Abstract
Particle deposition in a child's nasal cavity is much different than that in the nasal airway of an adult because of the differences in geometry and breathing patterns. However, most deposition studies have focused on adults, and only a limited number of studies have been reported in a child's nasal cavity. This study was conducted as an in vitro test and computational fluid dynamics (CFD) analysis of particle deposition in the nasal replica of a 5-year-old child; both total and regional depositions were evaluated. The geometry of the nasal replica was based on magnetic resonance images of the head of the child. The replica was made by a rapid-prototyping machine. Monodisperse oleic acid and polystyrene latex aerosols ranging in size between 1 and 20 μm were delivered into the replica at flow rates of 10 and 20 L/min. Results showed that the total deposition from the in vitro experiments and CFD predictions matched to a high degree. Good agreement was also obtained when results were compared to existing in vitro deposition data from children having comparable nasal geometries. For regional depositions, patterns between the replica and CFD data were similar in trend and magnitude for all four regions considered, although some regions deviated slightly. More tests in nasal replicas of different aged children will be carried out.
Copyright 2013 American Association for Aerosol Research
INTRODUCTION
Aerosol deposition processes in the nasal cavity are important in aerosol therapy and inhalation toxicology applications. Data on particle deposition in the nasal cavity will determine the variability of particle deposition in the lung by nasal breathing. The direct nose-to-brain drug delivery, which can bypass the blood–brain barrier, is also a noninvasive and convenient method of targeting the central nervous system with a minimum of systemic exposure (Charlton et al. Citation2007; Dhuria et al. Citation2010). Many deposition studies have focused on adult nasal airways (Cheng Citation2003; Garcia et al. Citation2009). However, the efficiency of the nose for filtering particles is dependent on the anatomy of the nasal passage and breathing conditions, which change with age. Compared to adults, children have more opportunities for exposure to ambient toxicants because they spend more time outdoors and breathe faster. Because their defense mechanism is not mature, children are more susceptible to toxicants than adults (Dieffenbach et al. Citation2009).
Few in vivo studies on particle deposition in a child's nasal airways have been carried out. Becquemin et al. (Citation1991) investigated nasal particle deposition and pressure drop in 20 children ages 5 to 15 years. Their results indicated that nasal particle deposition was lower in children than in adults for breathing rates of rest and light exercise. The nasal deposition was a function of particle size, ventilation rate, and nasal resistance. The study of Bennett et al. (Citation2008) drew the same conclusion. However, these two studies included only a few monodisperse particle sizes (1−2.8 μm) without any relation to nasal geometry.
Using an airway replica to investigate nasal deposition provides the advantage of avoiding the use of human subjects. Before 2000, the technique of making nasal cast was limited; many researchers only focused on nasal studies in adults. Only a few nasal casts of children were developed and used for deposition studies (Swift Citation1991; Cheng et al. Citation1995). With the development of new manufacturing technologies, especially three dimensional (3-D) printing, preparing nasal replicas has become much easier and less costly. More in vitro studies have focused on nasal deposition in children (Janssens et al. Citation2001; Storey-Bishoff et al. Citation2008; Golshahi et al. Citation2011). Swift (Citation1991) compared nasal deposition in adult and infant replicas for particle sizes from 1 to 10 μm. Cheng et al. (Citation1995) evaluated deposition of ultrafine particles in nasal replicas from young children. Both studies indicated a higher nasal deposition in children than in adults at the same breathing rates. Swift (Citation1991) suggested similar deposition in infant and adult at rest, whereas at the same flow rate, deposition in the infant was higher.
TABLE 1 5-year-old boy nasal cavity dimension
Nasal replicas have recently been developed in infants and children from 3 months to 14 years on the basis of computer tomographic scans at the University of Alberta (Storey-Bishoff et al. Citation2008; Golshahi et al. Citation2010, Citation2011). The replicas were made of acrylic plastic. Ultrafine and microsized aerosols were tested in these replicas for deposition patterns. Empirical equations obtained by analyzing many experimental data indicated that the deposition efficiency was a function of Stokes number (Stk) and Reynolds number (Re). Since Stk and Re include nasal geometry and inhalation, the empirical equations can represent children of all ages. However, the definition of characteristic diameter (dc) of the geometry in the Stk can make different results. We will discuss the details in this study. During the same period, we also developed a nasal replica on the basis of magnetic resonance imaging (MRI) scan of a 5-year-old and devised a numerical calculation for aerosol deposition in the replica (Xi et al. Citation2011). Since no regional nasal deposition data are available for children, our replica was separated into four regions to get more detailed deposition information. This article presents the experimental portion of our study to evaluate total and regional particle deposition of monodisperse aerosols in a child's nasal replica.
EXPERIMENTAL METHOD
Nasal Replica
An anatomically realistic nasal airway replica was constructed on the basis of MRI tracing data from Materialise Inc. (Ann Arbor, MI, USA) by Xi et al. (Citation2011) at the University of Arkansas. The MRI scan tracings contained 128 coronal cross sections 1.5 mm apart from the nostril to the upper trachea. These tracings were segmented in Mimics (Materialise Inc., Ann Arbor, MI, USA) according to the contrast between the osseous structures and intranasal air to convert the raw image data into a 3-D model, a set of cross-sectional contours that define the nasal airway. The internal nasal geometry was constructed on the basis of these contours. The physical replica was made using a rapid-prototyping machine (Dimension 1200es, Eden Prairie, MN, USA) with an acrylonitrile butadiene styrene material. To determine the regional deposition, the nasal replica was separated into four units as shown in : anterior (#1), turbinate (#2), posterior (#3), and laryngeal and tracheal (#4) regions. The parameters of the replica are listed in . The pressure drop versus the flow rate was measured by connecting a VELOCICALC (Model 8360-M; TSI Inc., St. Paul, MN, USA) at the outlet of the replica. This replica was the complete nasal airway region from the nostril to the larynx, similar to the replicas studied by Golshahi et al. (Citation2011); some human replicas such as those used by Swift (Citation1991), Cheng et al. (Citation2001), and Garcia et al. (Citation2009) did not include the laryngeal region. Because the volume and surface area of our replica is close to the subjects 6, 7, and 10 of Golshahi et al. (Citation2011), our deposition data were also compared to these three subjects.
Aerosol Generation and Size Measurement
A vibrating orifice monodisperse aerosol generator (VOAG, Model 3050, TSI Inc., St. Paul, MN, USA) generated the monodisperse sodium-fluorescein-tagged oleic acid aerosols ranging from 2 to 20 μm in size for this study. In terms of aerosols <2 μm, the polystyrene latex (PSL) fluorescent aerosols (Duke Scientific, Palo Alto, CA, USA) were generated from a medical nebulizer (Hospital, Lindenhurst, NY, USA). The experimental setup was similar to our previous study for oral replica deposition (Zhou et al. Citation2011), Detailed description of the experimental setup was included as online supplemental information. The sample flow rates were set at 10 and 20 L/min, which are breathing conditions of sitting awake and light exercise for a 5-year-old child (ICRP Citation1994). This study used a constant flow as Golshahi et al. (Citation2011) compared unsteady with steady breathing patterns, and the differences have been found negligible. This experimental setup was also used for our adult nasal replica (Guilmette and Gagliano Citation1994) at a flow rate of 20 L/min as a comparison.
Particle Bounce and Electrostatic Effects
To minimize effects of particle bounce on particle collection, a grease coating was applied in the surface of the replica by filling up and draining out (12 h) with silicon oil (Dow Corning 550 Fluid; Dow Corning Inc., Midland, MI, USA). The coating will also minimize electrostatic effects on particle collection due to the use of polar oleic acid droplet and polystyrene latex particles. To verify that the grease coating could remove the charge, a test was conducted in a stainless steel (no charge) tube of the exactly same dimensions (90° bend) and a tube from the acrylonitrile butadiene styrene material. The result was judged by the p value from the two-tailed t test. There was no significant difference between these two tubes at 0.05 significant level ( p = 0.13), which indicates that no electrostatic charge occurred after a coating was applied to the inner surface of the replica.
Sample and Statistic Analysis
After each test, replicas were disassembled. Aerosols deposited on the surface of each unit were rinsed with a solution of 50% isopropyl and 50% distilled water for oleic acid particles. In general, 100% ethyl acetate was used to dissolve PSL particles. However, the ethyl acetate can also dissolve the replica material. Although the ethyl acetate cannot be used directly to rinse PSL aerosols from the replica, 100% ethanol was used. The solution was then put in the hood for 24 hours until the ethanol was evaporated. The ethyl acetate was added to dissolve the PSL aerosols. All filters were also placed in solution for 24 hours. The relative concentrations of fluorescent tracers in the solutions were measured with a fluorometer (Model 450; Sequoia-Turner Corp., Mountain View, CA, USA). One drop (35–40 μl) of 1 N NaOH was added into each glass tube, which contained around 6 ml of sample to stabilize the fluorescence. The relative concentration of the fluorescent tracer (C) in the solution was calculated by considering the sampling flow rate (Q), sampling time (t), volume of solution (V S), and dilution factor (f), as show in following equation:
For comparison to an equation, the coefficient of determination (r 2) was used. The reference equation was convert to a linear equation. All experimental data were also calculated with the same conversion. The general r 2 equation was applied as following:
Computational Fluid Dynamics Calculations
The transport and deposition of particles were simulated with a well-tested discrete Lagrangian tracking model enhanced with near-wall treatment. Airflows were assumed to be isothermal and incompressible. The inhaled particles were assumed to be dilute and did not influence the continuous-phase, i.e., one-way coupled particle motion. To establish grid-independent and particle-count-independent results, convergence sensitivity analysis was conducted after the method of Xi et al. (Citation2011). The final grid for reporting flow field and deposition conditions consisted of approximately 1.5–1.8 million cells. The final number of the particles tracked was 60,000. More details on boundary conditions and numerical methods are available (Xi et al. Citation2011). In this study, the numerical analysis was based on the regions that are shown in . For total particle deposition, the computational fluid dynamics (CFD) data were obtained from Xi et al. (Citation2011).
RESULTS
Total Deposition of the Nasal Replica
Total deposition in the nasal replica is shown in at flow rates of 10 and 20 L/min. The CFD calculation (Xi et al. Citation2011) with the same geometry was also included as a comparison. In general, the CFD calculation is close to the experimental data for the 5-year-old child replica.
FIG. 2 Comparison of experimental data and CFD calculation for particle deposition in the 5-year-old child's nasal replica at flow rates of 10 and 20 L/min (error bar: standard deviation).
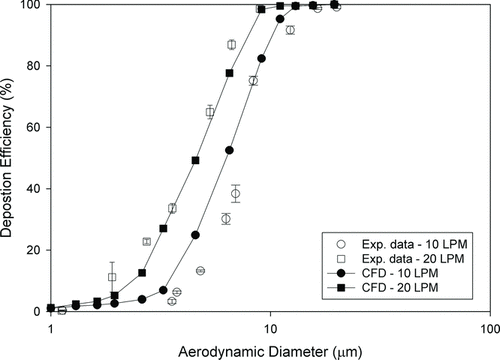
In the study of Golshahi et al. (2011), there was a very large degree of variability if data were plotted as a function of an impaction parameter (d a 2 Q) for different geometries, where d a is particle aerodynamic diameter and Q is the flow rate. As mentioned in previous section, our results were only compared to their replicas for subject 6, 7, and 10. The comparison as a function of impaction parameter was shown in . Also shown in are in vivo nasal deposition data in children (Becquemin et al. Citation1991; Bennett et al. Citation2008) and deposition data in an infant nasal airway replica (Swift Citation1991). shows that deposition data in the infant nasal replica are much higher than all other reported data. The deposition in this study are in the same range of in vivo depositon data.
FIG. 3 Deposition efficiency in a 5-year-old child's nasal replica as a function of the impaction parameter (error bar: standard deviation), compared with the deposition data of subjects 6, 7, and 10 from Golshahi et al. (Citation2011), in vivo children nasal deposition data of Becquemin et al. (Citation1991) and Bennett et al. (Citation2008) and deposition data in an infant nasal airway replica (Swift Citation1991).
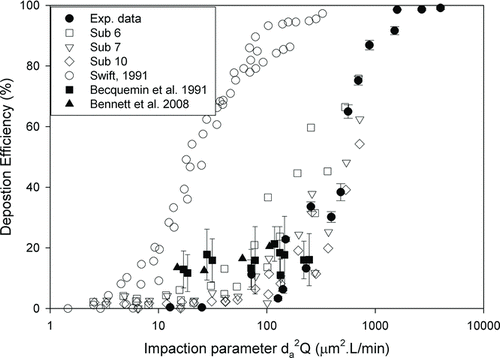
FIG. 4 Deposition efficiency in a 5-year-old child's nasal replica as a function of particle size and pressure drop (error bar: standard deviation), compared with the deposition data of subjects 6, 7, and 10 from Golshahi et al. (Citation2011) (a) and with the equation obtained by Garcia et al. (Citation2009) (b).
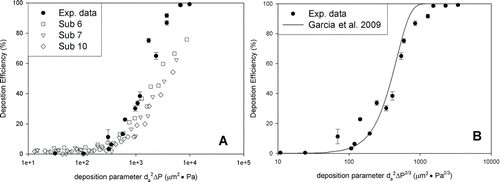
A parameter that includes the pressure drop (Δp) of a whole replica was used to reduce the scatter of deposition data (Hounam et al. Citation1971; Garcia et al. Citation2009; Golshahi et al. Citation2011; Xi et al. Citation2012). shows deposition in our replica, and three replicas (subjects 6, 7, and 10) from the Golshahi et al. (Citation2011) study as a function of d a 2Δp. In the study of Garcia et al. (Citation2009), a different pressure drop parameter (d a 2Δp 2/3) was fitted to their data using EquationEquation (3):
TABLE 2 Characteristic diameters and their corresponding references
also presents a comparison of our data to the results from Garcia et al. (Citation2009).
Several attempts have been made to correlate nasal deposition efficiency as a function of particle diameter, airway geometries, and flow rate, such as the dimensionless Stk (Stk = d a 2 U/18μd c). In the Stk, the characteristic diameter of the geometry (d c) is the key parameter. Cheng (Citation2003) used an equivalent diameter at a minimum cross section as the d c, which resulted in Stk = π0.5 d a 2 Q/18μ(A min)3/2. A single curve was obtained for deposition efficiencies in an adult replica and an infant replica as a function of the Stk. We used our dataset to examine Equation (9) in the study of Cheng. Our data appeared much lower than Cheng's equation. In addition, we studied the parameters used in the study of Golshahi et al. (Citation2011), and this empirical equation was obtained from their experimental data from 13 replicas of children 4 to 14 years old. The equation included the Stk and Re, in which the d c includes the square root of volume (V) and central length (L) of the replicas:
FIG. 5 Deposition efficiency (error bar: standard deviation) in a 5-year-old child's nasal replica as a function of Stk and Re compared with the equation developed by Golshahi et al. (Citation2011).
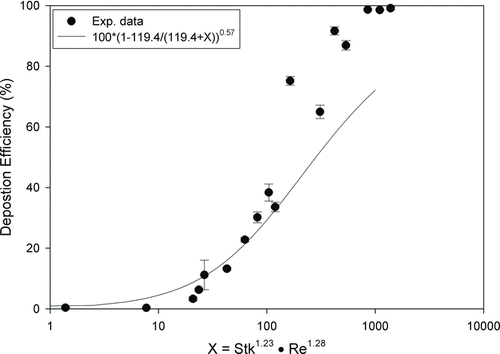
Garcia et al. (Citation2009) developed a new definition of d c, which included airway length, pressure drop, and the flow rate. Because d c is accompanied with a pressure drop, it was more reasonable to deliver all data from different geometries into one curve. We plotted our pressure drop data as a function of flow rate. The best fit equation is Δp = 0.3218Q 1.85 with a correlation coefficient r 2 of 0.999. Theoretically, the value of the exponent would be 1.75 for the pressure drop in a circular pipe (White Citation2008). We also plotted our data with the following equation:
FIG. 6 Pressure drop measured with and without laryngeal region as a function of flow rate, and fitted equations.
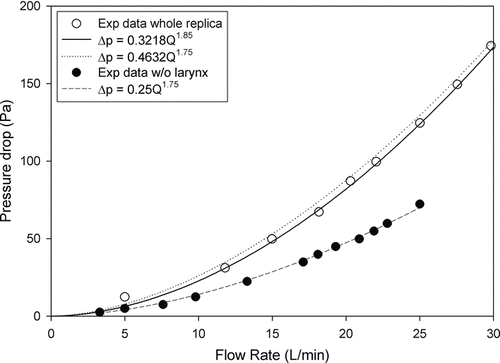
a value of d c for our nasal replica was obtained () compared with results from other studies. The R nose with and without the larynx is also listed in . In the study of Garcia et al. (Citation2009), an empirical equation was developed on the basis of the deposition data from replicas of four healthy adults. However, the Stk they used was simplified, not the real dimensionless Stk. After converting the Stk from Garcia et al. (Citation2009) to the regular Stk, a parameter of 6.53 × 10−5 was obtained between these two Stks (i.e., Stk = 6.53 × 10−5 StkGarcia). Their empirical equation can be converted to
FIG. 7 Deposition efficiency as a function of Stk, which Garcia et al. (Citation2009) developed. The Stk with different lengths and pressure drops are shown in this figure: (1) whole replica deposition efficiency vs. Stk from L nose and the whole pressure drop; (2) whole replica deposition efficiency vs. Stk from L, and the whole pressure drop; and (3); deposition efficiency without larynx vs. Stk from L nose and the pressure drop without larynx.
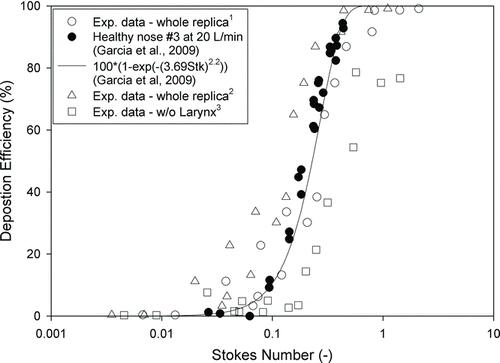
Regional Deposition
Particle deposition in different regions of the nasal replica of a child was also investigated in this study. Both experimental data and CFD calculations were carried out with the same replica and regions (four parts; see ). shows the experimental data as compared with the CFD calculation in Parts 1 and 2 at flow rates of 10 L/min (a) and 20 L/min (b). shows the comparison of in Parts 3 and 4 at flow rates of 10 (a) and 20 (b) L/min.
To investigate regional deposition, a study was carried out in an adult replica at 20 L/min, which had three parts but was minus the laryngeal and tracheal region. shows the regional deposition efficiencies at different flow rates and particle sizes (1−7 μm).
DISCUSSION
Close results were obtained between experimental data and CFD calculations, especially for the total deposition efficiency. This indicates that the CFD calculation method was acceptable for total nasal deposition. The CFD calculation in regional deposition was also found close to the experimental data in some cases but may need an improved simulation.
FIG. 9 Comparison of regional deposition between experimental data (error bar: standard deviation) and CFD calculation in posterior and laryngeal and tracheal regions at the flow rates of 10 L/min (a) and 20 L/min (b).
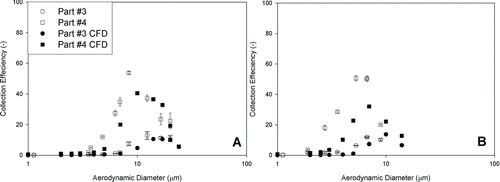
In addition to particle size and flow rate, nasal geometry was another factor that affected deposition efficiency. We compared depositions with the result of Golshahi et al. (Citation2011) for similar geometries (). Our data were in the same range with their subjects 7 and 10 but not with subject 6 that appeared higher than our data. The surface area of subject 6 is the smallest among these three replicas and our replica. This could be the factor that cause the higher deposition in subject 6. We also found the smallest As /L (L = total axial length of the replica) ratio for subject 6 among these replicas. Golshahi et al. (Citation2011) also suggested this ratio as the d c; however, we cannot compare the results without detailed data from Golshahi et al. As listed in , our replica has similar volume and surface area to the three replicas chosen from Golshahi et al. (Citation2011), but at least 20 mm less total replica length and a larger minimum bilateral cross-sectional area than the three replicas. It has been proposed that a longer airway and a smaller cross section would increase deposition efficiency. However, our results were close to those of the three subjects from Golshahi et al. (Citation2011). This indicates that the length and minimum cross-sectional dimension of the replica may not be important parameters for particle deposition.
Pressure drop in the nasal airway replica was used in these studies instead of flow rate as the impaction parameter, because the change in pressure drop reflects the changes of geometry and flow. When we plotted our deposition data as a function of d a 2Δp (), all data points collapsed into a single curve, but our data were higher than the results from the study of Golshahi et al. (Citation2011). As we discussed before, the bilateral minimum cross section of our replica is larger than the three subjects by Golshahi et al. (Citation2011). Large cross section may result in lower pressure drop at the given flow rate; thus, pushing the x-axis to the left in this replica due to lower pressure drop values. When we plotted our data as s function of d a 2Δp 2/3 (), not only did all data points collapse together, but they are also close to the nasal deposition efficiency equation (r 2 = 0.62) of the adult replicas of Garcia et al. (Citation2009). The results of pressure drop comparison may indicate that care should be taken in suggesting pressure drop as a good indicator of deposition since the regional geometry may not be included by just considering total pressure drop.
FIG. 10 Regional deposition efficiency in a child's nasal replica and an adult nasal replica for three different particle sizes.
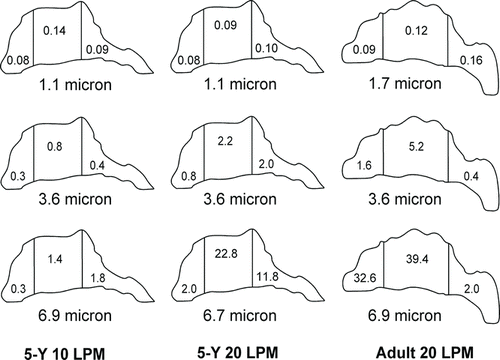
Several attempts have been made to unify the nasal deposition efficiencies from human nasal replicas of differing geometries and age groups with an Stk (Cheng Citation2003; Storey-Bishoff et al. Citation2008; Garcia et al. Citation2009; Golshahi et al. Citation2011). The Stk combines the flow rate, aerodynamic particle size, and a characteristic diameter of the geometry. We tested the Stk that Garcia et al. (Citation2009) and Golshahi et al. (Citation2011) proposed. Golshahi et al. (Citation2011) have investigated many forms of the Stk number with different characteristic diameters and have found that the one proposed by Garcia et al. (Citation2009), and two other forms (average perimeters and average cross section) fit well with their deposition in replicas of children and adolescents.
Our data were also plotted with the same parameters used in the study of Golshahi et al. (). Our data were around their empirical equation for deposition efficiency below 50%. However, for deposition efficiency higher than 50%, differences were found. The overall r 2 is 0.24. Most of our data with higher deposition efficiency were large particles that were out of the particle size range in the study of Golshahi et al. (Citation2011). Because of the experimental limitation, they could only test particles up to 5.3 μm. In our study, most of the deposition efficiencies were below 50% for particles below 5.3 μm. This indicates that a correlation cannot be extrapolated to outside of the range of its validity.
A new definition of characteristic diameter was developed in the study of Garcia et al. (Citation2009). This diameter includes the length of the nasal replica, flow rate, and pressure drop. Our data actually fit quite well (r 2 = 0.60) with their results from adult nasal replicas when plotted against the Stk using their characteristic diameter. This indicates that the pressure drop is a better parameter for particle deposition in nasal airways than the average diameters of various forms used by many groups. Because nasal airway geometry is complex, there is no simple way to calculate the pressure drop, which must be obtained by measurement from each replica. We also noted that in the study of Garcia et al., the Stk was in a simplified form, d a 2 Q/d c 3, which is not dimensionless as it should be. We used the dimensionless Stk and showed that our data from the child's replica and their data from adult replicas can be fitted with a single curve.
This study is the first to compare regional experimental data to the CFD calculation using the same nasal geometry and tested in series both in experiments and complementary CFD simulations. Although we still have discrepancies between the experimental and simulation results, they show similar trends. For the lower flow rate, the CFD calculation overestimated the deposition in the anterior region (1) but underestimated the deposition in the turbinate region (2). Agreement was found in the anterior region for 20 L/min; however, the CFD was still underestimated in the turbinate region (2). The underestimation of turbinate deposition by CFD than experiments in this study might result from the differences between an idealized smooth-surface computer model and a replica cast that inevitably has artifacts and rough surface due to limitations of current rapid-prototyping technique. Even partial or slight blockage in the narrow turbinate region will increase particle deposition in this region. If we consider these two regions as one, the comparison was much closer. In the relatively simple posterior (3) and laryngeal and tracheal (4) regions, our CFD calculation agreed with the experimental data at 10 L/min. However, the CFD calculations were lower than the experimental data at 20 L/min with the same trend.
A comparison with an adult replica in the same regions was presented in this study. For small particles, deposition efficiencies were very low in each region. Most of the small particles penetrated the replicas. However, with increased particle size, the deposition efficiency increased in all regions. Among these three regions, the turbinate region received more particles than the others. A high deposition was found in the anterior region in the adult replica but not in the child replica. The reason for this finding is that our adult replica is twice as long in the anterior region than in the child's replica. The geometry of this region in the adult replica is also more complicated.
Many theoretical and experimental issues affect regional deposition, and it is not easy to obtain good agreement in regional deposition. More studies should be carried out using different geometries.
CONCLUSION
This study investigated aerosol deposition in a nasal replica of a 5-year-old child. With the total deposition, the CFD calculation showed agreement with the experimental data. This study also added to the database for aerosol deposition in a child's nasal cavity. The deposition efficiency is strongly related to pressure drop. Regional in vitro deposition information for a child's nasal area was investigated first with the comparison of the CFD calculation. As this is the nasal regional deposition study with completed nasal geometry, no empirical equation was developed until further studies are carried out. The current findings may be useful in future application to drug delivery using nasal spray.
uast_a_749341_sm5979.zip
Download Zip (40.7 KB)REFERENCES
- Becquemin , M. H. , Swift , D. L. , Bouchikhi , A. , Roy , M. and Teillac , A. 1991 . Particle Deposition and Resistance in the Noses of Adults and Children . Eur. Respir. J. , 4 : 694 – 702 .
- Bennett , W. D. , Zeman , K. L. and Jarabek , A. M. 2008 . Nasal Contribution to Breathing and Fine Particle Deposition in Children Versus Adults . J. Toxicol. Environ. Health Part A , 71 : 227 – 237 .
- Charlton , S. T. , Davis , S. S. and Illum , L. 2007 . Evaluation of Effect of Ephedrine on the Transport of Drugs from the Nasal Cavity to the Systemic Circulation and the Central Nervous System . J. Drug Target , 15 : 370 – 377 .
- Cheng , Y. S. 2003 . Aerosol Deposition in the Extrathoracic Region . Aerosol Sci. Technol. , 37 : 659 – 671 .
- Cheng , Y. S. , Holmes , T. D. , Gao , J. , Guilmette , R. A. , Li , S. , Surakitbanharn , Y. and Rowlings , C. 2001 . Characterization of Nasal Spray Pumps and Deposition Pattern in a Replica of the Human Nasal Airway . J. Aerosol. Med. , 14 : 267 – 280 .
- Cheng , Y. S. , Smith , S. M. , Yeh , H. C. , Kim , D. B. , Cheng , K. H. and Swift , D. L. 1995 . Deposition of Ultrafine Aerosols and Thoron Progeny in Replicas of Nasal Airways of Young Children . Aerosol Sci. Technol. , 23 : 541 – 552 .
- Dhuria , S. V. , Hanson , L. R. and Frey , W. H. 2nd. 2010 . Intranasal Delivery to the Central Nervous System: Mechanisms and Experimental Considerations . Pharm. Sci. , 99 : 1654 – 1673 .
- Dieffenbach , C. W. , Tramont , E. C. and Plaeger , S. F. 2009 . Innate (General or Nonspecific) Host Defense Mechanisms, in Principles and Practice of Infectious Diseases, , 7th ed., , Edited by: Mandell , G. L. , Bennett , J. E. and Dolin , R. FL : Churchill Livingstone . , pp. 37–48
- Garcia , G. J. , Tewksbury , E. W. , Wong , B. A. and Kimbell , J. S. 2009 . Interindividual Variability in Nasal Filtration as a Function of Nasal Cavity Geometry . J. Aerosol Med. Pulm. Drug Deliv. , 22 : 139 – 155 .
- Golshahi , L. , Finlay , W. H. , Olfert , J. S. , Thompson , R. B. and Noga , M. L. 2010 . Deposition of Inhaled Ultrafine Aerosols in Replicas of Nasal Airways of Infants . Aerosol Sci. Technol. , 44 : 741 – 752 .
- Golshahi , L. , Noga , M. L. , Thompson , R. B. and Finlay , W. H. 2011 . In Vitro Deposition Measurement of Inhaled Micrometer-Sized Particles in Extrathoracic Airways of Children and Adolescents During Nose Breathing . J. Aerosol Sci. , 42 : 474 – 488 .
- Guilmette , R. A. and Gagliano , T. J. 1994 . Construction of a Model of Human Nasal Airways Using in Vivo Morphological Data . Ann. Occup. Hyg. , 38 : 69 – 75 .
- Hounam , R. F. , Black , A. and Walsh , M. 1971 . The Deposition of Aerosol Particles in the Nasopharyngeal Region of the Human Respiratory Tract . J. Aerosol Sci. , 2 : 47 – 61 .
- International Commission on Radiological Protection (ICRP), Publication 66 . 1994 . Human Respiratory Tract Model for Radiological Protection , Oxford : Pergamon Press .
- Janssens , H. M. , DeJongste , J. C. , Fokkens , W. J. , Robben , S. G. F. , Wouters , K. and Tiddens , H. A. W. M. 2001 . The Sophia Anatomical Infant Nose–Throat (Saint) Model: A Valuable Tool to Study Aerosol Deposition in Infants . J. Aerosol Med. Pulm. Drug Deliv. , 14 : 433 – 441 .
- Storey-Bishoff , J. , Noga , M. and Finlay , W. H. 2008 . Deposition of Micrometer-Sized Aerosol Particles in Infant Nasal Airway Replicas . J. Aerosol Sci. , 39 : 1055 – 1065 .
- Swift , D. L. 1991 . Inspiratory Inertial Deposition of Aerosols in Human Nasal Airway Replicate Casts: Implication for the Proposed NCRP Lung Model . Radiat. Protect. Dosim. , 38 : 29 – 34 .
- White , F. M. 2008 . Fluid Mechanics , New York : McGraw-Hill Companies, Inc. .
- Xi , J. X. , Berlinski , A. , Zhou , Y. , Greenberg , B. and Ou , X. W. 2012 . Breathing Resistance and Ultrafine Particle Deposition in Nasal Airways of a Newborn, an Infant, a Child, and an Adult . Ann. Biomed. Eng. , [Epub ahead of print].
- Xi , J. X. , Si , X. H. , Kim , J. W. and Berlinski , A. 2011 . Simulation of Air Flow and Aerosol Deposition in the Nasal Cavity of a 5-Year-Old Child . J. Aerosol Sci. , 42 : 156 – 173 .
- Zhou , Y. , Sun , J. J. and Cheng , Y. S. 2011 . Comparison of Deposition in the USP and Physical Mouth-Throat Models with Solid and Liquid Particles . J. Aerosol Med. Pulm. Drug Deliv , 24 : 277 – 284 .