Abstract
The filter artifact is a significant source of error in gravimetric measurements of particulate matter (PM) exhaust. However, only a few studies on the subject exist. Results from these studies show a large discrepancy mainly because the experiments were performed using real diesel vehicle exhaust with varying exhaust composition. In this study, a setup for mixing diesel-like soot and hydrocarbon vapor was constructed for generating a stable exhaust aerosol with adjustable composition. The particle size distribution of the diesel-fueled soot generator (GMD [geometric mean diameter] adjustable between 27 and 164 nm) was found to represent “real” exhaust particulate emission. This setup was applied for studying the filter artifact on Teflon-coated glass fiber filters using pentadecane as the hydrocarbon vapor. Experiments were performed using particle and hydrocarbon concentrations of 130–700 μg/m3 and 10–12 ppm, respectively. It was found that the particle concentration of the aerosol affects the filter artifact. At lower particle concentrations, more hydrocarbon adsorption was detected. In the absence of particles, the adsorption was highest. Furthermore, filter soot load, corresponding to 0.13%–0.66% of the clean filter mass, was found to affect adsorption. Sooty filters adsorbed less vapor than clean filters. However, increasing the soot load resulted in more adsorption. Moreover, it was found that the backup filter serves as a reasonable estimate of the filter artifact only for low particle concentrations and filter soot loads. These results indicate that the filter soot load is an important parameter influencing the filter artifact, and therefore, it should be considered when performing gravimetric sampling. The setup was proven to be a unique tool for quantitative studies of the filter artifact.
Copyright 2012 American Association for Aerosol Research
1. INTRODUCTION
Particulate emissions from vehicles are a major hazard to human health and the environment (Utell and Frampton Citation2000; European Commission Citation2001; Sydbom et al. Citation2001). Therefore, increasingly stringent regulations, such as the European emission standards, have been introduced to limit these emissions. As a consequence, the particulate matter (PM) mass emission from diesel vehicles has dramatically reduced—making the current measurement method, which is based on weighing the PM collected on a filter, challenging as the PM mass approaches the sensitivity of the gravimetric method (Vouitsis et al. Citation2003). Furthermore, the adsorption of organic vapors onto the filter (positive artifact) and volatilization of semivolatile organic compounds from the filter (negative artifact) introduce errors in measuring low PM concentrations.
The effect of filter artifacts on the results of gravimetric sampling has been extensively studied in the atmospheric measurement community (McDow and Huntzicker Citation1990; Hart and Pankow Citation1994; Turpin et al. Citation1994, Citation2000; Mader et al. Citation2001; Mader and Pankow Citation2001; Viana et al. Citation2006; Vecchi et al. Citation2009; Chow et al. Citation2010). However, in exhaust emission measurements, the filter artifact has not gained much attention because the PM mass collected on filters has been large enough to make the effect insignificant. As the PM emission levels are dropping, the filter artifact has become significant. Quartz fiber filters have been found to be prone to filter artifacts (Schauer et al. Citation1999; Lipsky and Robinson Citation2006), and therefore, current legislation recommends using Teflon-coated glass fiber or membrane filters for PM exhaust measurements. However, recent studies show that Teflon filters also suffer from sampling artifacts (Chase et al. Citation2004; Khalek Citation2005). Chase et al. found a positive sampling artifact of 10%–20% for Teflon membrane filters and 30%–50% for Teflon-coated glass fiber filters. The positive artifact is believed to be dominant over the negative one when sampling diluted vehicle exhaust (Chase et al. Citation2004).
The adsorption of gas-phase compounds onto the filter surface is a complex process that depends on many factors, e.g., temperature, relative humidity (RH), concentration and composition of vapors, filter type, particle loading, and whether sufficient amount of vapor has been delivered to the filter in order to reach equilibrium between the gas phase and filter media (Mader and Pankow Citation2001). A commonly used method for characterizing the positive artifact is to use a backup filter, which is placed behind the actual sampling filter (front filter; Turpin et al. Citation2000; Chase et al. Citation2004; Lipsky and Robinson Citation2006). The front filter collects practically all PM and the backup filter is exposed only to the gas-phase compounds and thus serves as an estimate of the vapor adsorption onto the front filter. This method is valid only if (1) both filters have reached equilibrium with the gas phase, (2) the negative artifact is insignificant, and (3) the vapor adsorption is similar for the front and backup filters. The first assumption is valid if sufficient time for sampling is allowed and the second assumption is reasonable since the positive artifact is dominant over the negative artifact, but the third assumption is questionable since the filter loading is known to affect gas-phase adsorption (Mader and Pankow Citation2001).
Another means of characterizing the positive filter artifact is to use a denuder in front of the sampling filter to remove gas-phase organics from the aerosol (Schauer et al. Citation1999, Turpin et al. Citation2000; Lipsky and Robinson Citation2006). Thus, the positive artifact is given as the difference in the mass of an undenuded filter (collects both the PM and some gas-phase organics) and a denuded filter (collects only the PM). It should be noted that the use of a denuder disturbs the gas-particle equilibrium causing volatilization of PM from the sampling filter (Turpin et al. Citation2000). To compensate this effect, an adsorbent bed downstream of the filter is used for collecting the evaporated PM. However, the denuder system should have 100% vapor collection efficiency or the efficiency should be carefully characterized, both of which is challenging since the denuder efficiency depends on vapor composition and sampling conditions (Kamens and Coe Citation1997; Cui et al. Citation1998; Lane et al. Citation1998). Moreover, particle losses in the denuder should be minimized and/or carefully characterized.
Studies on the effect of the filter artifact in gravimetric measurements show a large discrepancy even for the same filter material (Schauer et al. Citation1999; Chase et al. Citation2004; Khalek Citation2005; Lipsky and Robinson Citation2006). This may be to some extent explained by the shortcomings of the measurement methods mentioned earlier, but probably to a greater extent by the varying exhaust composition encountered during emission tests of different vehicles. In order to gain a deeper understanding of the factors affecting the filter artifact, a reproducible and stable source of exhaust aerosol with adjustable particle and gas-phase composition is needed. In this article, we introduce such a source based on mixing diesel-like soot from a soot generator and hydrocarbon content from a hydrocarbon generator. The setup is applied for studying the positive filter artifact on Teflon-coated glass fiber filters using particle and hydrocarbon concentrations of 130–700 μg/m3 and 10–12 ppm, respectively. These concentrations correspond roughly to limit values of the Euro 4 standard for passenger cars and Euro V standard for heavy-duty vehicles under low dilution ratio and constant volume sampling (CVS) conditions. Moreover, the similarity of generated particles to “real” diesel exhaust particles is discussed.
2. GENERATORS
2.1. Soot Generator
The soot aerosol generator was made from a commercial diesel-fueled vehicle heater (Webasto Air Top 2000 ST). The burner was modified so that the fuel pump and the combustion air are controllable. By controlling the combustion air between 35 and 50 L/min, and keeping the fuel pump frequency constant at 1 Hz, the soot particle size distribution can be controlled in the mobility size range GMD (geometric mean diameter) 27–164 nm with GSD (geometric standard deviation) 1.73–2.5 and raw exhaust particle number of 1.94 × 106–4.52 × 108 /cm3. The generated size distribution was found to be lognormal and transmission electron microscope images confirmed that the generated soot particles were fractal-like agglomerates with primary sphere size around 20–30 nm. Thus, the generated soot particles resemble real diesel exhaust particles. The output particle number concentration was found very stable having a coefficient of variation (i.e., normalized standard deviation) of 4%, which is similar to the commercial soot generator Combustion Aerosol STandard (CAST, Jing Ltd.) with a coefficient of variation of 4% (Schlatter Citation2002).
2.2. Hydrocarbon Generator
The operating principle of the hydrocarbon generator is based on a bubbler saturator design, in which nitrogen gas enters the bottom of a saturator vessel and is allowed to bubble up through the liquid hydrocarbon in order to achieve saturation. The saturator is submerged in a temperature-controlled bath (LAUDA P40) so that the vapor pressure (and thus concentration) of the generated hydrocarbon vapor can be controlled by controlling the temperature of the bath. The generator is designed to be used at saturator temperatures between 25°C and 90°C. In this study, pentadecane (C15H32) was used as the hydrocarbon compound, since it closely corresponds to the average carbon number of diesel exhaust (Sakurai et al. Citation2003). Diesel exhaust also comprises heavier hydrocarbons, e.g., unburned lubrication oil (Kittelson Citation1998). These were not considered in this study as they are more challenging, owing to their semivolatile nature. The generator was found to fully saturate nitrogen gas with pentadecane, which enabled a very stable output concentration that fluctuated only 0.2 ppm. The pentadecane concentration was measured using a gas analyzer (Gasmet FTIR). Details on the performance tests of the generators are provided in the online Supplemental Information.
3. STUDY OF THE FILTER ARTIFACT
3.1. Experimental Setup
The constructed experimental setup is depicted in . In the setup, a mixture of diesel-like soot particles and hydrocarbon vapor is produced by mixing particles from the soot generator with vapor from the hydrocarbon generator. The mixing is performed in an ejector ensuring homogeneity of the generated mixture. Both generators can be bypassed, allowing filter sampling with only particles or vapor. Three kinds of aerosol/gas mixtures were used in the tests: (1) only pentadecane in the carrier gas, (2) only soot particles in the aerosol, and (3) both hydrocarbons and soot particles in the aerosol.
In order to avoid condensation of the vapor, heated lines are used at the hydrocarbon generator output and at the gas analyzer inlet. Moreover, all lines before the filter holder are insulated. The heater is adjusted so that the temperature of the aerosol entering the filter holder is between 47°C and 52°C. Thus, the sample temperature was in conformance with regulatory requirements of 47°C ± 5°C. Moreover, the filter holder is heated so as to maintain the aerosol at the aforementioned temperature.
In the studies with soot particles present, a part of the soot generator exhaust was taken as the test aerosol. The exhaust was first diluted with dry and purified compressed air so that there was no condensation of water at ambient laboratory temperature. The flow entered a mixing chamber (volume 6.6 L) that stabilizes the aerosol output. After that, there was a thermodenuder (Dekati® Thermodenuder), operating at 270°C, to remove hydrocarbons and other possible semivolatile components from the diluted exhaust.
The vapor concentration of the generated aerosol was measured with a gas analyzer (Gasmet FTIR), while the particle size distribution was measured with a scanning mobility particle sizer (SMPS; TSI 3071, TSI CPC 3775) and the particle mass concentration with the Dekati Mass Monitor (DMM). The SMPS was operated at 0.6/6 (polydisperse/sheath) L/min flow rates. The flow rates through the DMM and the gas analyzer were 8.6 L/min and 4 L/min, respectively. A sampling system (Gasmet Portable Sampling System), comprising of a heated pump and a heated filter, was used upstream of the gas analyzer to provide a preconditioned sample to the gas analyzer. The filter flow rate was 10 L/min corresponding to a filter face velocity of about 13 cm/s. Teflon-coated glass fiber filters (Pall Corp.; Type: Emfab TX40HI20-WW; Part no. 7221) with a diameter of 47 mm were used in filter artifact studies. The filters consist of pure borosilicate glass microfibers reinforced with woven glass cloth and coated with Teflon. These filters are specifically designed for diesel exhaust measurements.
3.2. Gravimetric Sampling
Before performing gravimetric sampling, the filter holder was bypassed until the particle and/or vapor concentration of the output aerosol was stable and the temperature of the aerosol and the holder was stable at a temperature between 47°C and 52°C. After stabilization, gravimetric sampling was done by redirecting the aerosol flow through the filter holder for a defined sampling time. Thereafter, the measurement was repeated with the generators bypassed in order to quantify the effect of background contamination on the filter mass. The background contamination was typically 10 μg for a 20-min measurement. The result of gravimetric sampling was corrected for this effect by subtracting the filter mass gain caused by background contaminants from the result.
The mass sampled on the filter was determined as the difference in the mass of the filter before and after sampling. The filters were weighed using a microbalance (Sartorius CCE6) with 0.1-μg resolution. Each filter was weighed at least three times. The standard deviation of the weighing was typically 1 μg. Before weighing, the filters were allowed to equilibrate for at least 24 h in the temperature- and humidity-controlled laboratory (20°C ± 1°C, 45% ± 3% RH), where the weighing was performed (Lassila et al. Citation2011). This was done because right after the collection, the filter masses were significantly higher, due to humidity and/or excess hydrocarbons, than after proper stabilization. The effect of static charge on weighing was studied by comparing weighing results before and after neutralizing a filter sample. No effect was found.
3.3. Experiments
The adsorption of hydrocarbon vapor on filters (positive filter artifact) was studied with and without particles from the soot generator. Experiments without particles (soot generator bypassed) were performed using both clean and sooty filters in order to study the effect of filter soot load on adsorption. The sooty filters were prepared by sampling soot from the soot generator. Sampling time was adjusted in order to achieve soot accumulation of 110, 200, and 590 μg that corresponds to 0.13%, 0.22%, and 0.66% of the clean filter mass, respectively. Thus, the filter mass loads conform to the ISO 16183 standard (ISO Citation2002), which recommends a minimum load of 0.13%. In addition, the effect of sampling time on the filter artifact was studied for clean and sooty filters.
Filter artifact studies with a test aerosol containing particles was carried out in order to simulate “real” exhaust measurements. In these experiments, two filters were used in series. The filter artifact was studied as follows. First, the initially clean filters were sampled using only particles (hydrocarbon generator bypassed). Thereafter, the measurement was repeated with a test aerosol containing particles and vapor (no bypass). As the particle mass concentration of the test aerosol was kept constant, the filter artifact was found as the difference in filter mass gain of these measurements. This value was compared with the backup filter mass gain from the latter measurement in order to evaluate the feasibility of using the backup filter as an estimate of the filter artifact of the front filter. The measurements were performed using two soot concentrations, 130 and 700 μg/m3, in order to find out the effect of soot concentration on the filter artifact.
A sampling time of 20 min was used for all filter artifact experiments (except for the studies on the effect of sampling time). Besides ensuring that sufficient soot mass was gathered for accurately weighing the filters, this sampling time allowed the filters to reach equilibrium with the gas phase. The temperature of the hydrocarbon generator bath was about 50°C, which resulted in a pentadecane concentration of 10–12 ppm at the sampling filter. A summary of the performed experiments is shown in .
TABLE 1 Summary of the performed experiments showing soot and hydrocarbon (HC) concentrations, initial filter soot load (expressed as the percentage of clean filter mass), sampling time, and associated figures presenting the results
FIG. 2 Effect of sampling time on filter artifact for initially clean filters (open squares) and sooty filters with 0.13% soot content (filled squares). Arrows indicate filter artifact for pentadecane concentrations other than 12 ppm. Error bars indicate the estimated uncertainty of measurement results.
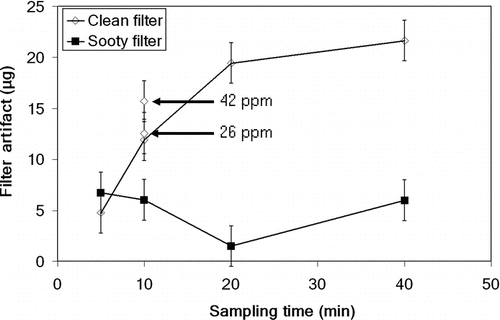
4. RESULTS OF THE FILTER ARTIFACT STUDY
The adsorption of pentadecane on clean filters increased with sampling time until saturation was reached at about 20 min with a pentadecane concentration of 12 ppm (). Based on the result, the adsorption of pentadecane appears to be a saturation process depending on the magnitude of available surface area and interacting materials. For the initially sooty filters, adsorption was far less, only 5 μg as compared with 20 μg for the clean filters. Moreover, the adsorption of pentadecane on sooty filters did not show time dependency for sampling times exceeding 5 min. The 20-min sampling point for the sooty filter is an outlier that may have resulted from loss of filter material during filter handling. The pentadecane concentration was found to have only a small effect on the filter artifact. The artifact was 12, 13, and 16 μg for 12, 26, and 42 ppm concentrations, respectively, when sampling for 10 min (). However, saturation conditions were not reached after 10 min of sampling. The effect of filter holder temperature on pentadecane adsorption was also studied with the initially clean filters in the temperature range 40°C–70°C (results not shown in the figure). However, no notable effect was found when sampling for 10 min. The error bars shown in and indicate the uncertainty related to sampling and weighing such small masses.
For filters with low soot loads (0.13% and 0.22% of the clean filter mass), the adsorption of pentadecane was much less than that for clean filters (), which is also observed in . But for high soot loads (0.66% of the clean filter mass), an adsorption artifact of 50 μg was observed. This is 2.5 times higher than for clean filters. The observed filter artifacts correspond to 1.3%, 3.6%, and 8.6% of the soot mass of the sooty filters with soot loads of 0.13%, 0.22%, and 0.66%, respectively.
The effect of the filter artifact when sampling a test aerosol containing both particles and pentadecane vapor is shown in for two different soot concentrations. For low soot concentrations (130 μg/m3), adding pentadecane to the test aerosol resulted in 11 μg higher filter mass gain, corresponding to 31% of the sampled PM. Correcting for this, using the backup filter mass gain of 15 μg would result in slightly underestimating the soot load on the front filter. For high soot concentrations (700 μg/m3), adding pentadecane did not affect the mass sampled on the front filter as the mass gain was practically equal with and without pentadecane. The backup filter mass gain was similar to the low soot concentration case, indicating that equilibrium between the filter and the gas phase was reached. In the case of high soot concentration, correcting the front filter mass gain with the backup filter mass gain would lead to underestimating the soot load on the front filter. The fact that no artifact was found at high concentrations also indicates that pentadecane remained in the gas phase and did not adsorb on particles. It is reasonable to assume that pentadecane remains in the gas phase also at lower particle concentrations. Therefore, the filter artifact found at low particle concentrations is due to gas-phase adsorption of pentadecane. The error bars of indicate the measurement uncertainty comprising the uncertainty of weighing and the uncertainty due to fluctuating particle concentration during and between measurements performed with and without pentadecane.
FIG. 3 Filter artifact for an initially clean filter and for filters with different soot loads (expressed as the percentage of clean filter mass). The pentadecane concentration was 12 ppm during the 20-min measurement. Photographs of the filters are shown in the subfigure. Error bars indicate the estimated uncertainty of measurement results. (Color figure available online.)
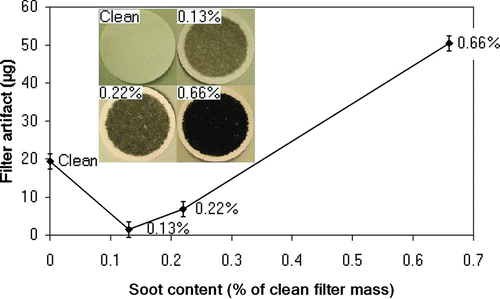
FIG. 4 Filter mass gain when sampling only soot (white columns) and a mixture of soot and pentadecane (dotted columns and artifact corrected result as checkered columns). The soot concentrations were kept constant at 130 μg/m3 (low soot content) and 700 μg/m3 (high soot content) and the pentadecane concentration was 10 ppm during the 20-min measurement. Error bars indicate the estimated uncertainty of measurement results.
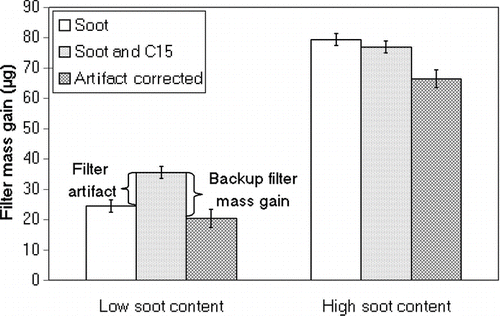
5. DISCUSSION
The self-made soot particle generator operates adjustably at a wide particle size distribution range. The particle size was found adjustable between GMD 27 and 164 nm with a GSD of 1.73–2.5 and raw exhaust particle number concentration of 1.94 × 106–4.52 × 108 /cm3. Comparing with some diesel engine exhaust studies, Vaaraslahti et al. (Citation2004) observed a soot mode number concentration of 1.58 × 107–1.96 × 107 /cm3 and a GMD of 50–70 nm with a Euro II heavy-duty diesel engine. Virtanen et al. (Citation2004) observed a GSD of 1.8–2.07 with a Euro 2 passenger car engine. Lähde et al. (Citation2011) reported a soot mode GMD of 26–67 nm and a GSD of 2.0–2.6 with a modern Euro 3B nonroad heavy-duty engine, both depending on fuel injection pressure. With a high injection pressure, the soot mode GMD decreased while the GSD increased. This applies to our soot generator, i.e., the smaller the GMD, the larger is the GSD. Overall, the soot generator is able to produce representative fractal-like soot particles with respect to particle size and distribution width as well as total particle number.
Filter artifact studies performed with clean filters using a test gas containing only pentadecane vapor show that filters adsorb hydrocarbon vapor until equilibrium between the filter medium and the gas phase is reached. The mass of adsorbed pentadecane reaches asymptotically a constant value. This adsorption behavior agrees with the adsorption mechanism presented by Mader and Pankow (Citation2001). For the initially sooty filters, the adsorption of pentadecane vapor was far less and equilibrium was already reached after 5 min of sampling.
Measurements performed with the sooty filters show that pentadecane adsorption was less for the sooty filters with low soot loads (0.13% and 0.22% of the clean filter mass) than for the initially clean filters. This indicates that the adsorption affinity of pentadecane for soot is less than that for the filter fibers. Moreover, the adsorption of pentadecane increases as the soot load increases. This is probably due to the increased effective surface area of the loaded filters, introducing more adsorption sites for pentadecane.
Filter artifact studies performed using a test aerosol containing particles and vapor show that soot particle concentration of the aerosol affects the adsorption of pentadecane onto a filter in such a way that lower particle concentrations yield more adsorption. This is reasonable because soot particles deposited on the filter occupy potential adsorption sites for pentadecane, and it was shown that pentadecane adsorb more readily on clean filters than on soot-loaded filters. For low particle concentrations (130 μg/m3), the positive artifact was 31% of the collected PM. This result is in good agreement with the positive artifact of 30%–50% reported by Chase et al. (Citation2004) for Teflon-coated glass fiber filters in experiments performed on heavy-duty diesel vehicles.
The use of a backup filter for estimating and correcting for the filter artifact of the front filter was found inadequate for high soot concentrations (700 μg/m3), as it would result in underestimating the soot mass on the front filter. As shown in this study, the adsorption of hydrocarbon vapor is less for filters exposed to soot particles (i.e., front filter) than for filters exposed only to vapor (i.e., backup filter). Therefore, the backup filter mass gain does not represent the vapor adsorbed on the front filter. However, for low particle concentrations (130 μg/m3), the backup filter gives a rather good estimate of the filter artifact of the front filter as long as sufficient sampling time is used for it to reach equilibrium with the gas phase.
It can be said that sampling soot particles reduces the filter artifact to a point where the increased effective surface area of the filter becomes large enough (promoting adsorption) to offset this effect. This indicates that in real measurements, where hydrocarbons and soot particles coexist, the adsorption depends on the ratio of the two. This implies that prolonged sampling times used for achieving higher soot loads on filters might even be detrimental for minimizing the effect of the filter artifact. If this can be generalized to diesel exhaust, the current PM measurement standards and regulation should define not only minimum filter loadings but also maximum filter loadings. However, it is important to stress that these experiments were performed using only one type of hydrocarbon present in diesel exhaust. More experiments are needed to verify these results using different kinds and different mixtures of hydrocarbons.
The particle and hydrocarbon vapor concentrations used in this study correspond roughly to limit values of the Euro 4 standard for passenger cars and the Euro V standard for heavy-duty vehicles under low dilution ratio and CVS conditions. The studied particle and hydrocarbon concentrations might not coexist in real applications, and furthermore, the hydrocarbon content of “real” diesel exhaust is a complex mixture of different compounds (Kittelson Citation1998). However, the presented experimental approach of generating a well-defined aerosol provides a means of systematically studying the influence of different parameters on the sampled PM. The obtained results are relevant in a qualitative manner since they show how particle concentration and filter soot load affect the adsorption of gas-phase hydrocarbons.
6. SUMMARY
An experimental setup for mixing diesel-like soot particles and hydrocarbon vapor was constructed with the aim of studying the effect of the filter artifact on gravimetric sampling. Studies with pentadecane showed that the developed method has the potential to obtain results with high scientific relevance and impact in vehicle exhaust emission measurements. The studied particle and vapor concentrations were 130–700 μg/m3 and 10–12 ppm, respectively, which correspond roughly to limit values of the Euro 4 standard for passenger cars and the Euro V standard for heavy-duty vehicles under low dilution ratio and CVS conditions.
The aerosol output of the setup was found very stable with a particle and vapor concentration output having a coefficient of variation of 0.3% and 4.0%, respectively. The particle size distribution of the self-made diesel-fueled soot generator was found to represent “real” exhaust particulate emission with respect to size (adjustable between 27 and 164 nm) and distribution width (GSD 1.73–2.5) as well as particle number (up to 106– 108 /cm3).
Results from the filter artifact studies on Teflon-coated glass fiber filters show that the soot particle concentration of the aerosol affects the positive filter artifact, such that a lower particle concentration yields more hydrocarbon adsorption. This was explained by the fact that soot particles deposited on the filter occupy potential adsorption sites for hydrocarbon vapor and it was found that hydrocarbon vapor adsorbs more readily on clean filters than on sooty filters. As a consequence, backup filters serve as a reasonable estimate of the positive filter artifact only for low particle concentrations and filter soot loads. It was also shown that increasing the soot load resulted in more adsorption, which was explained by the increased effective surface area of the filter. These results indicate that filter soot load is an important parameter influencing hydrocarbon adsorption, and thus, it should be taken into consideration when performing gravimetric sampling.
The experimental setup presented in this article was proven to be a unique tool for quantitative studies of the filter artifact, as it provides a means of producing a stable and well-defined test aerosol with adjustable composition.
uast_a_689118_sup_25901175.zip
Download Zip (597.7 KB)Acknowledgments
The first two authors contributed equally to this work.
This work was supported by European Metrology Research Programme (EMRP) jointly funded by the EMRP participating countries within EURAMET and the European Union and by the project “MESTAN—Traceable measurement of nanoparticles” funded by Tekes—the Finnish Funding Agency for Technology and Innovation. We are also grateful for the support from Dekati Ltd., Ecocat Oy, and Gasmet Technologies Oy.
[Supplementary materials are available for this article. Go to the publisher's online edition of Aerosol Science and Technology to view the free supplementary files.]
REFERENCES
- Chase , R. , Duszkiewicz , G. , Richert , J. , Lewis , D. , Maricq , M. and Xu , N. 2004 . PM Measurement Artifact: Organic Vapor Deposition on Different Filter Media , Troy , MI : SAE International . SAE Technical Paper Series 2004-01-0967
- Chow , J. C. , Watson , J. G. , Chen , L. -W. A. , Rice , J. and Frank , N. H. 2010 . Quantification of PM2.5 Organic Carbon Sampling Artifacts in US Networks . Atmos. Chem. Phys. , 10 : 5223 – 5239 .
- Cui , W. , Eatough , D. J. and Eatough , N. L. 1998 . Fine Particulate Organic Material in the Los Angeles Basin—I: Assessment of the High-Volume Brigham Young University Organic Sampling System, BIG BOSS . J. Air Waste Manag. Assoc. , 48 : 1024 – 1037 .
- European Commission . 2001 . CAFE (Clean Air for Europe) programme , Brussels , Belgium : European Commission .
- Hart , K. M. and Pankow , J. F. 1994 . High-Volume Air Sampler for Particle and Gas Sampling. 2. Use of Backup Filters To Correct for the Adsorption of Gas-Phase Polycyclic Aromatic Hydrocarbons to the Front Filter . Environ. Sci. Technol. , 28 : 655 – 661 .
- ISO . 2002 . 16183:2002 Heavy Duty Engines—Measurement of Gaseous Emissions from Raw Exhaust Gas and of Particulate Emissions Using Partial Flow Dilution Systems Under Transient Test Conditions , Geneva : International Organization for Standardization (ISO) .
- Kamens , R. M. and Coe , D. L. 1997 . A Large Gas-Phase Stripping Device to Investigate Rate of PAH Evaporation from Airborne Diesel Soot Particles . Environ. Sci. Technol. , 31 : 1830 – 1833 .
- Khalek , I. 2005 . 2007 Diesel Particulate Measurement Research, Final Report CRC Project E-66-Phase 1 , San Antonio , TX : Southwest Research Institute . (2005; 03.10415)
- Kittelson , D. B. 1998 . Engines and Nanoparticles: A Review . J. Aerosol. Sci. , 29 : 575 – 588 .
- Lähde , T. , Rönkkö , T. , Happonen , M. , Söderström , C. , Virtanen , A. Solla , A. 2011 . Effect of Fuel Injection Pressure on a Heavy-Duty Diesel Engine Nonvolatile Particle Emission . Environ. Sci. Technol. , 45 : 2504 – 2509 .
- Lane , D. A. , Johnson , N. D. , Barton , S. C. , Thomas , G. H. S. and Schroeder , W. H. 1998 . Development and Evaluation of a Novel Gas and Particle Sampler for Semivolatile Chlorinated Organic Compounds in Ambient Air . Environ. Sci. Technol. , 22 : 941 – 947 .
- Lassila , A. , Kari , M. , Koivula , H. , Koivula , U. , Kortström , J. Leinonen , E. 2011 . Design and Performance of an Advanced Metrology Building for MIKES . Measurement , 44 : 399 – 425 .
- Lipsky , E. M. and Robinson , A. L. 2006 . Effects of Dilution on Fine Particle Mass and Partitioning of Semivolatile Organics in Diesel Exhaust and Wood Smoke . Environ. Sci. Technol. , 40 : 155 – 162 .
- Mader , B. T. , Flagan , R. C. and Seinfeld , J. H. 2001 . Sampling Atmospheric Carbonaceous Aerosols Using a Particle Trap Impactor/Denuder Sampler . Environ. Sci. Technol. , 35 : 4857 – 4867 .
- Mader , B. T. and Pankow , J. F. 2001 . Gas/Solid Partitioning of Semivolatile Organic Compounds (SOCs) to Air Filters. 3. An Analysis of Gas Adsorption Artifacts in Measurements of Atmospheric SOCs and Organic Carbon (OC). When Using Teflon Membrane Filters and Quartz Fiber Filters . Environ. Sci. Technol. , 35 : 3422 – 3432 .
- McDow , S. R. and Huntzicker , J. J. 1990 . Vapor Adsorption Artifact in the Sampling of Organic Aerosol: Face Velocity Effects . Atmos. Environ. , 24A : 2563 – 2571 .
- Sakurai , H. , Tobias , H. J. , Park , K. , Zarling , D. , Docherty , K. S. Kittelson , D. B. 2003 . On-line Measurements of Diesel Nanoparticle Composition and Volatility . Atmos. Environ. , 37 : 1199 – 1210 .
- Schauer , J. J. , Kleeman , M. J. , Cass , G. R. and Simoneit , B. R. T. 1999 . Measurement of Emissions from Air Pollution Sources. 2. C1 Through C30 Organic Compounds from Medium Duty Diesel Trucks . Environ. Sci. Technol. , 33 : 1578 – 1587 .
- Schlatter , J. 2002 . Application of CAST for Comparison of Instruments . Proceedings of the Sixth ETH Conference on Nanoparticle Measurement, 19–21 August, Zurich, Switzerland
- Sydbom , A. , Blomberg , A. , Parnia , S. , Stenfors , N. , Sandström , T. and Dahlén , S. E. 2001 . Health Effects of Diesel Exhaust Emissions . Eur. Respir. J. , 17 : 733 – 746 .
- Turpin , B. J. , Huntzicker , J. J. and Hering , S. V. 1994 . Investigation of Organic Aerosol Sampling Artifacts in the Los Angeles Basin . Atmos. Environ. , 28 : 3061 – 3071 .
- Turpin , B. J. , Saxena , P. and Andrews , E. 2000 . Measuring and Simulating Particulate Organics in the Atmosphere: Problems and Prospects . Atmos. Environ. , 34 : 2983 – 3013 .
- Utell , M. J. and Frampton , M. W. 2000 . Acute Health Effects of Ambient Air Pollution: The Ultrafine Particle Hypothesis . J. Aerosol. Med. , 13 : 355 – 359 .
- Vaaraslahti , K. , Virtanen , A. , Ristimäki , J. and Keskinen , J. 2004 . Nucleation Mode Formation in Heavy-Duty Diesel Exhaust with and Without a Particulate Filter . Environ. Sci. Technol. , 38 : 4884 – 4890 .
- Vecchi , R. , Valli , G. , Fermo , P. , D’Alessandro , A. , Piazzalunga , A. and Bernardoni , V. 2009 . Organic and Inorganic Sampling Artefacts Assessment . Atmos. Environ. , 43 : 1713 – 1720 .
- Viana , M. , Chi , X. , Maenhaut , W. , Cafmeyer , J. , Querol , X. Alastuey , A. 2006 . Influence of Sampling Artefacts on Measured PM, OC, and EC Levels in Carbonaceous Aerosols in an Urban Area . Aerosol Sci. Technol. , 40 : 107 – 117 .
- Virtanen , A. , Ristimäki , J. , Vaaraslahti , K. and Keskinen , J. 2004 . Effect of Engine Load on Diesel Soot Particles . Environ. Sci. Technol. , 38 : 2551 – 2556 .
- Vouitsis , E. , Ntziachristos , L. and Samaras , Z. 2003 . Particulate Matter Mass Measurements for Low Emitting Diesel Powered Vehicles: What's Next? . Prog. Energ. Combust. , 29 : 635 – 672 .