Abstract
An integrated airborne system comprising two condensation particle counters (CPCs, models 3771 and 3772, TSI Inc.) and a low-pressure impactor (LPI) has been developed (LPI-CPCs) for fast measurement of mode-segregated aerosol number concentration. The CPC 3771 is connected to the LPI to measure aerosol number concentrations below 0.17 μm in aerodynamic diameter, while the CPC 3772 directly measures the total aerosol number concentration. The former approximately corresponds to the Aitken mode fraction of the aerosol number concentration. The key concept is that the cutoff diameter of the LPI (aerodynamic diameter at which the transmission efficiency is 50%) is controlled by simultaneously modifying the pressure and flow rate through the LPI. The instrument was deployed onboard the King Air B200T aircraft during the Aerosol Radiative Forcing in East Asia (A-FORCE) conducted over the western Pacific in the spring of 2009. The results from the aircraft measurements, together with those from laboratory experiments, are used to demonstrate the in-flight performance of the instrument. We propose that fast airborne measurement of mode-segregated aerosols by LPI-CPCs is useful for a better understanding of the variability of aerosol number concentration in the troposphere.
Copyright 2013 American Association for Aerosol Research
1. INTRODUCTION
Particle number concentration is one of the most fundamental parameters for characterizing atmospheric aerosols. The majority of aerosol particles are found in the nucleation mode (diameters smaller than ∼0.01 μm) and the Aitken mode (diameters ranging from ∼0.01 to ∼0.1 μm). Aitken-mode particles can grow into the accumulation mode (diameters ranging from ∼0.1 to 1 μm) by coagulation and condensation. Accumulation-mode particles often contribute a major fraction of cloud condensation nuclei (CCN).
Condensation nucleus counters (CNCs) or condensation particle counters (CPCs) manufactured by TSI Inc. (Minneapolis, MN, USA) have been used for measuring number concentrations of aerosol particles as small as a few nanometers with a time resolution of the order of seconds onboard aircraft (Clarke Citation1993; Brock et al. Citation2000; Weber et al. Citation2003; Weigelt et al. Citation2009). A CPC can be combined with a differential mobility analyzer (DMA) to obtain size-resolved aerosol number concentrations with a time resolution of the order of minutes. The fast integrated mobility spectrometer (FIMS), which consists of an electrostatic separator (similar to DMA), condenser, and optical detector, can be used for the rapid measurement of aerosol-size distributions (Olfert and Wang Citation2009). Care should be taken when operating an airborne DMA system because the transfer function of the DMA is sensitive to changes in pressure and flow rate. The use of a radioactive source as a neutralizer may be another difficulty when deploying a DMA onboard aircraft in Japan because of the strict regulation of radioactive materials.
We have developed an integrated airborne system consisting of two CPCs and a low-pressure impactor (LPI) for the fast measurement of mode-segregated (total and Aitken fraction) aerosol number concentration. The system is hereafter referred to as the LPI-CPCs. Although the combination of an impactor and CPC for aircraft measurements was previously reported by Hermann and Wiedensohler (Citation2001), a detailed description was not presented. The most important advantage of our system is in the use of an online-controlled LPI as a front-end device for airborne measurements of ultrafine particles. The key concept is that the cutoff diameter of the LPI (aerodynamic diameter at which the transmission efficiency is 50%) is controlled by simultaneously modifying the pressure and flow rate through the LPI. Compared to highly size-resolved but relatively delicate, slow measurements by a DMA-CPC system, the LPI-CPCs can provide a simple but effective measure of dynamic changes in aerosol number size distributions with high horizontal/vertical resolutions. This point will be well demonstrated by vertical profile measurements shown in Section 3.2.
An additional rationale for the design of this system is that it can be combined with any type of aerosol instrument. A combination with an online aerosol composition analyzer will enable fast and effective measurements of number concentration and chemical composition of ultrafine particles with a unified size category. Such data would be useful for validating chemistry-transport models, many of which utilize a simplified, modal approximation for representing aerosol size distributions.
The major purpose of this article is to describe the concept of the LPI-CPCs and also to demonstrate the in-flight performance of the instrument during an aircraft measurement campaign (Aerosol Radiative Forcing in East Asia: A-FORCE) conducted in the spring of 2009 over the western Pacific (Oshima et al. Citation2012).
2. METHODS
2.1. Instrument Description
A schematic diagram of the LPI-CPCs is shown in . Two commercial butanol CPCs (TSI models 3771 and 3772, which are functionally identical) are used to detect particles with mobility diameters (dm ) larger than 0.01 μm. One CPC samples air directly from the inlet to measure the aerosol number concentrations above 0.01 μm in mobility diameter. The second CPC samples air downstream of an LPI to measure the aerosol number concentrations below a certain threshold diameter. The former measurement is referred to as total CN and the latter as PM x CN, where x is the LPI aerodynamic cutoff diameter in micrometers. The performance of the CPC 3771 under low-pressure conditions has been evaluated by our previous experiments (Takegawa and Sakurai Citation2011). The other components of the system include an LPI, mass flow meters, pressure sensors, temperature sensors, DC vacuum pumps, and electronics and a laptop for data acquisition and control.
FIG. 1 Schematic diagram of LPI-CPCs (MFM: mass flow meter; T: temperature sensor; P: pressure sensor). The pressure sensors incorporated in the 3771 and 3772 (not explicitly shown) are used to measure the pressure downstream and upstream of the LPI, respectively. The additional pressure sensor downstream of the LPI is used for backup and checking consistency.
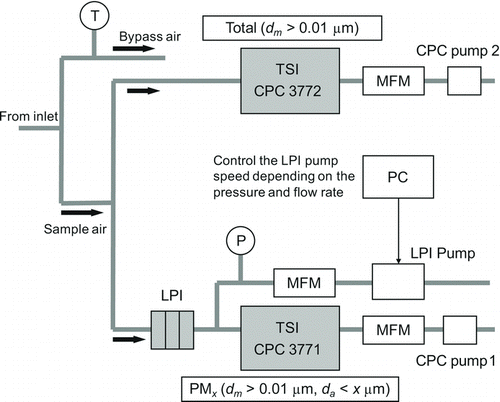
The LPI uses two stages from the commercial 10-stage, circular jet cascade impactors (MAIS, Tokyo Dylec Corp., Tokyo, Japan). These were chosen to provide a cutoff diameter of 0.1–0.2 μm at inlet pressures of 350–1010 hPa within reasonable ranges of flow rates (below 20 l min−1) and Reynolds numbers (500–3000, Marple and Willeke Citation1976). The dimensions of the impactors used in our system are shown in . Stage 2 (nominal cutoff diameter of ∼2 μm) was used as a “precollector” to remove dusts and debris that may clog the nozzles of stage 1. The ratios of the separation distance (S) to nozzle diameter (W) are within the recommended value of 1–5 (Hinds Citation1999). The flow rate through the LPI was varied by changing the rotation speed of a DC pump (LPI pump). The cutoff diameter of stage 2 was not actively controlled because a subtle difference in the cutoff diameter does not yield a significant difference in the measured aerosol number concentration. The cutoff diameter of stage 1 was controlled by a custom-made feedback system, as described later in this section.
TABLE 1 Dimensions of the low-pressure impactor (LPI)
Silicon grease was manually smeared on the impaction plates to reduce particle bounce. We also tested Apiezon L vacuum grease, which is widely used for impactors, but it turned out that silicon grease was more resistant against blow off than Apiezon L for our instrument. Although the coating of impaction plates should be performed using a uniform greasing apparatus (Hillamo and Kauppinen Citation1991), such a device for the silicon grease was not available at the time of the aircraft measurements. Nevertheless, we did not find a significant difference in the reproducibility of the LPI performance, at least under atmospheric pressure conditions.
The performance of an impactor is generally characterized by the Stokes number of particles in the nozzle (Marple and Willeke Citation1976; Hinds Citation1999). The cutoff diameter can be calculated (dm 50,calc) using the measured mass flow rates and pressures, i.e.,
The set value of the cutoff diameter (da 50,set) can be arbitrarily defined. The voltage applied to the LPI pump (V pump) was controlled to keep da 50,calc close to da 50,set: i.e., the voltage increment was set proportional to the difference between da 50,calc and da 50,set. Varying the LPI pump speed can simultaneously modify the pressure and flow rate through the LPI (hence the Stokes number of particles). This is beneficial for aircraft measurements because the size of the instrument can be significantly reduced compared to the use of mass flow controllers, which generally require a large vacuum pump to generate sufficient pressure differences.
In the current configuration, the cutoff diameter of da = 0.17 μm, which corresponds to dm = 0.11–0.13 μm assuming ρp = 1.5 g cm−3 (Section 3.2), was chosen for the aircraft measurements. The choice of da = 0.17 μm was determined based on laboratory experiments and test flights by optimizing various factors, including the pressure ratio across the nozzle, Reynolds number, LPI transmission efficiency, and stability of flow rates. Ideally, a slightly lower value of dm (e.g., 0.08–0.1 μm) is preferred for better representation of the Aitken fraction. To achieve this, we need to modify the dimensions of impactor stage 1, which may be performed in future studies.
2.2. Laboratory Evaluation
Laboratory experiments were conducted prior to the aircraft measurements to test the overall performance of the system and also to determine an appropriate Stk50 value for the LPI (hereafter referred to as laboratory experiment [1]). Additional laboratory experiments were performed after the aircraft measurement campaign to further investigate the instrumental performance (hereafter referred to as laboratory experiment [2]). Monodisperse, dry ammonium sulfate ((NH4)2SO4) particles were generated using a Collison atomizer (Model 3076, TSI, Inc., Minneapolis, MN, USA), diffusion dryer, and a differential mobility analyzer (DMA, Model 3081, TSI, Inc., Minneapolis, MN, USA). The monodisperse aerosol flow was diluted using particle-free air to match with the total flow rate of the LPI-CPCs (∼14 L min−1 STP for experiments at 1 atm). A mixing chamber was used to uniformly mix the aerosol and dilution flows. The pressure can be reduced using a valve downstream of the mixing chamber. Prior to the laboratory experiment [1], we confirmed that the detection efficiencies of these two CPCs at dm = 0.06 μm (nearly equivalent to the asymptotic detection efficiencies at larger diameters) agreed to within <1% at 1 atm.
2.3. Aircraft Deployment
Details of the A-FORCE campaign were presented by Oshima et al. (Citation2012), and thus only a brief description is given here. The instruments deployed onboard the King Air B200T aircraft also included a single particle soot photometer (SP2, DMT, Inc.) (Moteki et al. Citation2012). The sample air was aspirated through a shrouded inlet nozzle (DMT, Inc., Boulder, CO, USA) mounted on the top of the aircraft fuselage. The design of the inlet nozzle was basically the same as that given by McNaughton et al. (Citation2007). The sample air was drawn into the aircraft cabin through a stainless steel tube (outer diameter [OD] of 25.4 mm) and split to the main and bypass lines. The main line was then split to the LPI-CPCs and SP2 through Y-splitters and reducers. The bypass line was connected to two piston pumps to achieve nearly isokinetic flows through the inlet nozzle. We use the data obtained during flight 15 (from Kagoshima [31.8°N, 130.7°E] to Gimpo [37.6°N, 126.8°E]) for assessing in-flight performance of the LPI-CPCs.
The calibration of the LPI during the campaign was rather limited because of the very tight assembly of the instrument racks onboard the aircraft. The transmission efficiency for 0.35-μm (NH4)2SO4 or polystyrene latex (PSL) particles was checked every one to three flights to ensure that there was no significant bounce of larger particles. Grease was smeared on the impaction plate before the campaign and exchanged twice during the campaign. The LPI performance was tested near the end of the campaign, and the result was found to be consistent with the laboratory experiment [1].
3. RESULTS
3.1. Laboratory Calibration
shows the transmission efficiency of the LPI as a function of Stk1/2 for inlet pressures (P in) of 980 and 475 hPa obtained during the laboratory experiment [1]. The transmission efficiency was determined by the concentration ratio of 3771 to 3772. The key variables of the experiment including U, pressure ratio (r), and Reynolds number (Re) are shown in . The experimental Stk50 1/2 was found to be 0.445 and 0.454 for 980 and 475 hPa, respectively. The average value of 0.450 was smaller than the default value (0.490) and close to the one determined for the Berner LPI (0.465: Hillamo and Kauppinen Citation1991) and for the Electrical LPI (0.456: Marjamäki et al. Citation2000). In , the LPI transmission efficiency for smaller diameters at 475 hPa was lower than that at 980 hPa. This is more clearly illustrated in , showing the dependence of the transmission efficiency on P in for dm = 0.05 μm. The error bars represent random variability and do not include the systematic uncertainties in the flow rate calibration (∼1%: Takegawa and Sakurai Citation2011) because it is cancelled out by taking the ratio of two CPCs.
FIG. 2 Transmission efficiency of the LPI as a function of the square root of Stk for inlet pressures (P in) of 980 and 475 hPa. The solid and dashed lines represent the fitting results for 980 and 475 hPa, respectively. Corresponding aerodynamic diameters at 980 hPa are shown on the top axis.
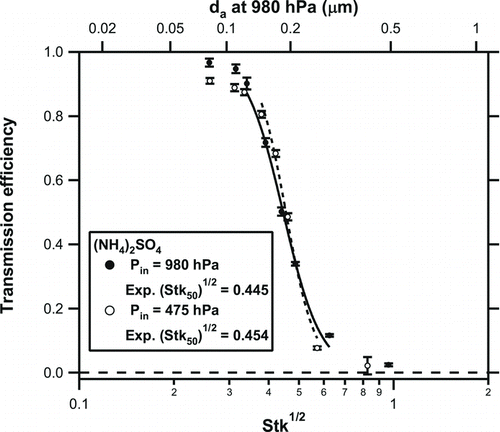
TABLE 2 Laboratory evaluation of the LPI stage 1a
FIG. 3 Dependence of the transmission efficiency of the LPI on P in for particles with a mobility diameter (dm ) of 0.05 μm obtained before the aircraft measurement campaign (laboratory experiment [1]). The solid line represents the fitting result. The calculated cutoff diameter (da 50,calc) and volumetric flow rate through the LPI ranged from 0.23 to 0.19 μm and from 4 to 9 L min−1, respectively, at inlet pressures ranging from 380 to 980 hPa.
![FIG. 3 Dependence of the transmission efficiency of the LPI on P in for particles with a mobility diameter (dm ) of 0.05 μm obtained before the aircraft measurement campaign (laboratory experiment [1]). The solid line represents the fitting result. The calculated cutoff diameter (da 50,calc) and volumetric flow rate through the LPI ranged from 0.23 to 0.19 μm and from 4 to 9 L min−1, respectively, at inlet pressures ranging from 380 to 980 hPa.](/cms/asset/326ca635-0464-46e7-bef2-20538b1ebd83/uast_a_822462_o_f0003g.gif)
As mentioned previously, additional laboratory experiments (laboratory experiment [2]) were performed after the aircraft measurement campaign to further investigate this trend. The decreasing trend of the transmission efficiency with decreasing pressures was commonly found for dm = 0.035, 0.040, 0.050, and 0.062 μm (see the online supplemental information). A possible mechanism for the loss of these smaller particles may be Brownian diffusion. Hillamo and Kauppinen (Citation1991) and Kelly and McMurry (Citation1992) also found a loss of smaller particles in impactors (dm < 0.05 μm, well below the cutoff diameter). The Brownian diffusion may explain the observed loss to some extent, but it is unlikely that they are the major cause considering the weak dependence on the particle diameter and flow rate. Because the loss mechanism is not well understood at present, we do not make any correction for this dependency.
Here, we use the following equation to fit the data points in :
The fitting results are a = 0.963 ± 0.003, b = 162 ± 19, and p 0 = 11 ± 44. This equation will be used to assess possible uncertainties during the aircraft measurements.
TABLE 3 Possible errors (1σ)a
FIG. 4 Time series of some key variables of the LPI, including (a) P in, jet velocity (U), and pressure ratio (r); (b) Reynolds number (Re), calculated cutoff diameter (da 50,calc); and (c) total CN concentration, and PM0.17 CN concentration during flight 15 on 12 April 2009. The data are 1-s averages.
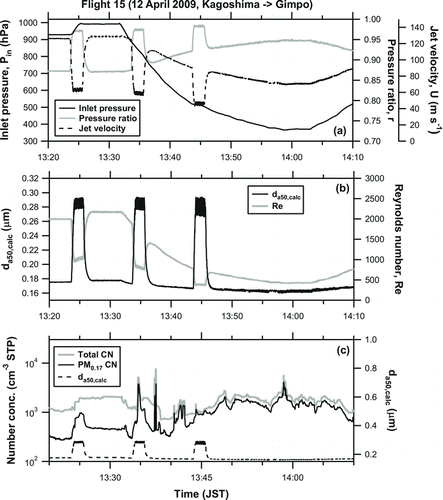
3.2. In-Flight Performance
show time series of some key variables of the LPI, including P in, U, r, Re, and da 50,calc during flight 15. One-second average data are used in these plots. The data acquisition software modulated V pump every 10 min for the performance check, which is illustrated by the periodic increase of da 50,calc in (hereafter referred to as slow pump mode). Effects of the slow pump mode are described later in this section. Note that da 50,calc showed relatively large fluctuations in slow pump mode because V pump was close to the lower limit. The modulation was not performed at P in < 450 hPa because V pump became too low to stabilize the pump operation. Except for the slow pump mode, the da 50,calc value was kept nearly constant (0.16 and 0.18 μm at 350 and 1000 hPa, respectively, with an average value of 0.17 μm), and the Reynolds numbers were mostly within the recommended range (500–3000). These results demonstrate that the feedback system successfully controlled the da 50,calc value even though the inlet pressure significantly changed with ascent/descent of the aircraft. Hereafter, the CPC 3771 data obtained downstream of the LPI are referred to as PM0.17 CN.
The relationship between aerodynamic and mobility diameters depends on the density of particles. If we assume ρp = 1.5 g cm−3 (mixtures of inorganic and organic compounds), the cutoff diameters range from 0.11 to 0.13 μm in mobility diameter at inlet pressures ranging from 350 and 1000 hPa. Thus, the PM0.17 CN approximately corresponds to the Aitken mode fraction.
shows time series of the total and PM0.17 CN concentrations for the same period. In the slow pump mode, the transmission efficiency of the LPI likely covered the majority of aerosol number size distributions (except for some cases where a substantial fraction of the aerosol number concentration was included in the accumulation mode). In fact, we can see that the two concentration data sets became closer in this mode. The slow pump mode is useful for detecting degradation of the instrumental performance that cannot be easily recognized from housekeeping data (such as flooding of butanol in the optics).
FIG. 5 Vertical profiles of (a) total CN concentration and (b) the ratio of PM0.17 to the total CN concentration (PM0.17/total ratio) observed over the Yellow Sea during flight 15. Open and solid circles represent the aircraft descent and ascent data, respectively. The data are 10-s averages. Error bars for some selected data points (at ∼1000, 700, 350 hPa) are shown. See Section 3.2 for details of the error estimate.
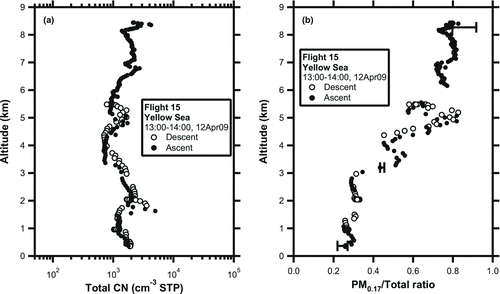
summarizes possible errors in total CN, PM0.17 CN, and (total − PM0.17) CN under various conditions. Note that the discussion in this section focuses on systematic errors because random errors were mostly below 1% for 10-s average data. The (total − PM0.17) CN values approximately represent the aerosol number concentrations in the accumulation mode, which has been used for the analysis of aerosol–cloud interaction by Koike et al. (Citation2012). Errors in total CN are primarily due to the pressure dependence of the minimum detectable diameter (Takegawa and Sakurai Citation2011), which possibly leads to an underestimation of “true” total CN concentrations above 0.01 μm, depending on aerosol number size distributions in ambient air. Errors in PM0.17 CN are due to the pressure dependencies of the minimum detectable diameter, LPI cutoff diameter, and LPI transmission efficiency. As for the pressure dependencies of the minimum detectable diameter and LPI cutoff diameter, we assume typical number size distributions of ambient aerosol particles based on Takegawa and Sakurai (Citation2011) (originally quoted from Seinfeld and Pandis [Citation2006]): i.e., “remote continental air” for 1000 and 700 hPa and “urban air” for 350 hPa. The use of “urban air” instead of “free tropospheric air” for 350 hPa is simply because it appears to be more appropriate considering the size distribution measured by the SP2 (not shown). As for the pressure dependence of the LPI transmission efficiency, we use ϵ(p) from EquationEquation (2). We assume that the pressure dependence of the minimum detectable diameter is cancelled out when calculating the (total − PM0.17) CN.
shows vertical profiles of total CN concentration and the ratio of PM0.17 CN to total CN concentration (PM0.17/total ratio) observed over the Yellow Sea during flight 15. The data in the slow pump mode were removed. The good agreement in the vertical fine structure between the data obtained during descent and ascent indicates that there was no hysteresis error associated with pressure changes. The total number showed small changes with altitude, while the PM0.17/total ratio exhibited lower values in the boundary layer than in the free troposphere, suggesting a dominance of Aitken-mode particles at higher altitudes. There was a sharp gradient in the vertical profile of the PM0.17/total ratio from the boundary layer to the free troposphere (with the variability exceeding the possible uncertainty), suggesting a significant shift in the aerosol number size distributions above the boundary layer. Similar vertical profiles were frequently observed during other flights (not shown). Although the size-resolving capacity is limited compared to DMA-CPC measurements, the dynamic changes in aerosol number size distributions were successfully observed with reasonable accuracy and high vertical resolution. A more detailed analysis of these results will be presented elsewhere.
4. DISCUSSION AND SUMMARY
We have developed an integrated airborne system consisting of two CPCs and an LPI for fast measurement of mode-segregated (PM0.17 and total) aerosol number concentration. The key concept is that the cutoff diameter of the LPI is controlled by simultaneously modifying the pressure and flow rate through the LPI. The transmission efficiency of the LPI was evaluated in the laboratory using monodisperse (NH4)2SO4 particles at inlet pressures of 980 and 475 hPa. The average Stk50 1/2 was experimentally determined to be 0.450, which was close to the values previously reported for other LPIs.
The in-flight performance of the instrument was evaluated using data obtained during the A-FORCE campaign (March–April 2009). The cutoff diameter calculated using the measured pressure and flow rate was found to be nearly constant at 0.17 ± 0.01 μm, demonstrating that the system successfully controlled the cutoff diameter even though the inlet pressure significantly changed with ascent/descent of the aircraft. If we assume ρp = 1.5 g cm−3, the cutoff diameter was calculated to be 0.11–0.13 μm in mobility diameter. Thus, the PM0.17 CN approximately corresponded to the Aitken mode fraction of the aerosol number concentration.
During the aircraft measurements, we frequently observed a sharp gradient in the vertical profile of PM0.17/total ratio from the boundary layer to the free troposphere, suggesting a significant shift in the aerosol number size distributions above the boundary layer. These data demonstrated a benefit of fast measurements of mode-segregated aerosol number concentrations.
Finally, as described in Section 1, our LPI system can be readily combined with other instruments including an aerosol composition analyzer as long as the sample flow rate of the analyzer is below a few liters per minute. A combination with an online aerosol composition analyzer will enable fast measurement of mode-segregated aerosol chemical composition onboard aircraft.
Takegawa_LPI-CPC_SI.zip
Download Zip (124.6 KB)Acknowledgments
This study was funded by the Grant-in-Aid for Scientific Research of the Japan Society for the Promotion of Science (JSPS) and the Global Environment Research Fund of the Japanese Ministry of the Environment (A-0803 and A-1101).
[Supplementary materials are available for this article. Go to the publisher's online edition of Aerosol Science and Technology to view the free supplementary files.]
REFERENCES
- Brock , C. A. , Schröder , F. , Kärcher , B. , Petzold , A. , Busen , R. and Fiebig , M. 2000 . Ultrafine Particle Size Distribution in Aircraft Exhaust Plumes . J. Geophys. Res. , 105 : 26555 – 26567 .
- Clarke , A. D. 1993 . Atmospheric Nuclei in the Pacific Midtroposphere: Their Nature, Concentration, and Evolution . J. Geophys. Res. , 98 : 20633 – 20647 .
- Flagan , R. C. 1982 . Compressible Flow Inertial Impactors . J. Colloid Interface Sci. , 87 : 291 – 299 .
- Hering , S. V. 1987 . Calibration of the QCM Impactor for Stratospheric Sampling . Aerosol Sci. Technol. , 7 : 257 – 274 .
- Hermann , M. and Wiedensohler , A. 2001 . Counting Efficiency of Condensation Particle Counters at Low-Pressures with Illustrative Data from the Upper Troposphere . J. Aerosol Sci. , 32 : 975 – 991 .
- Hillamo , R. E. and Kauppinen , E. I. 1991 . On the Performance of the Berner Low Pressure Impactor . Aerosol Sci. Technol. , 14 : 33 – 47 .
- Hinds , W. C. 1999 . Aerosol Technology: Properties, Behavior, and Measurement of Airborne Particles , Wiley-Interscience, New York .
- Kelly , W. P. and McMurry , P. H. 1992 . Measurement of Particle Density by Inertial Classification of Differential Mobility Analyzer-Generated Monodisperse Aerosols . Aerosol Sci. Technol. , 17 : 199 – 212 .
- Koike , M. , Takegawa , N. , Moteki , N. , Kondo , Y. , Nakamura , H. and Kita , K. 2012 . Measurements of Regional-Scale Aerosol Impacts on Cloud Microphysics over the East China Sea: Possible Influences of Warm Sea Surface Temperature over the Kuroshio Ocean Current . J. Geophys. Res. , 117 : D17205 doi: 10.1029/2011JD017324
- Marjamäki , M. , Keskinen , J. , Chen , D.-R. and Pui , D. Y. H. 2000 . Performance Evaluation of the Electrical Low-Pressure Impactor (ELPI) . J. Aerosol Sci. , 31 : 249 – 261 .
- Marple , V. and Willeke , K. 1976 . Impactor Design . Atmos. Environ. , 10 : 891 – 896 .
- McNaughton , C. S. , Clarke , A. D. , Howell , S. G. , Pinkerton , M. , Anderson , B. Thornhill , L. 2007 . Results from the DC-8 Inlet Characterization Experiment (DICE): Airborne Versus Surface Sampling of Mineral Dust and Sea Salt Aerosols . Aerosol Sci. Technol. , 41 : 136 – 159 .
- Moteki , N. , Kondo , Y. , Oshima , N. , Takegawa , N. , Koike , M. Kita , K. 2012 . Size Dependence of Wet Removal of Black Carbon Aerosols during Transport from the Boundary Layer to the Free Troposphere . Geophys. Res. Lett. , 39 : L13802 doi: 10.1029/2012GL052034
- Olfert , J. S. and Wang , J. 2009 . Dynamic Characteristics of a Fast-Response Aerosol Size Spectrometer . Aerosol Sci. Technol. , 43 : 97 – 111 .
- Oshima , N. , Kondo , Y. , Moteki , N. , Takegawa , N. , Koike , M. Kita , K. 2012 . Wet Removal of Black Carbon in Asian Outflow: Aerosol Radiative Forcing in East Asia (A-FORCE) Aircraft Campaign . J. Geophys. Res. , 117 : D03204 doi: 10.1029/2011JD016552
- Seinfeld , J. H. and Pandis , S. N. 2006 . Atmospheric Chemistry and Physics, , 2nd ed. , New York : John Wiley and Sons .
- Takegawa , N. and Sakurai , H. 2011 . Laboratory Evaluation of a TSI Condensation Particle Counter (Model 3771) Under Airborne Measurement Conditions . Aerosol Sci. Technol. , 45 : 272 – 283 .
- Weber , R. J. , Lee , S. , Chen , G. , Wang , B. , Kapustin , V. Moore , K. 2003 . New Particle Formation in Anthropogenic Plumes Advecting from Asia Observed During TRACE-P . J. Geophys. Res. , 108 : 8814 doi: 10.1029/2002JD003112
- Weigelt , A. , Hermann , M. , van Velthoven , P. F. J. , Brenninkmeijer , C. A. M. , Schlaf , G. Zahn , A. 2009 . Influence of Clouds on Aerosol Particle Number Concentrations in the Upper Troposphere . J. Geophys. Res. , 114 : D01204 doi: 10.1029/2008JD009805