res. , 104 ( 27 ) : 473 – 489 .
- Rosenfeld , D. , Woodley , W. L. , Axisa , D. , Freud , E. , Hudson , J. G. and Givati , A. 2008 . Aircraft Measurements of the Impacts of Pollution Aerosols on Clouds and Precipitation Over the Sierra Nevada . J. Geophys. Res. , 113 : D15203
- Remer , L. A. and Kaufman , Y. J. 1998 . Dynamic Aerosol Model: Urban/Industrial Aerosol . J. Geophys. Res. , 103 ( D12 ) : 13859 – 13871 .
- Sapkota , B. and Rajan , D. 2002 . Atmospheric Turbidity Over Kathmandu Valley . Atmos. Environ. , 36 : 1249 – 1257 .
- Schuster , G. L. , Dubovik , O. and Holben , B. N. 2006 . Angstrom Exponent and Bimodal Aerosol Size Distributions . J. Geophys. Res. , 111 : D07207
- Schwartz , S. E. , Arnold , F. , Blanchet , J.-P. , Durkee , P. , Hofmann , D. J. , Hoppel , W. , King , M. D. , Lacis , A. , Nakajima , T. , Ogren , J. A. , Toon , O. B. and Wendisch , M. 1995 . Group Report: In Aerosol Forcing of Climate , Edited by: Charlson , R. J. and Heintzenberg , J. 251 – 280 . Hoboken, NJ : John Wiley .
- Singh , R. P. , Dey , S. , Tripathi , S. N. , Tare , V. and Holben , B. 2004 . Variability of Aerosol Parameters Over Kanpur, Northern India . J. Geophys. Res. , 109 : D23206
- Singh , S. , Nath , S. , Kohli , R. and Singh , R. 2005 . Aerosols Over Delhi During Pre-Monsoon Months: Characteristics and Effects on Surface Radiation Forcing . Geophys. Res. Lett. , 32 : L13808
- Singh , S. , Singh , B. , Gera , B. S. , Srivastava , M. K. , Dutta , H. N. , Garg , S. C. and Singh , R. 2006 . A Study of Aerosol Optical Depth in the Central Indian Region (17.3o N to 28.6o N) During ISRO-GBP Field Campaign . Atmos. Environ. , 40 : 6494 – 6503 .
- Singh , S. , Soni , K , Bano , T. , Tanwar , R. S. , Nath , S. and Arya , B. C. 2010 . Clear-Sky Direct Aerosol Radiative Forcing Variations Over Mega-City Delhi . Ann. Geophys. , 28 : 1157 – 1166 .
- Smirnov , A. , Holben , B. N. , Kaufman , Y. J. , Dubovik , O. , Eck , T. F. , Slutsker , I. , Pietras , C. and Halthore , R. N. 2002 . Optical Properties of Atmospheric Aerosol in Maritime Environments . J. Atmos. Sci. , 59 : 501 – 523 .
- Soni , K. , Singh , S. , Bano , T. , Tanwar , R. S. and Nath , S. 2010 . Variations in Single Scattering Albedo and Angstrom Absorption Exponent During Different Seasons Over Delhi . Atmos. Environ. , 44 ( 35 ) : 4355 – 4363 .
- Srivastava , M. K. , Singh , S. , Saha , A. , Dumka , U. C. , Hegde , P. , Singh , R. and Pant , P. 2006 . Direct Solar Ultraviolet Irradiance Over Nainital, India in the Central Himalayas for Clear Sky Day Conditions During December 2004 . J. Geophys. Res. , 111 : D08201
- Tripathi , S. N. , Dey , S. , Tare , V. and Satheesh , S. K. 2005 . Aerosol Black Carbon Radiative Forcing at an Industrial City in Northern India . Geophys. Res. Lett. , 32 : L08802
- Tripathi , S. N. 2006 . Measurements of Atmospheric Parameters During Indian Space Research Organization Geosphere Biosphere Programme Land Campaign II at a Typical Location in the Ganga Basin: 1. Physical and Optical Properties . J. Geophys. Res. , 111 : D23209
- Twomey , S. 1991 . Aerosols, Clouds, and Radiation . Atmos. Environ. , 25 ( A ) : 2435 – 2442 .
- Vinoj , V. , Satheesh , S. K. and Moorthy , K. K. 2008 . Aerosol Characteristics at a Remote Island: Minicoy in Southern Arabian Sea . J. Earth Syst. Sci. , 117 : 389 – 398 .
- Zakey , A. S. , Abdelwahab , M. M and Makar , P. A. 2004 . Atmospheric Turbidity Over Egypt . Atmos. Environ. , 38 : 1579 – 1591 .