Abstract
Hourly concentrations of heavy metals in PM10 samples were continuously measured using Laser Induced Breakdown Spectroscopy (LIBS) to determine the metal distribution among Asian Dust (AD) events, local pollution events, and nonevents. Quantification of metals was performed by establishing a calibration line between 24 h average data determined by the ICP-MS after filter sampling and LIBS intensity data. It was found that in AD and local pollution events, significant anthropogenic heavy metals, such as Pb, Cr, Ni, and Zn, were detected compared to a nonevent, and that crustal elements (e.g., Al, Ca, Mg) were more abundant in the AD events than those in a local pollution event or nonevent. The AD events were further classified into “nonpolluted AD” and “polluted AD” events, depending on the air mass transport pathways. During “polluted AD” events where the air mass passed over industrialized zones, both crustal (Al, Ca, Mg) and anthropogenic (Cr, Ni, Zn) metal elements simultaneously increased with time, suggesting that the AD particles could not only include crustal elements but also have a significant quantity of anthropogenic heavy metals. The concentration of anthropogenic heavy metals (Cr + Pb + Zn) was the highest in the AD3 event in order of AD3 (polluted) > AD1 (polluted) > local pollution > AD2 (nonpolluted). However, the PM10-weighted value (Cr + Pb + Zn/PM10) was the highest in the local pollution event where concentrations of only anthropogenic heavy metals increased. Also, the hourly LIBS data was successfully used to discriminate metal contributions between AD events and local pollution events or among AD events by employing a chemometric method.
Copyright 2012 American Association for Aerosol Research
INTRODUCTION
Particulate matter (PM) in the ambient atmosphere of size less than 10 um or 2.5 um is known to have significant effects on the earth's radiation balance (IPCC Citation2007), cloud formation, visibility impairment, and human health (Dockery and Pope Citation1994). Heavy metals, despite being only a small fraction of the total mass in PM10, have been of special concern due to their adverse health effects (i.e., long term exposure of excessive concentrations of heavy metals is known to cause acute health effects in humans) (Pope Iii et al. Citation1991; Oberdörster Citation2000). It was reported that the metals can play a catalytic role in chemical reactions in the ambient atmosphere (Gelencser et al. Citation2003). Increased concentration of heavy metals in the atmosphere can be caused by various local sources (e.g., combustion of fossil fuels, exhaust emission from vehicles, industrial processes, forest fire, and waste incineration) (Buerki et al. Citation1989; Allen et al. Citation2001; Srivastava et al. Citation2008; Zhang et al. Citation2008) or by long-range transported aerosols from other regions (e.g., Asian Dust [AD]) (Husar et al. Citation2001; Sun et al. Citation2005; Lee et al. Citation2006). In Korea, AD particles that originate from desert areas in northwestern and central China, and in the southern part of Mongolia are frequently transported to Korea with a high speed winds from the northwest (Chun et al. Citation2001; Lee et al. Citation2004). According to previous reports, when the dust-laden air mass passes over heavily industrialized zones, it can pick up anthropogenic air pollutants (Choi et al. Citation2001; Wang et al. Citation2011). Severe desertification of the source region due to climate change and human activities, along with strong wind conditions in the desert areas has caused the frequency and intensity of the AD events in Korea to increase in recent years (Kim Citation2008; Kurosaki et al. Citation2011). When an AD event occurs, it is reported that humans more often suffer from respiratory diseases and hospital admissions due to increases in asthma (Yang et al. Citation2005; Kanatani et al. Citation2010), allergic rhinitis (Chang et al. Citation2006), conjunctivitis (Yang Citation2006), and contact dermatitis (Choi et al. Citation2010).
Typically, the AD events last from several hours to a few days (Hoffmann et al. Citation2008). In the traditional filter-based technique for analyzing airborne particles, atmospheric particles are drawn through a PM10 or PM2.5 inlet and collected on to filter for ∼24 h. Then, the collected samples are extracted using HNO3 with subsequent analysis by Inductively Coupled Plasma-Mass Spectrometry (ICP-MS) or Atomic Absorption Spectroscopy (AAS). However, due to the long sampling time (12 ∼ 24 h) and a series of pretreatments of the sample, the filter-based technique suffers from a low time resolution and sampling artifacts (evaporative and condensational artifacts) (Chow et al. Citation2008). Thus, the filter-based technique using 24 h filter sampling has limited use in determining transient behaviors of metals and in identifying the source of metallic particles during events that occur in a short time scale (∼several hours), as happens when there is rapid mixing of various metals from multiple sources (Mader and Pankow Citation2001; Snyder et al. Citation2009).
Laser induced breakdown spectroscopy (LIBS) can be used for real-time measurements of the elemental composition of aerosols (Cremers et al. Citation1985; Madhavi et al. Citation1999; Neuhauser et al. Citation1999; Cheng Citation2000; Hahn and Lunden Citation2000; Fisher et al. Citation2001; Panne et al. Citation2001; Hettinger et al. Citation2006; Mukherjee et al. Citation2006; Kuhlen et al. Citation2008; Park et al. Citation2009; Diwakar et al. Citation2012; Gallou et al. Citation2011). Cremers et al. (Citation1985) sampled particles on a filter with subsequent analysis by the LIBS. It does not require any sample preparation procedures, and measurement can be done at atmospheric pressure (i.e., no high vacuum system is required). The LIBS technique uses a powerful pulsed laser to generate a microplasma on a collected sample or from a single particle. The excited atomic, ionic, and molecular fragments within the plasma emit light with specific wavelengths that are signatures of elements in the sample. The single shot measurement can provide a broad range of emission lines, leading to the detection of multi-elements simultaneously. With proper (empirical) calibration, the intensity of the emission lines can be used to quantify the amount of each element. However, the LIBS emission lines from small particles (<200–300 nm) are weak, and experimental calibration for each element must be preformed for its accurate quantification. We have developed the LIBS to focus particles into a narrow spot on a collection substrate providing high sample mass in a small laser focusing area (Park et al. Citation2009). This provides strong LIBS emission lines to be used for the quantification of elements.
In this study, we applied the LIBS with a particle collection substrate stage that was automatically moved in a specific time interval to determine 1 h average concentration of heavy metals (e.g., Al, Ca, Fe, Cr, Pb, and Zn) in PM10. Measurements were conducted during AD events, local pollution events, and nonevents to examine differences in metal distribution and their time-resolved behavior in such events. Filter sampling with subsequent analysis by the ICP-MS was also carried out to measure concentrations of metals (24 h average) that were used to determine the relationship between LIBS emission intensity and concentration of metals (i.e., a calibration line for quantification of metals from LIBS data). Comparison of hourly concentrations of heavy metals among particle events was made by employing a chemometric method (principal component analysis [PCA]) to examine the effects of the AD source and transport pathways on heavy metal distribution in dust aerosols and to identify the difference in heavy metal distribution between AD and local pollution events.
EXPERIMENTAL SECTION
A schematic of the current LIBS system employed in this study is shown in . A particle collection substrate stage automatically movable in a specific time interval was added in the LIBS system. A pulsed Nd:YAG laser (Continuum Inc., USA) (1064 nm wavelength, 5 ns pulse width, 2 Hz) with a 90 mJ/pulse energy was focused to a sample spot on the substrate and generated a microplasma from the collected particles. In an aerosol-sampling chamber, a narrow beam of atmospheric aerosols, which can lead to concentrated samples and provide enough mass within a narrow spot for enhanced LIBS emission signal, was produced by a sheath air focusing nozzle system. Theoretical calculation suggested that particles larger than ∼100 nm would be collected on the substrate. A nylon membrane chosen for its lack of interference elements with the heavy metals of current interest was placed on the collection substrate stage, which was automatically shifted in 1 h intervals from 09:00 to 22:00 and 2 h intervals from 22:00 to 08:00 (next day). The laser beam was tightly focused onto the collected aerosol particles on the substrate using a plano convex lens (focal length = 75 mm). The laser intensity was monitored by a laser power meter (Gentec-EO, Canada). The relative standard deviation (RSD) of major emission lines from blank filter was around 4%. After each laser shot, the emitted light was measured through a fiber optic cable with a collecting lens. The broadband spectrometer (LIBS2000+, Ocean Optics Inc., USA), which is connected to the fiber optic cable, measured the emitted light spectra at wavelengths of 200–980 nm simultaneously. The broadband spectrometer is equipped with seven charge-coupled device (CCD) detectors having a slit of 10 μm and a 2048 element linear CCD array with a spectral resolution of 0.1 nm. The blank spectrum was also recorded for correction of LIBS spectra. Typically, at current atmospheric level of particle concentration, the LIBS spectrum of blank membrane without any particle spectra was appeared after 1–3 laser shots (i.e., particle spectrum was visible in the first 1–3 shots before particles were ablated away). We used the LIBS spectrum obtained at the first laser shot. A delay generator (BNC565, Berkeley Inc., USA) was used to control the delay time that decides when the spectrometer receives the emitted light after the laser fires. The gate delay time plays an important role in minimizing the continuum emission and enhancing the atomic lines of the LIBS spectra. The gate delay time and integration time in the spectrometer were optimized to 2 and 2.1 ms, respectively. The optimization process of LIBS parameters (laser energy, delay time, integration time, etc.) was explained in our previous article (Park et al. Citation2009). Optimization was based on data gathered using standard metal particles generated from metal solution using an atomizer, dried by a diffusion dryer, and then sent into the LIBS chamber. The data acquired by the spectrometer were stored on a PC and analyzed through the OOILIBS software (Ocean Optics Inc., USA) and MATLAB (Version 7.0). Hourly metal concentrations were analyzed by a chemometric method (e.g., PCA) to find differences in metal distributions among specific particle events (AD and local pollution events). The PCA is a multivariate analysis to reduce a set of original variables to a small number of principal components (PCs) that can explain the maximum amount of variance (Jolliffe Citation1986). Rather than using entire LIBS spectrum, particular peaks (i.e., Al, Ca, Fe, Mg, Co, Cr, Mn, Pb, and Zn emission lines) were selected for PCA analysis in this study. Each PC was assigned with loadings greater than 60% of the maximum coefficient in absolute value.
Atmospheric aerosol measurements were performed at urban Gwangju (35°13′N and 126°50′E), Korea, during the periods of October 07–October 30, 2009, March 15–March 16, 2010, and March 20–March 21, 2010. The sampling site is influenced by nearby traffic highways (western), residential heating from residential/commercial areas (southeastern), biomass burning from agricultural areas (northern), and local industrial complexes (southwestern). Atmospheric aerosols were first dried out using a diffusion dryer after passing through a PM10 inlet placed on the rooftop of the building. Then, the aerosols were sent into the LIBS sampling chamber. Also, atmospheric aerosols were sampled with a Mini-volume sampler (AirMetrics, USA) for 24 h. We followed Standard Operating Procedure in the EPA Work Assignment (US EPA Citation1999; Park and Dam Citation2008) for determination of metal concentrations from the filter sample. Hourly PM10, NO2, SO2, CO, O3, and meteorological data, such as RH and wind direction/speed, were obtained from nearby local meteorological station (∼4.5 km away from the LIBS sampling site) in Gwangju, Korea (http://www.airkorea.or.kr). A backward air trajectory analysis to find the air mass pathway during AD events at a current sampling site at an altitude of 100, 500, and 1000 m above ground level was done by using the HYSPLIT (Hybrid Single-Particle Lagrangian-Integrated Trajectory) model from the National Oceanic and Atmospheric Administration (NOAA) (http://www.arl.noaa.gov/ready/hysplit 4.html) (Draxler and Hess Citation1998).
RESULTS AND DISCUSSION
Particle events where the PM10 concentration increased by more than a factor of 2 compared to a nonevent were reported. The nonevent was defined when the 24 h average PM10 concentration was comparable to annual PM10 standard in Korea (i.e., 50 μg/m3). During the current sampling period, three AD events and two local pollution events occurred. Previous studies reported that the visual existence of dust particles with high PM10 defined an AD event, while in the local pollution event, PM10 or PM2.5 and gaseous pollutants (SO2, CO, and NOx) often increased simultaneously with a stagnant condition (low wind speed) (Lin et al. Citation2007; Tan et al. Citation2009; Kim et al. Citation2010). In this study, we compared hourly PM10, SO2, CO, NO2, O3, RH, and wind speed among particle events, and found that PM10, SO2, and wind speed among above parameters provided the most clear discrimination among the AD event, local pollution event, and nonevent, as shown in . The AD events were characterized by high PM10 and high wind speed (∼4.1 m/s) with low SO2 levels (<4 ppb), while in the local pollution event a high SO2 (7 ∼ 9 ppb) concentration with a low wind speed (∼1.6 m/s) that leads to stagnant atmospheric conditions with accumulation of air pollutants was observed (Sun et al. Citation2006). During nonevents, both PM10 and SO2 levels were lower than those in local pollution event. Average PM10, SO2, CO, NO2, O3, RH, and wind speed in each event period are summarized in .
TABLE 1 Average PM10, SO2, CO, NO2, O3, and wind speed in each event period
FIG. 2 Comparison of hourly PM10, SO2, and wind speed in Gwangju, Korea among AD events, local pollution events, and nonevents. (Color figure available online.)
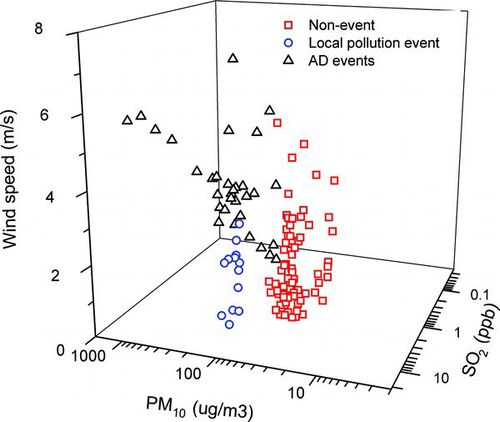
The AD events can be classified into weak, regular, and strong according to the level of PM10 (MOE Citation2004). The AD1 event (weak AD) occurred at 10:00 on October 19, 2009 and lasted only hours with increased hourly PM10 mass concentration upto 171 μg/m3. The AD2 event (regular AD) was observed on March 15–March 16, 2010 for 12 h and hourly PM10 mass concentration increased up to 416 μg/m3. The AD3 event (strong AD) occurred on March 20 to March–21, 2010 and lasted 11 h with hourly PM10 concentrations up to 1860 μg/m3. In the AD1 event, the lower levels of SO2, NO2, and CO concentrations compared to the local pollution event in the same month probably occurred due to the front air mass with a high wind speed sweeping local pollutants away (cleaning effect) (Guo et al. Citation2004). During the AD3 event, SO2, NO2, and CO values were relatively high compared to those in AD1 and AD2 events as shown in probably because the locally polluted air mass was mixed with the AD air mass. This will be discussed more in a later section. During all AD events, the RH was typically lower than that in local pollution events and nonevents, suggesting that dry atmospheric conditions favor the occurrence of AD events. A northwestern wind with a high speed (>3 m/s) was also dominant during the AD events, providing a higher probability of long-range transport of dust particles suspended from the desert areas making their way to Korea. Previous studies reported that a high surface wind speed is an important factor for uplifting and carrying of enormous dust and sand particles into the air followed by long-range transport (Duce et al. Citation1980; Husar et al. Citation2001).
FIG. 3 A backward air trajectory ending at an altitude of 100, 500, and 1000 m above ground level at the sampling point during (a) AD1, (b) AD2, and (c) AD3 events (the shaded area indicates industrial zones). (Color figure available online.)
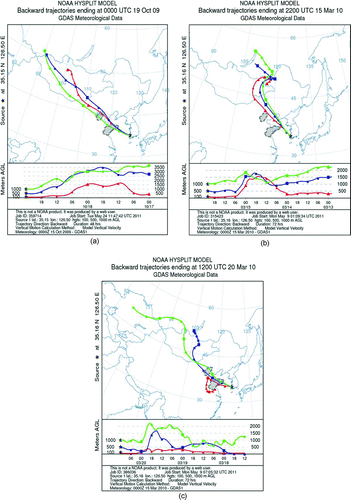
FIG. 4 Comparison of LIBS emission spectra of particles observed (a) between AD1 event and nonevent and (b) between local pollution event and non-event. (Color figure available online.)
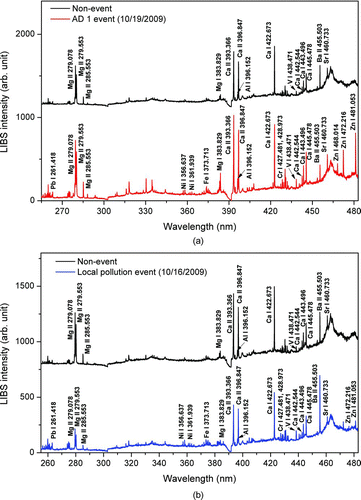
In order to identify the origin and pathways of the AD particles, a backward air trajectory analysis was performed at an altitude of 100, 500, and 1000 m above ground level at the sampling point during AD events by using the NOAA HYSPLIT 4 model. As shown in , the air mass originated from Inner Mongolia and Gobi desert areas in current AD events, but their transport pathway varied among AD events. Depending on the transport pathway, the AD events were classified into “polluted AD” and “nonpolluted AD” event. During a “nonpolluted AD” (AD2 belongs to this) event, air masses arrived directly at our sampling point without passing over any industrial areas (). However, during a “polluted AD” (AD1 and AD3) event, the air masses arrived at the sampling site after passing over industrial zones in China as shown in and c. Previous studies reported that significant anthropogenic air pollutants including heavy metals (i.e., As, Cd, Ni, Zn, Cu, and Pb) could be produced from such industrial areas where various steel, brass, and battery industries (Sun et al. Citation2004; Park and Dam Citation2008) and coal combustion sources exist (Hu et al. Citation2010). The effect of the transport pathway on metal distribution in the AD particles will be examined in the next section.
TABLE 2 Average metal concentrations of Al, Ca, Fe, Cr, Pb, and Zn during AD events, local pollution events, and non-events
A qualitative difference in LIBS emission spectra among an AD event (AD1), local pollution event, and nonevent is shown in . In all cases, Ca (393.366, 396.847, 422.673, 443.496, and 445.478 nm), Fe (238.204, 259.837, 274.932, and 373.713 nm), Pb (261.418 nm), Mg (279.553 and 280.271 nm), Ba (455.503 nm), V (438.471 nm), Sr (460.733 nm), Al (396.152 nm), and K (766.49 nm) emission lines were detected although their intensity differed among events. In the AD (AD1, AD2, and AD3) and local pollution event, emission lines of heavy metals such as Cr (427.481 and 428.973 nm), Ni (356.637 and 361.939 nm), and Zn (468.014, 472.216, and 481.053 nm) were newly detected compared to the nonevent. Typically, emission lines of crustal elements (e.g., Al, Ca, and Mg) in the AD event were much stronger than those in the local pollution event or nonevent, while in the local pollution event, we observed the stronger emission lines of anthropogenic heavy metals (e.g., Pb, Ni, Cr, and Zn) compared to those in the nonevent.
To determine concentrations of metals, the relationship between LIBS intensity and concentration for each metal must be determined. The emission intensity is typically normalized by a major common element (i.e., internal standardization) to minimize shot-to-shot variation in the LIBS emission signal and matrix effects (Archambault et al. Citation2005). The internal standard element is usually chosen, existing in the same spectral region of metallic elements of interest and having similar excitation energy to the analyte (Archambault et al. Citation2005). Significant carbon emission lines were observed from the blank substrate (i.e., nylon membrane filter). Since the carbon emission lines from the underlying filter were much stronger compared to those from PM, we used the carbon line for internal standard. Carbon emission line at 247.856 nm was selected as the internal standard, which led to the highest sensitivity in the calibration line for each metal as shown in . Metal concentrations in ambient PM samples (24 h average data) independently collected in the filter were determined by the ICP-MS, and were used to obtain the calibration lines (i.e., the relationship between LIBS emission intensity and concentration of metals) as shown in . A good linear correlation between the LIBS intensity ratio (24 h average data) and concentration for each metal for the given concentration range was observed with a correlation coefficient (r) ranging from 0.87 to 0.99 as shown in . The slope and y-intercept of the calibration lines varied for each metal. In the strong AD3 events, the LIBS emission lines for Ca, Fe, and Al were saturated possibly due to self-absorption as not shown here. For this case, we used extrapolation of the linear curve, possibly leading to errors in determination of such metal concentration determined by the LIBS at the high concentration range. The LIBS intensity was known to be associated with various thermal properties (i.e., melting/boiling point and specific heat) and physical parameters (1st ionization energy, reflectivity, and energy level) (Geertsen et al. Citation1994; Mukherjee and Cheng Citation2008). We observed that lower values of boiling points and upper energy levels led to higher sensitivity (i.e., higher slope of the calibration line). Note that all LIBS experimental conditions were identical in the above measurements. When the LIBS intensity ratio was normalized to boiling point (Tb ) and upper energy level (ΔE) of each metal in a similar concentration range (0.02 ∼ 1 μg/m3) (i.e., the LIBS intensity of a certain element was multiplied by the ratio of Tb of the element to Tb of element having the highest value and the ratio of ΔE of the element to ΔE of element having the highest value among tested elements), a single universal line (r = 0.83) was established regardless of the metals, as shown in . A further study on this issue is required. In this study, we used the calibration line (i.e., data in ) for each metal for quantification of its hourly concentration.
FIG. 5 (a) A relationship between LIBS intensity ratios (Al/C, Ca/C, Fe/C, Cr/C, Pb/C, and Zn/C) and concentrations and (b) a relationship between LIBS intensity ratios normalized by boiling point (Tb ) and upper energy level (ΔE), and concentrations.
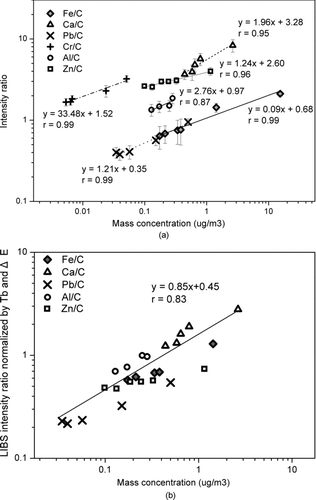
FIG. 6 Average concentration of (Cr + Pb + Zn) and PM10-weighted value [(Cr + Pb + Zn)/PM10] during AD and local pollution events. (Color figure available online.)
![FIG. 6 Average concentration of (Cr + Pb + Zn) and PM10-weighted value [(Cr + Pb + Zn)/PM10] during AD and local pollution events. (Color figure available online.)](/cms/asset/e3920cd9-f885-4059-8269-86fd5e422b41/uast_a_692492_o_f0006g.jpg)
FIG. 7 Temporal variations of the concentrations of crustal elements and anthropogenic heavy metals during (a) AD2 event and (b) local pollution event on October 13, 2009. (Color figure available online.)
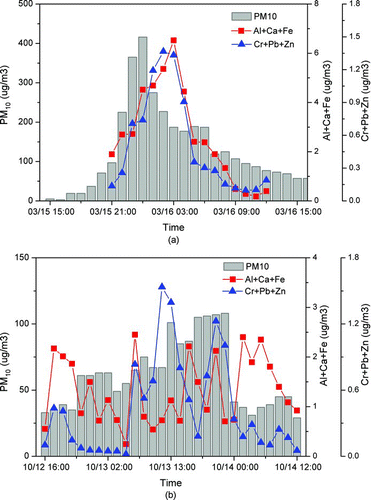
FIG. 8 Score plots of (a) PC1 and PC2 during AD and local pollution events and (b) PC1, PC2, and PC3 among AD events (AD1, AD2, and AD3).
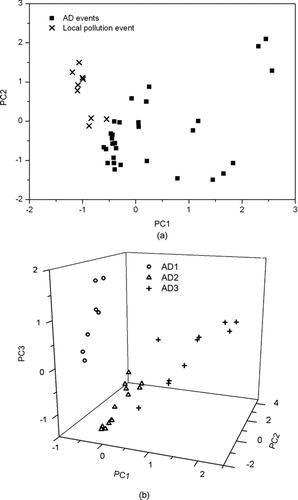
Average concentrations of Al, Ca, Fe, Cr, Pb, and Zn determined by the LIBS during the whole period of events are summarized in . During AD events, concentrations of crustal elements (Al, Ca, and Fe) in PM10 significantly increased by a factor of 2–13 compared to those in a nonevent, indicating that the dust particles consisting of crustal elements significantly contributed to PM10. Concentrations of anthropogenic heavy metals (Cr, Pb, and Zn) also increased, especially in the AD1 and AD3 events that were considered “polluted AD.” This suggests that the AD particles could not only include crustal elements, but also have a significant quantity of anthropogenic metals, depending on their transport pathway. Since the Cr, Zn, and Pb were seldom observed in original dust particles collected in the source region (Choi et al. Citation2001; Kim et al Citation2006), they can be used as tracer for contaminated dust particles. In the local pollution event period, little increase of crustal elements was observed while the concentrations of anthropogenic heavy metals (Cr, Pb, and Zn) increased significantly. A sum of anthropogenic heavy metals (Cr + Pb + Zn) and its PM10-weighted value ((Cr + Pb + Zn)/PM10) during AD and local pollution events are compared in . The concentration of anthropogenic heavy metals (Cr + Pb + Zn) was the highest in the AD3 event in the order of AD3 (polluted) > AD1 (polluted) > local pollution > AD2 (nonpolluted). This occurred probably because the dust-laden air mass encountered polluted air mass from heavily industrial area leading to different mixing components in the dust particles from original dust particles mainly consisting of mineral components (). The PM10-weighted value was the highest in the local pollution event. Due to a significant increase of crustal elements in addition to anthropogenic heavy metals in PM10 during AD events, the fraction of the anthropogenic heavy metals in PM10 was relatively smaller than that in the local pollution event. The fraction of the anthropogenic heavy metals was also found to be the lowest in the AD2 (nonpolluted) event.
Temporal variations of hourly anthropogenic heavy metals (Cr + Pb + Zn) and crustal elements (Al, Ca, and Fe) during AD and local pollution events were investigated, as shown in and b. During AD events, hourly concentrations of both crustal and anthropogenic metals, and PM10 simultaneously increased with time, while during the local pollution event (), there was no such behavior. During AD events, PM10 concentrations showed a good correlation with crustal elements (r = 0.62–0.81) with the highest correlation (r = 0.81) in the AD2 event, indicating that in the AD2 event (“nonpolluted AD”) crustal elements from dust particles contributed significantly to the PM10. No significant correlation among crustal elements, anthropogenic metals, and PM10 in the local pollution event suggests that the crustal elements exist as a small fraction of PM10, and various local sources contributed to the increase of such elements, leading to a lack of correlation among them. In a certain local pollution event, it was reported that the PM was correlated well with crustal elements (i.e., Ca) (Hettinger et al. Citation2006).
To investigate the difference in distribution of metals among AD and local pollution events in more detail, hourly metal emission lines (Al, Ca, Fe, Mg, Co, Cr, Mn, Pb, and Zn) were analyzed using the chemometric method (i.e., PCA). Three PCs (PC1, PC2, and PC3) with eigenvalues greater than unity accounted for 82.7% of the total variance. Accounting for 40.8% of the total variance, Al, Ca, Mg, and Co, which are known to have a crustal origin (Almeida et al. Citation2005; Zhang et al. Citation2010), had high values for PC1. Accounting for 24.1% of the total variance, Fe, Mn, and Pb were assigned to PC2, which usually originate from metal operation from industries (Polissar et al. Citation2001; Watson and Chow Citation2001). Accounting for 17.8% of the total variance, Cr and Zn were grouped into PC3, which is related to exhaust, industrial, and municipal waste incineration emissions (Watson and Chow Citation2001; Tanner et al. Citation2008). presents scores plot of the PCs determined by using emission lines of such metals. presents score plots of PC1 and PC2 between AD and local pollution events. A clear difference in heavy metal distribution between AD and local pollution events was found. In AD events, the PC1 (crustal elements) is relatively high, and varying PC2 was observed, while in the local pollution event, only PC1 was low. Also, we found that metal distribution differed depending on the type of AD events (i.e., polluted AD [AD1 and AD3] versus nonpolluted AD [AD2]), as shown in . In a “nonpolluted AD,” the PC3 were relatively low compared to a “polluted AD,” which was consistent with low anthropogenic heavy metal concentration in a “nonpolluted AD.” Our data suggest that hourly metal emission lines determined by the LIBS were useful to discriminate AD events from local pollution events and to classify AD events having different origins and pathways.
CONCLUSIONS
We successfully applied the LIBS technique to determine 1 h average concentration of heavy metals in PM10. Measurements were conducted during AD events, local pollution events, and nonevents, and time-resolved behaviors of crustal (Al, Ca, and Mg) and anthropogenic (Cr, Ni, and Zn) metal elements, and differences in metal distribution were investigated. During “polluted AD” events where the air mass passed over industrialized zones, both crustal (Al, Ca, and Mg) and anthropogenic (Cr, Ni, and Zn) metal elements simultaneously increased with time, and the concentration of anthropogenic heavy metals (Cr + Pb + Zn) was the highest in the AD3 event in the order of AD3 (polluted) > AD1 (polluted) > local pollution > AD2 (nonpolluted), suggesting that the AD particles could not only include crustal elements, but also have a significant quantity of anthropogenic heavy metals depending on their transport pathways. Also, the hourly LIBS data with a chemometric method were applied to discriminate metal contributions between AD events and local pollution events or among AD events, providing useful insights into effects of sources and transport pathways of dust particles on their heavy metal distribution. Currently, we are developing the LIBS system without a collection substrate, enabling to detect a single particle in air by employing a triggering laser system. The LIBS technique could be a promising tool for challenging issues associated with elemental analysis of atmospheric aerosols in a rapid manner.
Acknowledgments
The research described in this article was supported by the National Research Foundation of Korea grant funded by the Korea government (MEST) (No. 2011-0015548) and by Korea Ministry of Environment as “The GAIA Project” (No. G111-17002-0002-0).
REFERENCES
- Allen , A. G. , Nemitz , E. , Shi , J. P. , Harrison , R. M. and Greenwood , J. C. 2001 . Size Distributions of Trace Metals in Atmospheric Aerosols in the United Kingdom . Atmos. Environ. , 35 : 4581 – 4591 .
- Almeida , S. M. , Pio , C. A. , Freitas , M. C. , Reis , M. A. and Trancoso , M. A. 2005 . Source Apportionment of Fine and Coarse Particulate Matter in a Sub-Urban Area at the Western European Coast . Atmos. Environ. , 39 : 3127 – 3138 .
- Archambault , J. F. , Vintiloìu , A. and Kwong , E. 2005 . The Effects of Physical Parameters on Laser-Induced Breakdown Spectroscopy Analysis of Intact Tablets . AAPS Pharm. Sci. Tech. , 6 ( 2 ) : E253 – E261 .
- Buerki , P. R. , Gaelli , B. C. and Nyffeler , U. P. 1989 . Size-Resolved Trace Metal Characterization of Aerosols Emitted by Four Important Source Types in Switzerland . Atmos. Environ. , 23 ( 8 ) : 1659 – 1668 .
- Chang , C. C. , Lee , I. M. , Tsai , S. S. and Yang , C. Y. 2006 . Correlation of Asian Dust Storm Events with Daily Clinic Visits for Allergic Rhinitis in Taipei, Taiwan . J. Toxicol. Environ. A , 69 : 229 – 235 .
- Cheng , M. D. 2000 . Real-Time Measurement of Trace Metals on Fine Particles by Laser-Induced Plasma Techniques . Fuel process. Technol. , 65 : 219 – 229 .
- Choi , H. , Shin , D. W. , Kim , W. , Doh , S. J. , Lee , S. H. and Noh , M. 2010 . Asian Dust Storm Particles Induce a Broad Toxicological Transcriptional Program in Human Epidermal Keratinocytes . Toxicol. Lett. , 200 : 92 – 99 .
- Choi , J. C. , Lee , M. , Chun , Y. , Kim , J. and Oh , S. 2001 . Chemical Composition and Source Signature of Spring Aerosol in Seoul, Korea . J. Geophys. Res. , 106 ( D16 ) : 18067 – 18074 .
- Chow , J. C. , Watson , J. G. , Lowenthal , D. H. , Park , K. , Doraiswamy , P. Bowers , K. 2008 . Continuous and Filter-Based Measurements of PM2.5 Nitrate and Sulfate at the Fresno Supersite . Environ. Monit. Assess. , 144 : 179 – 189 .
- Chun , Y. , Boo , K. O. , Kim , J. , Park , S. U. and Lee , M. 2001 . Synopsis, transport, and physical characteristics of Asian dust in Korea . J. Geophys. Res. , 106 ( D16 ) : 18,461 – 18,469 .
- Cremers , D. A. and Radziemski , L. J. 1985 . Direct Detection of Beryllium on Filters Using the Laser Spark . Appl. Spectrosc. , 39 : 57 – 63 .
- Diwakar , P. , Kulkarni , P. and Birch , M. E. 2012 . New Approach for Near-Real-Time Measurement of Elemental Composition of Aerosol Using Laser-Induced Breakdown Spectroscopy . Aerosol Sci. Technol. , 46 : 316 – 332 .
- Dockery , D. W. and Pope , C. A. 1994 . Acute Respiratory Effects of Particulate Air Pollution . Ann. Rev. Public Health , 15 : 107 – 132 .
- Draxler , R. R. and Hess , G. D. 1998 . An Overview of the HYSPLIT_4 Modelling System for Trajectories, Dispersion and Deposition . Aust. Meteorol. Mag. , 47 : 295 – 308 .
- Duce , R. A. , Unni , C. K. , Ray , B. J. , Prospero , J. M. and Merrill , J. T. 1980 . Long-Range Atmospheric Transport of Soil Dust from Asia to the Tropical North Pacific: Temporal Variability . Science , 209 : 1522 – 1524 .
- Fisher , B. T. , Johnsen , H. A. , Buckley , S. G. and Hahn , D. W. 2001 . Temporal Gating for the Optimization of Laser-Induced Breakdown Spectroscopy Detection and Analysis of Toxic Metals . Appl. Spectrosc. , 55 : 1312 – 1319 .
- Gallou , G. , Sirven , J. B. , Dutouquet , C. , Bihan , O. L. and Frejafon , E. 2011 . Aerosols Analysis by LIBS for Monitoring of Air Pollution by Industrial Sources . Aerosol Sci. Technol. , 45 ( 8 ) : 918 – 926 .
- Geertsen , C. , Briand , A. , Chartier , F. , Lacour , J. L. , Mauchien , P. Sjostrom , S. 1994 . Comparison Between Infrared and Ultraviolet Laser Ablation at Atmospheric Pressure-Implications for Solid Sampling Inductively Coupled Plasma Spectrometry . J. Anal. Atom. Spectrom. , 9 : 17 – 22 .
- Gelencser , A. , Hoffer , A. , Kiss , G. , Tombacz , E. , Kurdi , R. and Bencze , L. 2003 . In-Situ Formation of Light-Absorbing Organic Matter in Cloud Water . J. Atmos. Chem. , 45 : 25 – 33 .
- Guo , J. , Rahn , K. A. and Zhuang , G. 2004 . A Mechanism for the Increase of Pollution Elements in Dust Storms in Beijing . Atmos. Environ. , 38 : 855 – 862 .
- Hahn , D. W. and Lunden , M. M. 2000 . Detection and Analysis of Aerosol Particles by Laser-Induced Breakdown Spectroscopy . Aerosol Sci. Technol. , 33 : 30 – 48 .
- Hettinger , B. , Hohreiter , V. , Swingle , M. and Hahn , D. W. 2006 . Laser-Induced Breakdown Spectroscopy for Ambient Air Particulate Monitoring: Correlation of Total and Speciated Aerosol Particle Counts . Appl. Spectrosc. , 60 : 237 – 245 .
- Hoffmann , C. , Funk , R. , Sommer , M. and Li , Y. 2008 . Temporal Variations in PM10 and Particle Size Distribution During Asian Dust Storms in Inner Mongolia . Atmos. Environ. , 42 : 8422 – 8431 .
- Hu , M. , Guo , S. , Yue , D. , Hu , W. , Zhu , T. Zhang , Y. 2010 . Particle pollution in Beijing: Features, Source and Secondary Formation . Geophys. Res. Abstr. , 12 : 8356
- Husar , R. B. , Tratt , D. M. , Schichtel , B. A. , Falke , S. R. , Li , F. Jaffe , D. 2001 . Asian Dust Events of April 1998 . J. Geophys. Res. , 106 ( D16 ) : 18,317 – 18,330 .
- Intergovernmental Panel on Climate Change (IPCC) . 2007 . Climate Change 2007 , New York : Cambridge Univ. Press .
- Jolliffe , I. T. 1986 . Principal Component Analysis , New York : Springer-Verlag .
- Kanatani , K. T. , Ito , I. , Al-Delaimy , W. K. , Adachi , Y. , Mathews , W. C. Ramsdell , J. W. 2010 . Desert Dust Exposure is Associated with Increased Risk of Asthma Hospitalization in Children . Am. J. Respir. Crit. Care Med. , 182 : 1475 – 1481 .
- Kim , J. 2008 . Transport Routes and Source Regions of Asian Dust Observed in Korea During the Past 40 Years (1965–2004) . Atmos. Environ. , 42 : 4778 – 4789 .
- Kim , K. H. , Kang , C. H. , Lee , J. H , Choi , K. C. , Youn , Y. H. and Min , H. S. 2006 . Investigation of Airborne Lead Concentrations in Relation to Asian Dust Events and Air Mass Transport Pathways . J. Aerosol Sci. , 37 : 1809 – 1825 .
- Kim , S. W. , Yoon , S. C. , Kim , J. , Kang , J. Y. and Sugimoto , N. 2010 . Asian Dust Event Observed in Seoul, Korea, During 29–31 May 2008: Analysis of Transport and Vertical Distribution of Dust Particles from Lidar and Surface Measurements . Sci. Tot. Environ. , 408 : 1707 – 1718 .
- Kuhlen , T. , Fricke-Begemann , C. , Strauss , N. and Noll , R. 2008 . Analysis of Size-Classified Fine and Ultrafine Particulate Matter on Substrates with Laser-Induced Breakdown Spectroscopy . Spectrochim. Acta B. , 63 : 1171 – 1176 .
- Kurosaki , Y. , Shinoda , M. and Mikami , M. 2011 . What Caused a Recent Increase in Dust Outbreaks Over East Asia . Geophys. Res. Lett. , 38 : L11702
- Lee , B. K. , Jun , N. Y. and Lee , H. K. 2004 . Comparison of Particulate Matter Characteristics Before, During, and After Asian Dust Events in Incheon and Ulsan, Korea . Atmos. Environ. , 38 : 1535 – 1545 .
- Lee , B. K. , Lee , H. K. and Jun , N. Y. 2006 . Analysis of Regional and Temporal Characteristics of PM10 During an Asian Dust Episode in Korea . Chemosphere , 63 : 1106 – 1115 .
- Lin , C. Y. , Wang , Z. , Chen , W. N. , Chang , S. Y. , Chou , C. C. K. Sugimoto , N. 2007 . Long-Range Transport of Asian Sust and Air Pollutants to Taiwan: Observed Evidence and Model Simulation . Atmos. Chem. Phys. , 7 : 423 – 434 .
- Mader , B. T. and Pankow , J. F. 2001 . Gas/Solid Partitioning of Semivolatile Organic Compounds (SOCs) to Air Filters. An Analysis of Gas Adsorption Artifacts in Measurements of Atmospheric SOCs and Organic Carbon (OC) when Using Teflon Membrane Filters and Quartz Fiber Filters . Environ. Sci. Technol. , 35 : 3422 – 3432 .
- Madhavi , Z. M. , Cheng , M. D. and Martin , R. C. 1999 . Aerosol Measurement by Laser-Induced Plasma Technique: A Review . Aerosol Sci. Technol. , 31 : 409 – 421 .
- Ministry of the Environment (MOE) in Republic of Korea . 2004 . Strategy for Improving the Air Quality in the Metropolitan Area . Available online at: http://www.me.go.kr/web/286/me/common/board
- Mukherjee , D. and Cheng , M. D. 2008 . Quantitative Analysis of Carbonaceous Aerosols Using Laser-Induced Breakdown Spectroscopy: A Study on Mass Loading Induced Plasma Matrix Effects . J. Anal. Atom. Spectrom. , 23 : 119 – 128 .
- Mukherjee , D. , Rai , A. and Zachariah , M. R. 2006 . Quantitative Laser-Induced Breakdown Spectroscopy for Aerosols Via Internal Calibration: Application to the Oxidative Coating of Aluminum Nanoparticles . J. Aerosol Sci. , 37 : 677 – 695 .
- Neuhauser , R. E. , Panne , U. and Niessner , R. 1999 . Laser-Induced Plasma Spectroscopy (LIPS): A Versatile Tool for Monitoring Heavy Metal Aerosols . Anal. Chim. Acta. , 392 : 47 – 54 .
- Oberdörster , G. 2000 . Toxicology of Ultrafine Particles: In Vivo Studies . Philos. Trans. R. Soc. A. , 358 : 2719 – 2740 .
- Panne , U. , Neuhauser , R. E. , Theisen , M. and Niessner , H. F. R. 2001 . Analysis of Heavy Metal Aerosols on Filters by Laser-Induced Plasma Speactroscopy . Spectrochim. Acta B. , 56 : 839 – 850 .
- Park , K. , Cho , G. and Kwak , J. H. 2009 . Development of an Aerosol Focusing-Laser Induced Breakdown Spectroscopy (Aerosol Focusing-LIBS) for Determination of Fine and Ultrafine Metal Aerosols . Aerosol Sci. Technol. , 43 : 375 – 386 .
- Park , K. and Dam , H. D. 2008 . Characterization of Metal Aerosols in PM10 from Urban, Industrial, and Asian Dust Sources . Environ. Monit. Assess. , 160 : 289 – 300 .
- Polissar , A. V. , Hopke , P. K. and Poirot , R. L. 2001 . Atmospheric Aerosol Over Vermont: Chemical Composition and Sources . Environ. Sci. Technol. , 35 : 4604 – 4621 .
- Pope Iii , C. A. , Dockery , D. W. , Spengler , J. D. and Raizenne , M. E. 1991 . Respiratory Health and PM10 Pollution: A Daily Time Series Analysis . Am. Rev. Respir. Dis. , 144 : 668 – 674 .
- Snyder , D. C. , Schauer , J. J. , Gross , D. S. and Turner , J. R. 2009 . Estimating the Contribution of Point Sources to Atmospheric Metals Using Single-Particle Mass Spectrometry . Atmos. Environ. , 43 : 4033 – 4042 .
- Srivastava , A. , Gupta , S. and Jain , V. K. 2008 . Source Apportionment of Total Suspended Particulate Matter in Coarse and Fine Size Ranges Over Delhi . Aerosol Air Qual. Res. , 8 : 188 – 200 .
- Sun , Y. , Zhuang , G. , Tang , A. , Wang , Y. and An , Z. 2006 . Chemical Dharacteristics of PM2.5 and PM10 in Haze-Fog Episodes in Beijing . Environ. Sci. Technol. , 40 : 3148 – 3155 .
- Sun , Y. , Zhuang , G. , Wang , Y. , Han , L. , Guo , J. Dan , M. 2004 . The Air-Borne Particulate Pollution in Beijing - Concentration, Composition, Distribution and Sources . Atmos. Environ. , 38 : 5991 – 6004 .
- Sun , Y. , Zhuang , G. , Wang , Y. , Zhao , X. , Li , J. Wang , Z. 2005 . Chemical Composition of Dust Storms in Beijing and Implications for the Mixing of Mineral Aerosol with Pollution Aerosol on the Pathway . J. Geophys. Res. , 110 ( D24 ) : 1 – 11 .
- Tan , J. H. , Duan , J. C. , Chen , D. H. , Wang , X. H. , Guo , S. J. Bi , X. H. 2009 . Chemical Characteristics of Haze During Summer and Winter in Guangzhou . Atmos. Res. , 94 : 238 – 245 .
- Tanner , P. A. , Ma , H. L. and Yu , P. K. N. 2008 . Fingerprinting Metals in Urban Street Dust of Beijing, Shanghai, and Hong Kong . Environ. Sci. Technol. , 42 : 7111 – 7117 .
- US EPA . 1999 . Determination of Metals in Ambient Particulate Matter Using Inductively Coupled Plasma/Mass Spectrometry (ICP/MS), USEPA Report, EPA/625/R-96/101a, US Environmental Protection Agency, Cincinnati, Ohio, USA .
- Wang , Q. , Zhuang , G. , Juan , L. , Huang , K. , Zhang , R. Jiang , Y. 2011 . Mixing of Dust with Pollution on the Transport Path of Asian Dust – Revealed from the Aerosol Over Yulin, the North Edge of Loess Plateau . Sci. Tot. Environ. , 409 : 573 – 581 .
- Watson , J. G. and Chow , J. C . 2001 . Source Characterization of Major Emission Sources in the Imperial and Mexicali Valleys Along the US/Mexico Border . Sci. Tot. Environ. , 276 : 33 – 47 .
- Yang , C. Y. 2006 . Effects of Asian Dust Storm Events on Daily Clinical Visits for Conjunctivitis in Taipei, Taiwan . J. Toxicol. Environ. A , 69 : 1673 – 1680 .
- Yang , C. Y. , Tsai , S. S. , Chang , C. C. and Ho , S. C. 2005 . Effects of Asian Dust Storm Events on Daily Admissions for Asthma in Taipei, Taiwan . Inhal. Toxicol. , 17 : 817 – 821 .
- Zhang , R. J. , Shen , Z. X. , Zou , H. , Wang , W. , Han , Y. and Zhou , J. 2008 . Study of Elemental Mass Size Distributions of Aerosol in Lijiang, a Background Site in Southwest China . Aerosol Air Qual. Res. , 8 : 339 – 347 .
- Zhang , W. , Zhuang , G. , Huang , K. , Li , J. , Zhang , R. Wang , Q. 2010 . Mixing and Transformation of Asian Dust with Pollution in the Two Dust Storms Over the Northern China in 2006 . Atmos. Environ. , 44 : 3394 – 3403 .