Abstract
Humic-like substances (HULIS) constitute a class of organic compounds identified in atmospheric samples that influence many properties of aerosols in the atmosphere. In this study, 6 HULIS samples were isolated from atmospheric total suspended particle (TSP) samples collected at 3 locations in Guangzhou of China, 1 each in summer and winter. On the basis of analyses by elemental analyzer, more detailed chemical species of elements were explored by proton nuclear magnetic resonance (1H NMR) spectroscopy for hydrogen and X-ray photoelectron spectroscopy (XPS) for carbon, oxygen, nitrogen, and sulfur. The sources of HULIS were identified by carbon isotopic techniques. The results show that HULIS made up an important component of water-soluble organic carbon (WSOC). Carbon and oxygen were the predominant components in the HULIS. The 1H NMR spectra indicated that H functional groups consisted of aliphatic C‒H, aromatic C‒H, H‒C‒C˭, and H‒C‒O groups. They were all characterized by the highest contents of aliphatic protons and the lowest contents of aromatic protons. The XPS analysis of the HULIS fraction isolated from winter samples at the urban site Wushan (WS) showed that C, O, N, and S have various chemical states. The oxidized carbon groups accounted for 30.5% of total carbon. The nitrogen species contained amide, quaternary, and nitrate groups, and the sulfur species consisted of thiophenes, thioesters, mercaptanes, sulfones, and sulfates. The δ13C values of HULIS showed no obvious differences among the 6 HULIS samples. The δ15N values of summer HULIS samples in Maofengshan Mountain Forest Park (MFS) and Universities Town (UT) were significantly higher than those of winter samples. It is noteworthy that 14C data clearly indicate that even in urban areas biomass-derived carbon was the major component in the atmospheric HULIS samples.
Copyright 2012 American Association for Aerosol Research
INTRODUCTION
During the last 10–20 years, a particularly active research area has been the investigation of the amount and nature of humic-like substances (HULIS) in atmospheric particles. The HULIS are a class of organic compounds identified in atmospheric samples that bears a certain resemblance (acidity, UV-vis absorbance, fluorescence, FTIR, and NMR characteristics) to humic substances (HS) common in soils and aqueous environments (Havers et al. Citation1998; Zappoli et al. Citation1999; Kiss et al. Citation2002; Graber and Rudich Citation2006; Duarte et al. Citation2007). The HULIS are ubiquitous in the atmospheric system. They constitute a significant portion of organic matter (OM) in atmospheric aerosols (up to about 30%) collected in urban and rural environments (Havers et al. Citation1998; Zappoli et al. Citation1999; Decesari et al. Citation2000; Varga et al. Citation2001; Kiss et al. Citation2002; Limbeck et al. Citation2005; Krivácsy et al. Citation2008; Stone et al. Citation2009), at a high alpine site (Krivácsy et al. Citation2001), and in aerosols produced by biomass burning (Mayol-Bracero et al. Citation2002; Lin et al. Citation2009, Citation2010). They have also been found in fog samples (Kiss et al. Citation2001). HULIS can affect many aerosol properties, including hygroscopicity, cloud condensation nucleus (CCN) activity, surface tension, and optical parameters (Kiss et al. Citation2005; Dinar et al. Citation2006, Citation2007; Salma et al. Citation2006; Ziese et al. Citation2008). Because of their water-soluble and surface-active properties, HULIS play an important role in several tropospheric processes. They can influence the radiative balance of the atmosphere and influence the hydrological cycle (IPCC 2001; Ramanathan et al. Citation2005). HULIS also alter the surface tension of nucleating cloud droplets and thereby have important impacts on cloud microphysical properties (Facchini et al. Citation2000; Kiss et al. Citation2005; Dinar et al. Citation2006; Taraniuk et al. Citation2007).
Composition, properties, and interactions of HULIS have been studied by a large variety of experimental methods and techniques, such as different spectroscopic techniques (e.g., UV, IR, NMR, fluorescence; Havers et al. 1998; Decesari et al. 2000; Krivácsy et al. 2001; Kiss et al. 2003; Duarte et al. 2008), size exclusion chromatography methods (SEC; Zappoli et al. 1999; Krivácsy et al. 2000), high performance liquid chromatography–electrospray ionization mass spectrometry (HPLC-ESI-MS; Kiss et al. 2003), LC-MS/MS (Stone et al. 2009), capillary electrophoresis (Havers et al. 1998; Krivácsy et al. 2000), voltammetry (Gelencśer et al. 2000), pyrolysis gas chromatography coupled with mass spectrometry (Gelencśer et al. 2000; Carvalho et al. 2003), and elemental analysis (Kiss et al. 2002). The HULIS are regarded to be an unresolved mixture of water-extracted organic compounds having strong polar and weak (poly)acidic, colloidal, and chromophoric properties (Decesari et al. 2000; Decesari et al. 2001; Krivácsy et al. 2001; Varga et al. 2001; Graham et al. 2002; Kiss et al. 2002; Mayol-Bracero et al. 2002; Graber and Rudich Citation2006). Although there have been many publications about HULIS, the detailed and comprehensive chemical structure, changes of chemical composition with location, and sources of HULIS merit further investigation.
HULIS have been quantified and characterized in bulk aerosols sampled from a number of locations, including polluted/background rural sites (Decesari et al. Citation2001; Kiss et al. Citation2002), continental background areas with and without the influence of biomass burning (Gelencśer et al. Citation2000; Mayol-Bracero et al. Citation2002; Samburova et al. Citation2005; Lukács et al. Citation2007), urban environments (Havers et al. Citation1998; Krivácsy et al. Citation2008; Baduel et al. Citation2010), and marine environments (Cavalli et al. Citation2004). Few measurements of HULIS have been reported in China or other locations in Asia (Yu et al. Citation2004; Miyazaki et al. Citation2007; Lin et al. Citation2009, Citation2010). For example, Lin et al. (Citation2009) studied the abundance and size distributions of HULIS in ambient aerosols in a rural location in South China at a time of visible presence of crop residue burning. The results showed that HULIS were abundant in both ambient samples and in emissions from fresh biomass burning, accounting for 60% of the water-soluble organic carbon (WSOC) in the ambient aerosols and 30% in the aerosols from fresh biomass burning. However, to the best knowledge of the authors, no information has been published about chemical characteristics of the HULIS present in atmospheric aerosol from South China.
Guangzhou is the biggest city in South China, having a population of over 9 million and a total area of over 7000 km2. Because of rapid economic development in the area of the Pearl River Delta (PRD), the air quality in Guangzhou has quickly deteriorated over the past 3 decades, leading to serious concerns. Because of the frequent occurrence of heavy haze in recent years, research to prevent and control atmospheric haze pollution has become a topic of great interest (Wu et al. Citation2006; Tan et al. Citation2009). It is essential to know the chemical composition and features of atmospheric particles in the city to control air pollution in Guangzhou. In previous studies, chemical substances such as metals, water-soluble ions, and organic compounds of aerosol particles in the Guangzhou area have been determined (Cao et al. Citation2004; Wang et al. Citation2006; Duan et al. Citation2007; Bi et al. Citation2008; Ma et al. Citation2010). However, the chemical composition of macromolecular OM has not been investigated. In the present study, we examined the chemistry and possible sources of water-soluble HULIS in 3 regional areas of Guangzhou city, South China. The HULIS samples were isolated from atmospheric aerosols by the solid-phase extraction (SPE) method. The composition of bulk HULIS was analyzed by elemental analyzer, and more detailed information on chemical speciation of elements was obtained by proton nuclear magnetic resonance (1H NMR) for hydrogen and X-ray photoelectron spectroscopy (XPS) for carbon, oxygen, nitrogen, and sulfur. The sources of HULIS were studied by isotopic mass spectrometry. The information provided is useful in understanding the chemistries of HULIS in aerosol particles in relation to the formation of particles in heavily polluted areas.
EXPERIMENTAL
Sample Collection
Six groups of total suspended particle (TSP) samples were collected from Guangzhou city of China in July 2006 (representing summer) and January 2007 (representing winter). Three sampling sites were selected: Maofengshan Mountain Forest Park (MFS), Universities Town (UT), and Wushan (WS), representing biomass and suburban and urban areas of Guangzhou city. Detailed information on sampling sites was provided by Song and Peng (Citation2009).
The TSP samples were collected on Whatman glass fiber filters (GFF; 20.3 cm × 25.4 cm) using a high-volume air sampler at flow rates of 1.05 m3 min−1 (Tianhong Intelligent Instrument Plant of WuHan, China) at the 3 sites. The samples were collected in July 2006 and January 2007, and each filtration lasted for 40 h. A Whatman GFF was used for the aerosol collection and the samples were packed in aluminum foils. The foils and filters were pretreated by baking in a furnace for 4 h at 450°C to volatilize any organic contaminants. Before and after sampling, the foils and filters were weighed at 25°C and 50% RH on a microbalance (Sartorius Model BP210D) with an accuracy of 0.01 mg. The concentrations of TSP were determined by weighing the filters before and after exposure.
Isolation of Water-Soluble HULIS
The HULIS fraction was isolated by a modified one-step version of an SPE protocol based on molecular interactions of non-dissociated species (Varga et al. Citation2001). A total of 4–6 filters were randomly selected from each group of sample filters collected during 4-week sampling periods in July 2006 and January 2007. These samples represent summer and winter seasons, respectively. The collected filters were completely extracted with 500 mL of ultra-pure water by mechanical stirring for 60 min followed by an ultrasonic bath for 30 min. The ratio of filter sample area to water volume was about 4.0 cm2 mL−1. Removal of WSOC from the filters was checked occasionally by an additional sequential extraction and analysis, and it was found to be quantitative. The extracts were filtered through a 0.2-μm Teflon filter (Millipore, Billerica, MA) to remove the filter debris and suspended insoluble particles. The solution pH was measured and was adjusted to 2.0 using hydrochloric acid, and the solution was passed through a pre-conditioned SPE cartridge (Oasis HLB, 500 mg/cartridge, Waters Inc., USA). Hydrophilic organic compounds with acidic functional groups that are protonated at pH = 2 are retained by the column, while the majority of inorganic species, low-molecular-weight organic acids, and sugars were not retained by the SPE cartridge and appeared in the effluent solution (Lin et al. Citation2009). A yellowish band due to the analyte appeared at the top of the column, indicating that the analyte was efficiently retained. The isolation method was optimized to separate the fraction that exhibits the key spectral properties of the total WSOC fraction, i.e., more than 90% of the fluorescence activity and 70% of the UV activity of natural humic and fulvic acids (Varga et al. Citation2001). The exposed column was rinsed with water to remove residues of inorganic constituents, and it was dried in a nitrogen stream. The retained organics were eluted by 5 mL methanol, yielding a markedly colored yellow liquid that contained the HULIS. A disadvantage of the protocol is that some fatty acids, long-chain monocarboxylic acids, and aromatic alcohols could possibly remain in the isolated chemical fraction. The eluates were joined into a pooled sample, which was divided into several aliquots (for different types of measurements), and they were finally evaporated to dryness in a gentle nitrogen stream. The drying resulted in marked dark yellow or brownish matter/pulp. Later, when needed, the HULIS were re-dissolved in ultra-pure water.
The dissolved organic carbon (DOC) content of each aqueous extract was measured with an automated, segmented flow analyzer (TOC-VCPH, Shimadzu) based on a UV-persulfate oxidation method (Lopes et al. Citation2006). The organic carbon (OC) was determined by subtracting carbonate (IC) from total carbon (TC): OC = TC − IC. The data reported are the average results of 3 measurements.
Characterization of Water-Soluble HULIS
Six HULIS samples were characterized for their chemical, structural, and molecular properties by various techniques. The procedures for characterizing the HULIS materials are briefly described below ().
FIG. 1 A flowchart of the experimental procedure for isolation and characterization of atmospheric HULIS.
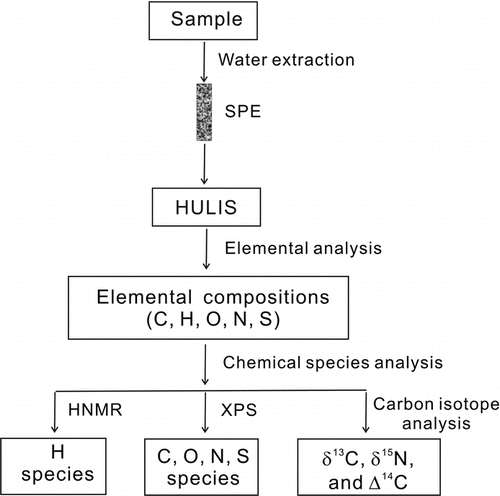
TABLE 1 Mass concentrations of TSP, HULIS, and contribution of HULIS to TSP mass and to the water-soluble OC mass in ambient aerosols
Elemental Composition
The elemental composition (C, H, N, and S) of the HULIS samples was determined with an elemental analyzer (Elementar Vario EL III, Hanau, Germany) following a standard high-temperature combustion procedure. The weight of sample for each determination is ∼1.0 mg. The final data reported are based on the analyses of triplicates for each sample, and the calculated relative standard deviation is less than 1%. Then oxygen contents were determined by calculating the difference: O% = 100 − (C+H+N+S)%.
Proton Nuclear Magnetic Resonance (1H NMR) Spectroscopy
The HULIS samples were analyzed by 1H NMR spectroscopy according to the method reported by Decesari et al. (Citation2000). The weighted samples (∼20 mg) were re-dissolved with D2O containing 0.05% (by weight) sodium 4,4-dimethyl-4-silapentane-1-sulfonate (DSS) as an internal standard. The 1H NMR spectra were obtained at a frequency of 500 MHz on a Varian INOVA 500NB spectrometer. Data were basically acquired 1024 times with a recycle time of 11.6 s for a condensed water sample. A sweep width of 5000 Hz was employed with a digital resolution of 0.15 Hz/point for a 16 K data set.
X-ray Photoelectron Spectroscopy (XPS) Analysis
The surface compositions and the chemical states of selected elements in the sample of WS-winter HULIS were determined by an ESCALAB 250 X-ray photoelectron spectrometer (Thermo VG Scientific, West Sussex, UK) with monochromatic Al Kα X-ray radiation (1486.6 eV) at 150 W (15 kV, 20 mA). The other 5 HULIS samples were not analyzed by XPS because the sample amounts were too small. Survey spectra of the particles (∼100 mg) were collected over the 1100-eV range at a resolution of 1 eV per step, 100 ms per step, and 150-eV pass energy. High-resolution spectra were collected for species of interest at a resolution of 0.1 eV per step, 100 ms per step, and 20.0-eV pass energy.
Because of a slight change in sample charging, the absolute peak positions were sometimes found to have shifted by 2 eV at most. Therefore, the usual procedure for energy calibration was applied to set the energy position of the Cls intensity maximum to the BE of carbon linked to carbon at 284.6 eV. Quantification was performed using a Shirley background and sensitivity factors from Wagner et al. (Citation1981) and Briggs and Seah (1990).
The XPS data were analyzed using XPSPEAK 4.1 software written by Raymund Kwok. The data reported are the average results of 5 measurements on randomly selected sites of each sample.
δ13C and δ15N Isotopic Compositions
The δ13C and δ15N of the HULIS samples were analyzed using a DELTA plus XL mass spectrometer (Thermo Finngan Scientific, USA). The weights of sample for each determination are ∼ 0.5 mg for δ13C and ∼ 3.0 mg for δ15N, respectively. Results are reported in parts per million (‰) calculated as follows:
14C Analysis
The 6 HULIS samples were analyzed by State Key Laboratory of Nuclear Physics and Technology (Peking University) for 14C by accelerator mass spectrometry (AMS). HULIS samples (∼1 mg) were first converted to CO2 and purified cryogenically. The purified CO2 was then reduced to graphite with H2 over Fe and its 14C/12C was measured with an AMS system. The 14C activities were determined with respect to the international standard oxalic acid. Final 14C activities are reported as the fraction of modern carbon (f m), which was determined by comparing the observed 14C content in a sample to that in a standard that has 14C content similar to atmospheric 14CO2 levels:
RESULTS AND DISCUSSION
Abundance of HULIS in TSP
The concentrations of HULIS and its contributions to TSP mass and WSOC fractions in the aerosol samples are listed in . The average ratio of mass to carbon of HULIS is 2.03 ± 0.14, similar to 1.9–2.6 reported in previous studies (Krivácsy et al. Citation2001; Kiss et al. Citation2002; Sullivan et al. Citation2004; Decesari et al. Citation2007; Lin et al. Citation2009). For example, Kiss et al. (Citation2002) measured the elemental composition of the HULIS fraction and obtained an average value of 1.93 for the mass-to-carbon ratio of the HULIS fraction isolated from fine aerosols sampled at rural sites in Hungary. They found that the elemental composition of HULIS was invariant throughout their sampling period. The studies by Krivácsy et al. (2001) and Lin et al. (Citation2009) reported an almost identical value of 1.91 for mass-to-carbon ratio of HULIS in rural alpine aerosols in Switzerland and 1.94 in ambient aerosols in South China. In addition, Turpin et al. (Citation2001) derived OM/OC ratios as concentration-weighted averages from molecular level data sets and recommended ratios of 2.1 for OC in rural areas and as high as 2.2–2.6 for wood smoke.
The ambient HULIS concentrations in our TSP ambient samples ranged from 3.3 to 13.4 μg m−3, which were compared with those available in the literature in locations including South China, Europe, Amazonia, and New Zealand (Zappoli et al. Citation1999; Mayol-Bracero et al. Citation2002; Kiss et al. Citation2002; Samburova et al. Citation2005; Duarte et al. Citation2007; Krivácsy et al. Citation2008; Lin et al. Citation2009, Citation2010; Baduel et al. Citation2010; Salma et al. Citation2010; ). The concentrations of HULIS in MFS are 5.7 and 3.3 μg m−3, in summer and winter, which are different to the concentrations of HULIS in UT and WS. These differences can be explained by the differences in source compositions and atmospheric photochemical reactions.
TABLE 2 Summary of abundance of HULIS in ambient aerosols reported in the literatures
HULIS is an important component of WSOC. It accounted for a large fraction of WSOC in our samples, 36.0–44.0% of the WSOC on a carbon basis. The percentages lie in the range of 15–72% in fine aerosols found in many earlier studies (; Zappoli et al. 1999; Mayol-Bracero et al. 2002; Kiss et al. 2002; Samburova et al. 2005; Krivácsy et al. 2008; Lin et al. 2009, 2010; Baduel et al. 2010; Salma et al. 2010). No significant differences among the 6 samples in our study were observed. The high HULIS levels in our study and in the other studies cited indicate that high-molecular-weight HULIS is an important component of WSOC.
WSOC accounted for 16.8–41.7% of OC in the ambient aerosols (). The range is similar to the 30–70% of the total carbon that can be water soluble, as reported in previous studies (Sempéré and Kawamura Citation1994; Zappoli et al. Citation1999; Yu et al. Citation2004). The measurements in the present study also confirmed the earlier measurements in the PRD region showing that water-soluble organic matter (WSOM) constitutes a substantial fraction of particulate OM (Yu et al. Citation2004; Huang et al. Citation2006; Lin et al. Citation2009).
Elemental Compositions
The elemental analysis data of HULIS samples from 6 pooled-TSPs are shown in , which indicates that C, H, O, N, and S were always present in the 6 HULIS samples. The average elemental composition was found to be 49.4% (C), 5.7% (H), 40.1% (O), 3.1% (N), and 1.6% (S). Except for sulfur content, the results were very similar to those obtained earlier by Krivácsy et al. (2001) for HULIS (52.3% (C), 6.7% (H), 38.5% (O), and 2.5% (N)) from rural alpine aerosol particles and by Kiss et al. (Citation2002) for HULIS (52% (C), 6.2% (H), 39% (O), and 2.5% (N)) in fine aerosol particles collected in a rural area in Hungary. These values are within the range of those published for Suwannee River humic acid (SRHA), Suwannee River fulvic acid (SRFA), and WSOC hydrophobic acid fractions or HULIS from atmospheric aerosols (Krivácsy et al. Citation2001; Kiss et al. Citation2002; Duarte et al. Citation2007). These data clearly show that carbon and oxygen are the dominant elements in the 6 HULIS samples. The relative weight percentages account for 88.0–91.7% of the HULIS samples. Although organic sulfur compounds in atmospheric aerosol have been analyzed in previous studies (Romero and Oehme Citation2005; Linuma et al. Citation2007; Surratt et al. Citation2007; Altieri et al. Citation2009; Nozière et al. Citation2010), the content of sulfur in atmospheric HULIS samples has not been determined previously. The content of sulfur measured in the current study is 1.24–2.13%. These sulfur contents are significantly higher than those of standard fulvic acids. For example, the contents of sulfur in SRFA and Nordic Lake fulvic acid (NLFA) are 0.44% and 0.46%, respectively (http://www.ihss.gatech.edu). These results suggest that organic-S-containing groups are an important component of atmospheric HULIS. Little information on seasonal or spatial differences can be derived from only the elemental composition data, but through the examination of the atomic ratios (H/C, O/C, N/C, and S/C), some qualitative estimation can be made.
FIG. 2 Changes of relative content of HULIS/TSP, Ar−H as a function of O/C or H/C atomic ratio for 6 atmospheric HULIS samples. Symbols represent data points and lines are the best linear fit. “▪”: MFS-summer; “□”: MFS-winter; “◊” : UT-summer; “⋄”: UT-winter; “▴”: WS-summer; “▵”: WS-winter.
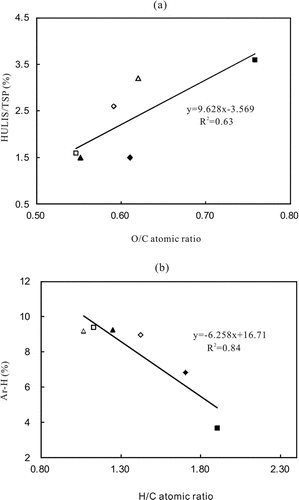
TABLE 3 Elemental composition (%), atomic ratios, δ13C, δ15N, and 14C of the 6 HULIS fractions
These atomic ratios are often used to describe characteristic and structural changes of organic macromolecules (Lu et al. Citation2000; Song et al. Citation2002, Citation2005). The results show that O/C atomic ratios for HULIS are similar to those for standard fulvic acids (Duarte et al. Citation2007), while the H/C atomic ratios for HULIS are substantially higher (Duarte et al. Citation2007). For example, the molar ratios of O/C and H/C for SRFA and NLFA are 0.60, 0.99 and 0.65, 0.91, respectively. Thus, high H/C atomic ratios in the 6 HULIS samples indicate the presence of large amounts of aliphatic compounds and, in addition, a predominance of oxygenated functional groups in the chemical structures. The O/C atomic ratio of HULIS samples appears to be positively correlated with the relative abundance of HULIS in these samples. shows the linear correlations between HULIS/TSP and O/C atomic ratios. These relationships further support the conclusion that oxygenated functional groups are an important component in atmospheric HULIS. The N/C atomic ratios for the HULIS samples are 0.033–0.067, in general, higher than those found for humic and fulvic acids from Suwannee River and for humic acid from River Vouga (Duarte et al. Citation2007). Such differences are likely to be a consequence of the distinct origins of both aerosol OM and aquatic HS (Graber and Rudich Citation2006). The S/C atomic ratios for the HULIS samples are 0.009–0.018. These are actually higher than those for fulvic acid from SRFA and NLFA (http://www.ihss.gatech.edu), suggesting that more organic sulfur groups were contained in the atmospheric HULIS samples.
FIG. 3 1H NMR spectrum for HULIS fraction obtained from WS-winter sample. Four specific spectral regions are identified at the top of the spectra. The region between 4‰ and 5‰ is not shown because of instrumental noise due to a residual signal of HDO.
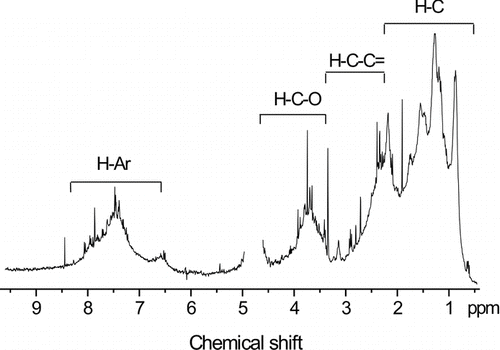
These atomic ratios manifest some seasonal and spatial changes. For example, H/C atomic ratios of the HULIS samples in summer were always higher than those in winter at the same sampling sites. The O/C atomic ratios of summer samples are different among the 3 sites (), showing the trend MFS > UT ≈ WS, contrary to the changing trend of winter samples. The seasonal changes of O/C differ among the 3 sites. The O/C atomic ratio of summer samples is actually higher than that of winter samples at the MFS site. However, no significant seasonal differences were observed for the O/C atomic ratio at the UT and WS sites. These changes can be explained by differences of primary and secondary sources among the 3 sites. For example, MFS is a forest park where more biogenic volatile organic compounds (bio-VOCs) were emitted, which took part in the atmospheric photochemical reactions in summer than in winter. The photochemical reactions resulted in a large amount of oxygen-containing compounds in the aerosol HULIS sample in the MFS summer sample (Bonn and Lawrence Citation2005; Krolla and Seinfeld Citation2008).
The N/C atomic ratios exhibit some seasonal variation, with the higher values being observed for HULIS samples of particles collected at MFS during summer and at UT and WS during winter (). The higher content of organic nitrogen groups in MFS summer HULIS can be attributed to the enhanced emissions of N-containing compounds in forest regions in summer (Li et al. Citation2007) and the presence of strong microbial activity caused by high temperatures and humidity in summer (Matthias-Maser and Jaenicke Citation2000). In comparison, the higher content of organic nitrogen compounds in winter samples from UT and WS may be associated with the enhancement of photochemical reactions of individual vapor-phase organic compounds with reactive inorganic forms of nitrogen during these periods of high solar intensity and great amounts of biomass-burning emissions in winter (Lin et al. Citation2009, Citation2010; Ma et al. Citation2010).
The S/C atomic ratio of HULIS from MFS in summer is 0.018, significantly more than the 0.09 from MFS in winter. This difference can be due to a great amount of S-containing compounds emitting from soil and taking part in the formation of aerosol particles in the hot summer at forest of MFS (Yi et al. Citation2010).
H Functional Group Analysis by 1H NMR
The 1H NMR is an effective technique for analyzing the H functional structure of macromolecular compounds (Decesari et al. Citation2000; Graham et al. Citation2002). The 1H NMR spectra of 6 HULIS samples collected at WS, UT, and MFS in summer and winter were obtained and the WS winter spectrum as an example is shown in . The most distinct feature of HULIS spectra is that sharp signals account for a minor portion of the total integrated area of the spectra. However, most of the signals remain unresolved, appearing as a continuous distribution and suggesting the occurrence of complex mixtures of structures. According to the chemical shifts, resonance peaks in the 1H NMR spectra can be divided into 4 representative categories of functional groups: (1) Ar-H: aromatic protons (6.5–8.3‰); (2) H‒C‒O: protons on carbon atoms singly bound to oxygen atoms (3.3–4.5‰), showing that carbohydrates and ethers are present in the HULIS; (3) H‒C‒C˭: aliphatic protons on carbon atoms adjacent to carbonyl groups or aromatic rings (2.0–3.2‰); and (4) H‒C: aliphatic protons (0.7–2.0‰). Among these functional groups, the wide range of chemical shifts of signals attributed to aromatic protons suggests the occurrence of highly substituted aromatic rings that cover shifts of phenols and alkylbenzenes (around 6.5–7.0‰), benzoic acids or esters, and, perhaps, nitroaromatics (>7.4‰). Other functional groups that could have been detected in regions of the spectra but for which low intensity (or no signal) was actually observed include aliphatic amines and sulfonates (2.9–3.2‰).
The abundances of the four main categories of structural units listed above can be estimated from the areas of the 1H NMR bands (). The 6 HULIS samples are all characterized by the highest contents of aliphatic protons (H‒C; 56.1 ± 2.1%), moderate contents of the H‒C‒C˭ group (23.5 ± 2.7%), and relatively low contents of the H‒C‒O group (12.5 ± 2.0%) and aromatic protons (H‒C; 7.9 ± 2.3%). These values of H functional groups are within the range of those published for WSOC hydrophobic acid fractions or HULIS from atmospheric aerosols (Decesari et al. Citation2000, Citation2001, 2005; Graham et al. Citation2002). These 1H NMR data are also similar to those reported for fulvic acids of Engaňo Bay, Chubut River, and Big Soda Lake (Spitzy and Leenheer Citation1988), which are characterized by high aliphatic carbon contents and low aromatic carbon contents. However, the proton compositions of HULIS are obviously different from those of fulvic acids isolated from sediments and waters. For example, SRFA contains high H‒C‒O and H‒C‒C˭ contents (Leenheer et al. Citation1998); the differences of origins and forming mechanisms for HULIS and fulvic acids may be the reason.
These 1H NMR data suggest that the 6 HULIS samples are similar in chemical structures of H species. However, some differences can be observed among the samples. The 1H NMR data of shows that the relative content of H‒C‒O group in MFS summer sample is 15.8%, significantly higher than the 10.1% of the MSF winter sample, whereas the H‒C‒O groups in HULIS samples at UT and WS do not present obvious seasonal differences. Such difference could be due to the predominant biomass sources at MFS emitting more bio-VOCs and oxygen-containing compounds such as sugars and polysaccharides in summer than in winter. These compounds can be oxidized in the atmosphere and result in the formation of H‒C‒O groups in the aerosol HULIS samples (Krolla and Seinfeld Citation2008). The aromatic moieties generally are correlated with emissions from anthropogenic activities. The relative content of aromatic protons in summer HULIS samples in MFS is significantly lower than those in UT and WS; this could be due to enhanced anthropogenic activities in UT and WS.
TABLE 4 The proton species in the 6 HULIS fractions
These functional groups in the HULIS samples appear to be correlated with the elemental composition. shows that the content of Ar-H linearly decreased as a function of H/C atomic ratio, with correlation coefficient R 2 = 0.84. Such linear correlations suggest that the Ar-H groups should be associated with precursors of hydrogen deficiency.
Close observation of the spectral bands indicates that the aliphatic moieties among the 6 HULIS samples are a little different. According to the chemical shifts, saturated and unsaturated aliphatic moieties can be recognized. The former refers to aliphatic protons (C‒H) and protons on carbon atoms singly bonded to oxygen atoms (H‒C‒O). The latter denotes aliphatic moieties that include methyls/methylenes/methynes bound to either C˭C (aromatic or vinylic substituents) or C˭O groups (carbonyls or carboxyls). The ratio (α) between saturated and unsaturated aliphatic C‒H groups varies from 0.28 to 0.39 among these samples. The reasons for these variations are not known, but the differences in the primary sources and secondary atmospheric formation processes may play an important role.
The saturated aliphatic protons (C‒H) are particularly abundant in the following regions: 0.7–1.0‰ (methyl groups, −CH3) and 1.0–2.0‰ (chain methylene groups, −CH2−). shows that the −CH3/−CH2− ratios lie in a narrow range (0.22–0.28), suggesting that the 6 HULIS samples have similar saturated aliphatic proton structures. The lower content of methylene groups indicates that HULIS contains a low content of long-chain alkanes.
C, O, N, and S Functional Groups Analyzed by XPS
The XPS spectrum is a powerful technique for determining the surface chemical composition and structure of solid materials (Kelemen et al. Citation1994, Citation2002; Haselbach and Ma Citation2008). Among its many advantages are that it is non-destructive and that it is sensitive to chemical shift. Because of these advantages, XPS has been used to investigate natural macromolecular OM such as humic acids and coals (Monteil-Rivera et al. Citation2000; Olivella et al. Citation2002a,b). Although this technique is a surface-restricted technique, XPS has great potential to resolve and to quantify some of the functional groups of bulk HS (Monteil-Rivera et al. Citation2000). Therefore, the XPS technique was employed to investigate the elemental composition and chemical states of the HULIS samples isolated from atmospheric aerosols.
In the present study, only the HULIS fraction collected in winter from WS was analyzed by XPS. The other samples were too small for XPS analysis. The survey spectra for the WS-winter HULIS sample reveal the presence of 4 major elements: carbon, oxygen, nitrogen, and sulfur (). Atomic compositions for each sample were calculated using narrow-scan peak areas and the appropriate sensitivity factors for each element (Chastain Citation1992; Atzei and Rossi Citation2004). Carbon and oxygen clearly dominated the chemical composition. Their relative weight percentages were about 94.4% for the HULIS samples, similar to the range of 94.6–98.8% for humic acid (Monteil-Rivera et al. Citation2000). The contents of nitrogen and sulfur were 4.0% and 1.6%, respectively.
In , the weight percentages measured by XPS are compared to the values deduced from elemental analysis. Both elemental and XPS analyses led to comparable amounts of nitrogen and sulfur. shows that noticeable differences occurred for oxygen in HULIS winter samples. The weight percentage of oxygen measured by XPS was 31.6%, significantly lower than the calculated value (42.9%) from the values (C, H, O, N) obtained with an elemental analyzer. This discrepancy in oxygen between surface and bulk contents can be due to the fact that the bulk oxygen content was calculated without considering the ash, which may lead to overestimation of bulk oxygen content in HULIS. These differences may also signify a preferential arrangement of the oxygen-containing groups inside the solid during the precipitation process. Although not fully satisfying for this HULIS, the observed agreement between elemental and XPS studies is reasonable enough for one to further investigate the XPS results (Monteil-Rivera et al. Citation2000).
Carbon and Oxygen Functional Groups
The high resolution XPS spectra show that more than 1 chemical embedment was identified in HULIS for the elements carbon, oxygen, nitrogen, and sulfur. Background-subtracted, high-resolution C1s spectra are shown in , which shows 2 consecutive peaks. To quantify the high energy tail of the Cls signal in more detail, carbon contents were classified into 7 types: (1) unsubstituted aromatic carbon (C‒C/C‒H), (2) aliphatic carbon (C‒C/C‒H), (3) α-carbon (C‒C(O)O), (4) ether or alcohol carbon (C‒O), (5) ketonic carbon (C=O and O‒C‒O), (6) amide carbon (C(O)N), and (7) carboxylic carbon (C(O)O) according to the C1s spectra (Monteil-Rivera et al. Citation2000; Atzei and Rossi Citation2004; Song and Peng Citation2009). Then a standard line shape analysis with a Lorentzian-Gaussian fitting function was used. The chemical assignments and experimental area percentages for each component of the C1s spectra of the HULIS sample are shown in .
TABLE 5 The percentage area (%) and standard deviation for chemical peaks under the C1s, N1s, and S2p envelopes for WS-winter HULIS
FIG. 4 XPS spectra for HULIS fraction obtained from WS-winter sample. (a) XPS survey spectra; (b) C1s spectra; (c) N1s spectra; (d) S2p spectra. Peak numbers of C1s spectra correspond to the carbons mentioned in the text: (1) unsubstituted aromatic carbon (C‒C/C‒H), (2) aliphatic carbon (C‒C/C‒H), (3) α-carbon (C‒C(O)O), (4) ether or alcohol carbon (C‒O), (5) ketonic carbon (C=O) and O‒C‒O, (6) amide carbon (C(O)N), and (7) carboxylic carbon (C(O)O). Peak 1, 2, 3 of N1s spectra correspond to amide, quaternary type nitrogen functionalities, and nitrate groups, respectively. Peak 1, 2, 3 of S2p spectra correspond to thiophenes, thioeters, and mercaptanes; sulphone; and sulphonate/sulphate groups, respectively.
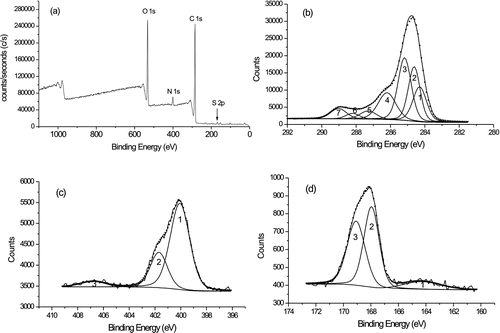
The results show that the C‒H functional groups are present, including aromatic C‒H and aliphatic C‒H. The ratio of C‒Haromatic/C‒Haliphatic was 0.88, indicating that aromatic and aliphatic C‒H groups are both important components of atmospheric HULIS. The ratio is lower than the range of 0.99–1.53 reported for humic acids (Monteil-Rivera et al. Citation2000), indicating that atmospheric HULIS contains a relatively low content of aromatic groups and a relatively high content of aliphatic groups compared with humic acids. Several different forms of oxidized carbon were also identified in the C1s spectra. Because the O1s peak is very smooth and symmetrical, the analysis of the amount and type of oxygen-containing species was performed only on the basis of the maximum C1s. The results show that the oxygen-containing groups including C‒O, C˭O (or O‒C‒O), C(O)N, and COO‒ were identified. The relative amount of these oxygen-containing surface groups is 30.5%, within the range of 28.3–38.1% for humic acids (Monteil-Rivera et al. Citation2000). Among these, the C‒O group has the highest content (15.3%), which suggests that carbohydrates and ethers may be the most important components in the HULIS.
Nitrogen Functional Groups
shows the high-resolution N1s spectra of the WS-winter HULIS sample. This is a well-defined, asymmetrical N1s peak, which was fitted to 3 components centered at (1) 400.1, (2) 401.5, and (3) 407.0 eV. The area percentages were then calculated and are shown in . A comparison of N1s peak areas showed that the peak at 400.1 eV had the highest intensity, which is a result of contributions from pyrrolic and amide nitrogen forms. By combining the percentage with the atomic concentration of nitrogen in HULIS (3.84%), one can evaluate the atomic concentration of −C(O)N and hence its proportion of total carbon. A concentration of 3.38% of total carbon was calculated, thus validating the concentration (3.44%) estimated from the C1s spectrum. These results suggest that the nitrogen in the 400.1 eV region is mainly assignable to amides. The other peaks, at 401.5 and 407.0 eV, indicate the presence of quaternary and nitrate nitrogen, respectively (Kelemen et al. Citation1994, Citation2002).
Sulfur Functional Groups
Organic sulfur compounds in atmospheric aerosol have been investigated previously (Romero and Oehme Citation2005; Linuma et al. Citation2007; Surratt et al. Citation2007; Altieri et al. Citation2009; Nozière et al. Citation2010). However, these studies only measured the small organic sulfur compounds by mass spectrometry. The chemical states of total sulfur compounds have not been studied.
In the present study, the high-resolution X-ray photoelectron spectra in the S2p region confirmed that the total sulfur species present in HULIS consisted essentially of thiophenes, thioesters, and mercaptanes, sulfones, and sulfates (; Olivella et al. 2002a,b; Pietrzak and Wachowska 2006). Among these sulfur species, the sulfur groups of thiophenes, thioesters, and mercaptanes may be formed from the S-containing compounds directly emitted from wetlands such as rice paddy fields, the litter layer of forest soils, the aerobic decomposition of food wastes, oil-fired boiler, and so on (Yi et al. Citation2008, Citation2010; Wu et al. Citation2010; Vander Wal et al. Citation2011). The occurrence of sulfone sulfur suggested the possibility of SOx incorporation into OM via photochemical processes. Sulfates are secondary particles mainly formed from heterogeneous and homogeneous photochemical reactions during transport (Seinfeld Citation1986; Raes et al. Citation2000). The relative amounts of sulfur groups were estimated and expressed as the percentage of the S2p peak at appropriate binding energies and are shown in . The content of sulfones in total sulfur species of HULIS was 45.9%, indicating that oxygenated sulfur in organic form is an important component in the sulfur of HULIS. Thus the percentage content of sulfones and sulfates was 89%, suggesting that highly oxygenated sulfur is the predominant component in the total sulfur of HULIS.
δ13C and δ15N of HULIS
Stable carbon and nitrogen isotopes are important markers of OM origin in atmospheric, terrestrial, and aquatic environments. Carbon isotopes are widely used in studies of ecology and the origin and migration of organic materials. Nitrogen isotopes are widely applied in research on the biological nitrogen cycle.
Six HULIS samples were analyzed for δ13C of CHULIS, with the results shown in . The average δ13C of the HULIS samples was −25.7 ± 0.3‰, falling in a narrow range of −25.2‰ to −26.1‰. These values fall within the range of the isotopic composition of C3 plants (Ballentine et al. Citation1998) as well as biomass burning and industrial combustion (Kelly et al. Citation2005). Therefore biogenic emissions and/or industrial emissions are likely to be the main sources of OC in the HULIS samples. No clear differences in δ13C were observed among the HULIS samples, perhaps because the stable carbon isotopic ranges of different source contributions to HULIS are similar. These considerations suggest that the δ13C value may not be a good index to indicate the source of atmospheric HULIS.
Nitrogen isotopic composition is also helpful in the identification of the source of OM. The nitrogen isotopic composition of HULIS samples spans a relatively wide range, from 1.24‰ to 5.60 ‰. This range is narrower than the −14.6‰ to +12.5‰ range found in a nitrogen isotope study of atmospheric OM by Kelly et al. (Citation2005). It is clear that the isotopic composition of the HULIS samples in general falls in the range of terrestrial plants and soil and overlaps with part of the range of atmospheric inorganic nitrogen components (Russell et al. Citation1998; Yeatman et al. Citation2001). The δ15N values manifest some seasonal differences. The δ15N values of summer samples are 5.49‰ and 5.60‰ at MFS and UT, respectively, significantly higher than that of winter samples. These seasonal differences can be explained by the fact that a great deal of nitrogen with more positive δ15N was emitted from terrestrial plant debris at high temperature and humidity in summer and took part in the formation of atmospheric HULIS at MFS and UT (Kelly et al. Citation2005). These resulted in the summer samples at MFS and UT having more positive δ15N values. However, the δ15N value of the WS winter sample is slightly lower than that of the summer samples, possibly because of differences in the formation mechanism of N-groups between summer and winter (Freyer et al. Citation1993).
Δ14C of HULIS
The radiocarbon data in show that the biomass fraction of atmospheric HULIS averaged 0.58 (0.51–0.65). The Δ14C data suggest that even in an urban area such as Guangzhou, biomass-derived carbon is the major component in atmospheric HULIS. This is similar to the 51–80% range of OC in urban aerosols of Zürich (Switzerland; Szidat et al. 2004). Some differences were observed among the samples. In the summer samples, there was a higher biomass fraction in MFS samples than in WS and UT samples. These differences can be explained by a combination of 2 factors: high primary biomass emissions and high secondary organic aerosol (SOA) concentrations formed from biomass-derived precursors. Compared with the summer sample, there was a higher biomass fraction in winter samples at MFS and WS than at UT. It is noteworthy that the biomass fraction of winter HULIS at WS is 0.63, significantly higher than the 0.51 of winter HULIS at UT. The reasons for this variation in fractions of biogenic carbon from season to season are not yet clear.
CONCLUSIONS
This study showed that HULIS isolated from the ambient TSP samples in Guangzhou city of South China is an important component of WSOC, accounting for 36.0–44.0% of the WSOC on a carbon basis. The mass concentration and contribution of HULIS to TSP and WSOC showed some spatial and seasonal differences. Six HULIS samples have similar chemical compositions. C, H, O, N, and S always existed in the HULIS samples, but the atomic ratios of O/C and N/C were different at different sites and in different seasons. The 1H NMR spectra indicated that H functional groups in HULIS samples are all characterized by the highest contents of aliphatic protons and the lowest contents of aromatic protons. They also showed that H‒C‒C˭ and H‒C‒O groups were distributed between the aliphatic and aromatic protons. The aliphatic H‒C group was always much richer than benzyls. The XPS analysis of the WS-winter sample shows that C, O, N, and S compounds predominated in the HULIS. Each of them has various chemical states. The oxidized carbon groups accounted for 30.5% of total carbon; the nitrogen species contained amide, quaternary, and nitrate groups; the sulfur species consisted of thiophenes, thioesters, mercaptanes, sulfones, and sulfates. These differences in chemical composition and species could be due to differences in the contributions from primary sources and secondary atmospheric reactions.
The δ13C values show no obvious differences among the HULIS samples, suggesting that δ13C is not a good indicator of the source of atmospheric HULIS. The δ15N values of summer samples at MFS and UT are significantly higher than those of winter samples, possibly because compounds with more positive δ15N values were emitted at the high temperature and humidity of summer at MFS and UT. The radiocarbon data clearly indicate that even in an urban area, biomass-derived carbon is the major component in atmospheric HULIS.
Acknowledgments
The work was supported by the Natural Science Foundation of China (No. 40975090, 40830745, and 40505026) and the Guangdong Natural Science Foundation (9151064004000008). This is contribution No. IS-1414 from GIGCAS. We also thank 2 anonymous reviewers for their valuable comments and suggestions on the original manuscript.
REFERENCES
- Altieri , K. E. , Turpin , B. J. and Seitzinger , S. P. 2009 . Oligomers, Organosulfates, and Nitrooxyorganosulfates in Rainwater Identified by Ultra-High Resolution Electrospray Ionization FT-ICR Mass Spectrometry . Atmos. Chem. Phys. , 9 : 2533 – 2542 .
- Atzei , D. and Rossi , A. 2004 . Quantitative Surface Analysis of Urban Airborne Particles by X-ray Photoelectron Spectroscopy . Ann. Chim. , 94 : 123 – 133 .
- Baduel , C. , Voisin , D. and Jaffrezo , J.-L. 2010 . Seasonal Variations of Concentrations and Optical Properties of Water Soluble HULIS Collected in Urban Environments . Atmos. Chem. Phys. , 10 : 4085 – 4095 .
- Ballentine , D. C. , Macko , S. A. and Turekian , V. C. 1998 . Variability of Stable Carbon from Combustion of C4 and C3 Plants: Implications for Biomass Burning . Chem. Geol. , 152 : 151 – 161 .
- Bi , X. , Simoneit , B. R. T. , Sheng , G. , Ma , S. and Fu , J. 2008 . Composition and Major Sources of Organic Compounds in Urban Aerosols . Atmos. Res. , 88 : 256 – 265 .
- Bonn , B. and Lawrence , M. G. 2005 . Influence of Biogenic Secondary Organic Aerosol Formation Approaches on Atmospheric Chemistry . J. Atmos. Chem. , 51 : 235 – 270 .
- Briggs , D. and Seah , M. P. 1990 . Practical Surface Analysis: Auger and X-ray Photoelectron Spectroscopy (Vol. 1) , New York. : Wiley .
- Cao , J. J. , Lee , S. C. , Ho , K. F. , Zou , S. C. , Fung , K. Li , Y. , . 2004 . Spatial and Seasonal Variations of Atmospheric Organic Carbon and Elemental Carbon in Pearl River Delta Region, China . Atmos. Environ. , 38 : 4447 – 4456 .
- Carvalho , A. , Pio , C. and Santos , C. 2003 . Water-Soluble Hydroxylated Organic Compounds in German and Finnish Aerosols . Atmos. Environ. , 37 : 1775 – 1783 .
- Cavalli , F. , Facchini , M. C. , Decesari , S. , Mircea , M. , Emblico , L. Fuzzi , S. 2004 . Advances in Characterization of Size-Resolved Organic Matter in Marine Aerosol Over the North Atlantic . J. Geophys. Res. , 109 : 14 doi:10.1029/2004JD005137
- Chastain , J. 1992 . Handbook of X-ray Photoelectron Spectroscopy Perkin-Elmer Corporation, Waltham, MA
- Decesari , S. , Facchini , M. C. , Fuzzi , S. and Tagliavini , E. 2000 . Characterization of Water Soluble Organic Compounds in Atmospheric Aerosol: A New Approach . J. Geophys. Res. , 105 : 1481 – 1489 .
- Decesari , S. , Facchini , M. C. , Matta , E. , Lettin , F. , Mircea , M. Fuzzi , S. , . 2001 . Chemical Features and Seasonal Variation of Fine Aerosol Water-Soluble Organic Compounds in the Po Valley Italy . Atmos. Environ. , 5 : 3691 – 3699 .
- Decesari , S. , Mircea , M. , Cavalli , F. , Fuzzi , S. , Moretti , F. Tagliavini , E. , . 2007 . Source Attribution of Water-Soluble Organic Aerosol by Nuclear Magnetic Resonance Spectroscopy . Environ. Sci. Technol. , 41 : 2479 – 2484 .
- Dinar , E. , Taraniuk , I. , Graber , E. R. , Anttila , T. , Mentel , T. F. and Rudich , Y. 2007 . Hygroscopic Growth of Atmospheric and Model Humic-Like Substances . J. Geophys. Res. , 112 :13, doi:10.1029/2006JD007442
- Dinar , E. , Taraniuk , I. , Graber , E. R. , Katsman , S. , Moise , T. Anttila , T. , . 2006 . Cloud Condensation Nuclei Properties of Model and Atmospheric HULIS . Atmos. Chem. Phys. , 6 : 2465 – 2481 .
- Ding , X. , Zheng , M. , Edgerton , E. S. , Jansen , J. J. and Wang , X. 2008 . Contemporary or Fossil Origin: Split of Estimated Secondary Organic Carbon in the Southeastern United States . Environ. Sci. Technol. , 42 : 9122 – 9128 .
- Duan , J. , Tan , J. , Cheng , D. , Bi , X. , Deng , W. Sheng , G. , . 2007 . Sources and Characteristics of Carbonaceous Aerosol in Two Largest Cities in Pearl River Delta Region, China . Atmos. Environ. , 41 : 2895 – 2903 .
- Duarte , R. M. B. O. , Santos , E. B. H. , Pio , C. A. and Duarte , A. C. 2007 . Comparison of Structural Features of Water-Soluble Organic Matter from Atmospheric Aerosols with those of Aquatic Humic Substances . Atmos. Environ. , 41 : 8100 – 8113 .
- Duarte , R. M. B. O. , Silva , A. M. S. and Duarte , A. C. 2008 . Two-Dimensional NMR Studies of Water-Soluble Organic Matter in Atmospheric Aerosols . Environ. Sci. Technol. , 42 : 8224 – 8230 .
- Facchini , M. C. , Decesari , S. , Mircea , M. , Fuzzi , S. and Loglio , G. 2000 . Surface Tension of Atmospheric Wet Aerosol and Cloud/Fog Droplets in Relation to their Organic Carbon Content and Chemical Composition. . Atmos. Environ. , 34 : 4853 – 4857 .
- Freyer , H. D. , Kley , D. , Volz-Thomas , A. and Kobel , K. 1993 . On the Interaction of Isotopic Exchange Processes with Photochemical Reactions in Atmospheric Oxides of Nitrogen . J. Geophys. Res. , 98 : 14791 – 14796 .
- Gelencśer , A. , Hoffer , A. , Krivácsy , Z. , Kiss , G. , Molnar , A. and Meszaros , E. 2000 . On the Possible Origin of Humic Matter in Fine Continental Aerosol . J. Geophys. Res. , 107 : 6 doi:10.1029/2001JD001299
- Graber , E. R. and Rudich , Y. 2006 . Atmospheric HULIS: How Humic-Like are they? A Comprehensive and Critical Review . Atmos. Chem. Phys. , 6 : 729 – 753 .
- Graham , B. , Mayol-Bracero , O. L. , Guyon , P. , Roberts , G. C. , Decesari , S. Facchini , M. C. 2002 . Water-Soluble Organic Compounds in Biomass Burning Aerosols Over Amazonia 1. Characterization by NMR and GC-MS . J. Geophys. Res. , 107 : 16 doi:10.1029/2001JD000336
- Haselbach , L. M. and Ma , S. 2008 . Potential for Carbon Adsorption on Concrete: Surface XPS Analysis . Environ. Sci. Technol. , 42 : 5329 – 5334 .
- Havers , N. , Burba , P. , Lambert , J. and Klockow , D. 1998 . Spectroscopic Characterization of Humic-Like Substances in Airborne Particulate Matter . J. Atmos. Chem. , 47 : 45 – 54 .
- Huang , X. F. , Yu , J. Z. , He , L. Y. and Yuan , Z. 2006 . Water-Soluble Organic Carbon and Oxalate in Aerosols at a Coastal Urban Site in China: Size Distribution Characteristics Sources and Formation Mechanisms . J. Geophys. Res. , 111 : 11 doi:10.1029/2006JD007408
- IPCC (Intergovernmental Panel on Climate Change) . 2001 . 3rd Assessment Report , Cambridge University Press, Cambridge .
- Kelemen , S. R. , Afeworki , M. , Gorbaty , M. L. , Kwiatek , P. J. , Solum , M. S. , Hu , J. Z. and Pugmire , R. J. 2002 . XPS and 15N NMR Study of Nitrogen Forms in Carbonaceous Solids . Energy & Fuels , 16 : 1507 – 1515 .
- Kelemen , S. R. , Gorbaty , M. L. and Kwiatek , P. J. 1994 . Quantification of Nitrogen Forms in Argonne Premium Coal . Energy & Fuels , 8 : 896 – 906 .
- Kelly , S. D. , Stein , C. and Jickells , T. D. 2005 . Carbon and Nitrogen Isotopic Analysis of Atmospheric Organic Matter . Atmos. Environ. , 39 : 6007 – 6011 .
- Kiss , G. , Tombacz , E. and Hansson , H. C. 2005 . Surface Tension Effects of Humic-Like Substances in the Aqueous Extract of Troposphere Fine Aerosol . J. Atmos. Chem. , 50 : 279 – 294 .
- Kiss , G. , Tombacz , E. , Varga , B. , Alsberg , T. and Persson , L. 2003 . Estimation of the Average Molecular Weight of Humic-Like Substances Isolated from Fine Atmospheric Aerosol . Atmos. Environ. , 37 : 3783 – 3794 .
- Kiss , G. , Varga , B. , Galambos , I. and Ganszky , I. 2002 . Characterization of Water-Soluble Organic Matter Isolated from Atmospheric Fine Aerosol . J. Geophys. Res. , 107 : 8 doi:10.1029/2001JD000603
- Kiss , G. , Varga , B. , Gelencser , A. , Krivácsy , Z. , Molnar , A. Alsberg , T. , . 2001 . Characterisation of Polar Organic Compounds in Fog Water . Atmos. Environ. , 35 : 2193 – 2200 .
- Krivácsy , Z. , Gelencser , A. , Kiss , G. , Meszaros , E. , Molnar , A. Hoffer , A. , . 2001 . Study on the Chemical Character of Water-Soluble Organic Compounds in fine Atmospheric Aerosol at the Jungfraujoch . J. Atmos. Chem. , 39 : 235 – 259 .
- Krivácsy , Z. , Kiss , G. , Ceburnis , D. , Jennings , G. , Maenhaut , W. Salma , I. , . 2008 . Study of Water-Soluble Atmospheric Humic Matter in Urban and Marine Environments . Atmos. Res. , 87 : 1 – 12 .
- Krivácsy , Z. , Kiss , G. , Varga , B. , Galambos , I. , Sárvári , Zs. , Gelencsér , A. , Molnár , Á. , Fuzzi , S. , Facchini , M. C. , Zappoli , S. , Andracchio , A. , Alsberg , T. , Hansson , H.-C. and Persson , L. 2000 . Study of Humic-Like Substances in Fog and Interstitial Aerosol by Size-Exclusion Chromatography and Capillary Electrophoresis, Atmos . Environ. , 34 : 4273 – 4281 .
- Krolla , J. H. and Seinfeld , J. H. 2008 . Chemistry of Secondary Organic Aerosol: Formation and Evolution of Low-Volatility Organics in the Atmosphere . Atmos. Environ. , 42 : 3593 – 3624 .
- Leenheer , J. A. , Brown , G. K. , Maccarthy , P. and Caraniss , S. E. 1998 . Models of Metal Binding Structures in Fulvic Acid from the Suwannee River, Georgia . Environ. Sci. Technol. , 32 : 2410 – 2416 .
- Li , D. , Wang , X. , Mo , J. , Sheng , G. and Fu , J. 2007 . Soil Nitric Oxide Emissions from Two Subtropical Humid Forests in South China. . J. Geophys. Res. , 112 : 9 doi:10.1029/2007JD008680
- Limbeck , A. , Handler , M. , Neuberger , B. , Klatzer , B. and Puxbaum , H. 2005 . Carbon-Specific Analysis of Humic-Like Substances in Atmospheric Aerosol and Precipitation Samples . Anal. Chem. , 77 : 7288 – 7293 .
- Lin , P. , Engling , G. and Yu , J. Z. 2010 . Humic-Like Substances in Fresh Emissions of Rice Straw Burning and in Ambient Aerosols in the Pearl River Delta Region, China . Atmos. Chem. Phys. , 10 : 6487 – 6500 .
- Lin , P. , Huang , X.-F. , He , L.-Y. and Yu , J. Z. 2009 . Abundance and Size Distribution of HULIS in Ambient Aerosols at a Rural Site in South China . J. Aerosol Sci. , 41 : 74 – 87 .
- Linuma , Y. , Müller , C. , Böge , O. , Gnauk , T. and Herrmann , H. 2007 . The Formation of Organic Sulfate Esters in the Limonene Ozonolysis Secondary Organic Aerosol (SOA) under Acidic Conditions . Atmos. Environ. , 41 : 5571 – 5583 .
- Lopes , C. B. , Abreu , S. , Valega , M. , Duarte , R. M. B. O. , Pereira , M. E. and Duarte , A. C. 2006 . The Assembling and Application of an Automated Segmented ?ow Analyzer for the Determination of Dissolved Organic Carbon Based on UV-Persulphate Oxidation . Anal. Lett. , 39 : 1979 – 1992 .
- Lu , X. Q. , Hanna , J. V. and Johnson , W. D. 2000 . Source Indicators of Humic Substances: An Elemental Composition, Solid State 13C CP/MAS NMR and Py-GC/MS Study . Appl. Geochem. , 15 : 1019 – 1033 .
- Lukács , H. , Gelencser , A. , Hammer , S. , Puxbaum , H. , Pio , C. Legrand , M. , . 2007 . Seasonal Trends and Possible Sources of Brown Carbon Based on 2-Year Aerosol Measurements at Six Sites in Europe . J. Geophys. Res. , 112 : 9 doi:10.1029/2006JD008151
- Ma , S. , Peng , P. , Song , J. , Bi , X. , Zhao , J. He , L. , . 2010 . Seasonal and Spatial Changes of Free and Bound Organic Acids in Total Suspended Particles in Guangzhou, China . Atmos. Environ. , 44 : 5460 – 5467 .
- Matthias-Maser , J. and Jaenicke , R. 2000 . The Size Distribution of Primary Biological Aerosol Particles in the Multiphase Atmosphere . Aerobiologia , 16 : 207e210
- Mayol-Bracero , O. L. , Guyon , P. , Graham , B. , Roberts , G. , Andreae , M. O. Decesari , S. , . 2002 . Water-Soluble Organic Compounds in Biomass Burning Aerosols Over Amazonia 2: Apportionment of the Chemical Composition and Importance of the Polyacidic Fraction . J. Geophys. Res. , 107 : 15 doi:10.1029/2001JD000522
- Miyazaki , Y. , Kondo , Y. , Han , S. , Koike , M. , Kodama , D. Komazaki , Y. , . 2007 . Chemical Characteristics of Water-Soluble Organic Carbon in the Asian Outflow . J. Geophys. Res. , 112 : 18 doi:10.1029/2007JD009116
- Monteil-Rivera , F. , Brouwer , E. B. , Masset , S. , Deslandes , Y. and Dumonceau , J. 2000 . Combination of X-ray Photoelectron and Solid-State 13C Nuclear Magnetic Resonance Spectroscopy in the Structural Characterization of Humic Acids . Anal. Chim. Acta , 424 : 243 – 255 .
- Nozière , B. , Ekatröm , S. , Alsberg , T. and Holmström , S. 2010 . Radical-Initiated Formation of Organosulfates and Surfactants in Atmospheric Aerosols . Geophys. Res. Lett. , 37 : 6 doi:10.1029/2009GL041683
- Olivella , M. A. , del Rio , J. C. , Palacios , J. M. , Vairavamurthy , A. and de las Heras , F. X. C. 2002a . Characterization of Humic Acid from Leonardite Coal: An Integrated Study of PY-GC-MS, XPS and XANES Techniques . J. Anal. Appl. Pyroly. , 63 : 59 – 68 .
- Olivella , M. A. , Palacios , J. M. , Vairavamurthy , A. , del Rio , J. C. and de las Heras , F. X. C. 2002b . A Study of Sulfur Functionalities in Fossil Fuels Using Destructive-(ASTM and Py-GC-MS) and Non-Destructive-(SEm-EDX, XANES and XPS) Techniques . Fuel , 81 : 405 – 411 .
- Pietrzak , R. and Wachowska , H. 2006 . The Influence of Oxidation with HNO3 on the Surface Composition of High-Sulphur Coals: XPS Study . Fuel Processing Tech. , 87 : 1021 – 1029 .
- Raes , F. , Van Dingenen , R. , Vignatti , E. , Wilson , J. , Putaud , J. P. , Seinfeld , J. H. and Adams , P. 2000 . Formation and Cycling of Aerosols in the Global Troposphere . Atmos. Environ. , 34 : 4215 – 4240 .
- Ramanathan , V. , Chung , C. , Kim , D. , Bettge , T. , Buja , L. Kiehl , J. T., . 2005 . Atmospheric Brown Clouds: Impacts on South Asian Climate and Hydrological Cycle . Proc. Nat. Acad. Sci. , 102 : 5326 – 5333 .
- Romero , F. and Oehme , M. 2005 . Organosulfates: A New Component of Humic-Like Substances in Atmospheric Aerosols? . J. Atmos. Chem. , 52 : 283 – 294 .
- Russell , K. M. , Galloway , J. N. , Macko , S. A. , Moody , J. L. and Scudlark , J. R. 1998 . Sources of Nitrogen in Wet Deposition to the Chesapeake Bay Region . Atmos. Environ. , 32 : 2453 – 2465 .
- Salma , I. , Mészáros , T. , Maenhaut , W. , Vass , E. and Majer , Z. 2010 . Chirality and the Origin of Atmospheric Humic-Like Substances . Atmos. Chem. Phys. , 10 : 1315 – 1327 .
- Salma , I. , Ocskay , R. , Varga , I. and Maenhaut , W. 2006 . Surface Tension of Atmospheric Humic-Like Substances in Connection with Relaxation, Dilution, and Solution pH. . J. Geophys. Res. , 111 : 7 doi:10.1029/2005JD007015
- Samburova , V. , Szidat , S. , Hueglin , C. , Fisseha , R. , Baltensperger , U. , Zenobi , R. and Kalberer , M. 2005 . Seasonal Variation of High-Molecular-Weight Compounds in the Water-Soluble Fraction of Organic Urban Aerosols . J. Geophy. Res. , 110 : D23210
- Seinfeld , J. H. 1986 . Atmospheric Chemistry and Physics of Air Pollution , New York : Wiley .
- Sempéré , R. and Kawamura , K. 1994 . Comparative Distributions of Dicarboxylic Acids and Related Polar Compounds in Snow, Rain Andaerosols from Urban Atmosphere . Atmos. Environ. , 28 : 449 – 459 .
- Song , J. and Peng , P. 2009 . Surface Characterization of Aerosol Particles in Guangzhou, China: A study by XPS . Aerosol Sci. Technol. , 43 : 1230 – 1242 .
- Song , J. , Peng , P. and Huang , W. 2002 . Black Carbon and Kerogen in Soils and Sediments: 1. Quantification and Characterization . Environ. Sci. Tech. , 36 : 3960 – 3967 .
- Song , J. , Peng , P. and Huang , W. 2005 . Characterization of Humic Acid Like Materials Isolated from the Humin Fraction of a Topsoil . Soil Sci. , 170 : 599 – 611 .
- Spitzy , A. and Leenheer , J. A. 1988 . “ Dissolved Organic Carbon in Rivers ” . In SCOPE 42: Biogeochemistry of Major World Rivers , Edited by: Degens , E. T. , Kempe , S. and Richey , J. E. New York : Wiley . Chap. 9. http://www.icsu-scope.org/downloadpubs/scope42/chapter09.html
- Stone , E. A. , Hedman , C. J. , Sheesley , R. J. , Shafer , M. M. and Schauer , J. J. 2009 . Investigating the Chemical Nature of Humic-Like Substances (HULIS) in North American Atmospheric Aerosols by Liquid Chromatography Tandem Mass Spectrometry . Atmos. Environ. , 43 : 4205 – 4213 .
- Sullivan , A. P. , Weber , R. J. , Clements , A. L. , Turner , J. R. , Bae , M. S. and Schauer , J. J. 2004 . A Method for On-Line Measurement of Water Soluble Organic Carbon in Ambient Aerosol Particles: Results from an Urban Site . Geophys. Res. Lett. , 31 : 4 doi:10.1029/2004GL019681
- Surratt , J. D. , Kroll , J. H. , Kleindienst , T. E. , Edney , E. O. , Claeys , M. Sorooshian , A. , . 2007 . Evidence for Organosulfates in Secondary Organic Aerosol . Environ. Sci. Technol. , 41 : 517 – 527 .
- Szidat , S. , Jenk , T. M. , Gäggeler , H. W. , Synal , H.-A. , Fisseha , R. Baltensperger , U. , . 2004 . Radiocarbon (14C)-Deduced Biogenic and Anthropogenic Contributions to Organic Carbon (OC) of Urban Aerosols from Zürich, Switzerland . Atmos. Environ. , 38 : 4035 – 4044 .
- Tan , J.-H. , Duan , J.-C. , Chen , D.-H. , Wang , X.-H. , Guo , S.-J. Bi , X.-H., . 2009 . Chemical Characteristics of Haze During Summer and Winter in Guangzhou . Atmos. Res. , 94 : 238 – 245 .
- Taraniuk , I. , Graber , E. R. , Kostinski , A. and Rudich , Y. 2007 . Surfactant Properties of Atmospheric and Model Humic-Like Substances (HULIS) . Geophys. Res. Lett. , 34 : 5 doi:10.1029/2007GL029576
- Turpin , B. J. , Saxena , P. and Andrews , E. 2001 . Measuring and Simulating Particulate Organics in the Atmosphere: Problems and Prospects . Atmos. Environ. , 34 : 2983 – 3013 .
- Vander Wal , R. L. , Bryg , V. M. and Hays , M. D. 2011 . XPS Analysis of Combustion Aerosols for Chemical Composition, Surface Chemistry, and Carbon Chemical State . Anal. Chem. , 83 : 1924 – 1930 .
- Varga , B. , Kiss , G. , Ganszky , I. , Gelencser , A. and Krivácsy , Z. 2001 . Isolation of Water-Soluble Organic Matter from Atmospheric Aerosol . Talanta , 55 : 561 – 572 .
- Wagner , C. D. , Davis , L. E. , Zeller , M. V. , Taylor , J. A. , Raymond , R. H. and Gale , L. H. 1981 . Empirical Atomic Sensitivity Factors for Quantitative Analysis by Electron Spectroscopy for Chemical Analysis . Surf. Interface Anal. , 3 : 211 – 225 .
- Wang , G. , Kawamura , K. , Lee , S. C. , Ho , K. F. and Cao , J. J. 2006 . Molecular, Seasonal, and Spatial Distributions of Organic Aerosols from fourteen Chinese cities . Environ. Sci. Technol. , 40 : 4619 – 4625 .
- Wu , D. , Tie , X. , Li , C. , Ying , Z. , Lau , A. K. Huang , J. , . 2006 . An Extremely Low Visibility Event Over the Guangzhou Region: A Case Study . Atmos. Environ. , 39 : 6568 – 6577 .
- Wu , T. , Wang , X. , Li , D. and Yi , Z. 2010 . Emission of Volatile Organic Sulfur Compounds (VOSCs) During Aerobic Decomposition of Food Wastes . Atmos. Environ. , 44 : 5065 – 5071 .
- Yeatman , S. G. , Spokes , L. J. , Dennis , P. F. and Jickells , T. D. 2001 . Comparison of Aerosol Nitrogen Isotopic Composition at Two Polluted Coastal Sites . Atmos. Environ. , 35 : 1307 – 1320 .
- Yi , Z. , Wang , X. , Ouyang , M. , Zhang , D. and Zhou , G. 2010 . Air-Soil Exchange of Dimethyl Sulfide, Carbon Disulfide, and Dimethyl Disulfide in three Subtropical Forests in South China . J. Geophys. Res. , 115 : 7 doi:10.1029/2010JD014130
- Yi , Z. , Wang , X. , Sheng , G. and Fu , J. 2008 . Exchange of Carbonyl Sulfide (OCS) and Dimethyl Sulfide (DMS) between Rice Paddy Fields and the Atmosphere in Subtropical China . Agr. Ecosys. Environ. , 123 : 116 – 124 .
- Yu , J. Z. , Yang , H. , Zhang , H. Y. and Lau , A. K. H. 2004 . Size Distributions of Water-Soluble Organic Carbon in Ambient Aerosols and its Size-Resolved Thermal Characteristics . Atmos. Environ. , 38 : 1061 – 1071 .
- Zappoli , S. , Andracchio , A. , Fuzzi , S. , Facchini , M. C. , Gelencser , A. Kiss , G. , . 1999 . Inorganic Organic and Macromolecular Components of Fine Aerosol in Different Areas of Europe in Relation to their Water Solubility . Atmos. Environ. , 33 : 2733 – 2743 .
- Ziese , M. , Wex , H. , Nilsson , E. , Salma , I. , Ocskay , R. Hennig , T. , . 2008 . Hygroscopic Growth and Activation of HULIS Particles: Experimental Data and a New Iterative Parameterization Scheme for Complex Aerosol Particles . Atmos. Chem. Phys. , 8 : 1855 – 1866 .