Abstract
Results of a numerical study of the RespiCon sampler performance in the calm air are presented. The air flow is described by the Navier–Stokes equations of axisymmetric stationary viscous flow of incompressible fluid that are numerically integrated by the computational fluid dynamics (CFD) software FLUENT. The collection efficiencies of RespiCon impactor stages agree quite well with experimental data and curves of the European standards for the thoracic and respirable dust fractions. The aspiration efficiencies derived from the numerical model overestimate the experimental data in the range of particle sizes of 10 μm < dp < 40 μm; however, they correctly predict the value of maximal size of aspirated particles. A new design of the RespiCon sampler with a higher volume flow rate was developed.
Copyright 2014 American Association for Aerosol Research
INTRODUCTION
One of the important problems in environmental science is the study of air quality in the context of human exposure in living and working environments. Aerosols, defining all types of suspended particles, represent an important class of air pollutants. Aerosol samplers of various shapes and sizes are routinely applied for aerosol measurements in the atmosphere and at workplaces. The sampling process in the real-life situation can be influenced by many factors and the measured concentration can differ significantly from the true one. To correct this discrepancy, aspiration efficiency is defined as the ratio of the particle concentration inside the sampler to the real aerosol concentration. A great number of experimental and theoretical investigations have been reported over the past few decades. A review of papers dedicated to the study of the sampling efficiency can be found in Vincent (Citation2007). The dependence of the aspiration efficiency of aerosol samplers on particle diameter matches the definition curve of the inhalable fraction of the airborne dust for workplace measurements.
At present, there is an ongoing interest in the indoor aerosol measurements which is caused by the fact that human health is greatly affected by inhalation of the indoor aerosol. In living and working environment, the air is usually still or moves with low velocity. In the study by Baldwin and Maynard (Citation1998), it was found that typical workplace wind velocities are much lower than the range 1.0–4.0 m/s used in establishing the criteria for measuring dust concentrations in the workplace and in fact they rarely exceed 20 cm/s. Calm air conditions are often used to analyze the performance of aerosol samplers for indoor measurements. The results of experimental studies of the aspiration efficiency for thin-walled and thick-walled tubes operating in still air were presented by Kaslow and Emrich (Citation1974), Gibson and Ogden (1977), Yoshida et al. (Citation1978), Belyaev and Kustov (Citation1980), Grinshpun et al. (Citation1989), and Su and Vincent (Citation2002). Mathematical models for thin-walled tube sampling in still air were developed by Agarwal and Liu (Citation1980) and Dunnett (Citation1992). The aspiration efficiency for the two-dimensional blunt sampler operating in calm air at horizontal and upward and downward vertical orientations was studied by Chung and Dunn-Rankin (Citation1993). Mathematical model and the results of studies of the spherical sampler operating in the calm air are given by Galeev and Zaripov (Citation2003) and Dunnett et al. (2006). The results of experimental studies on the inhalable fraction in slow moving air are given by Schmees et al. (Citation2008). A theoretical study of a thin-walled sampler in the environment with very low velocity was presented by Zaripov et al. (Citation2010).
The RespiCon sampler for concentration monitoring of aerosol particles is described in Koch et al. (Citation1999). As a single sampling device, it allows simultaneous collection of either personal or area samples of the respirable, thoracic, and inhalable fractions of airborne dust. The sizes of particles of these fractions are defined in the European and U.S. standards for health-related dust measurements at the workplace (CEN Citation1993; ACGIH Citation2007). The RespiCon sampler was developed primarily for workplace monitoring. Presently, its applications are also connected with epidemiological studies and particle size analysis.
The instrument is basically a two-stage virtual impactor containing three sampling filters. Airborne particles corresponding to the inhalable fraction are sucked into the RespiCon omnidirectionally through an annular slit (). The device operates at a flow rate of 3.1 l/min with an internal flow splitting of Q1 = 2.66 l/min in the first stage, Q2 = 0.33 l/min in the second stage, and Q3 = 0.11 l/min through the final filter. The diameters of the sending nozzles are 2.9 mm for the first stage and 2.5 mm for the second stage. For this configuration, the aerodynamic diameters of the 50%-value of the separation curves are 4.2 μm in the first stage and 10 μm in the second stage (Koch et al. Citation1999). The inlet velocity in the annular slit is 2.5 m/s.
The performance of RespiCon was experimentally studied by Koch et al. (Citation1999, Citation2002), Li et al. (Citation2000), and Feather and Chen (Citation2003). The latter publication addresses the sampling efficiency of the RespiCon under the calm-air conditions. This study showed an underestimation of the RespiCon's sampling efficiency compared to the definition curves starting at aerodynamic diameters of 15–20 μm. There was a final cutoff at about 65 μm. All other validation experiments reported earlier were carried out under moving-air conditions.
The scenario of sampling from calm air was also chosen for the present theoretical study of the RespiCon sampler to enable comparison with the aforementioned experimental findings. The calm-air conditions allow the axial symmetry of air flow to be used. The validated numerical procedure was then applied to study the aspiration and classification characteristics of a revised version of the RespiCon which operated at the flow rate of 6.2. l/min, i.e., twice the value of the standard version. The new version was built in order to reduce the detection limit for the gravimetric analysis of the airborne dust concentration.
Up till now, the aspiration efficiency and operating performance of the RespiCon sampler have not been analyzed theoretically. In this work, a mathematical model of the aerosol flow around and inside the RespiCon sampler operating in the calm air is developed. The air flow is described by the Navier–Stokes equations of axisymmetric stationary viscous incompressible flow. The Navier–Stokes equations are numerically integrated by the CFD software FLUENT. The particle trajectories are calculated by numerical integration of the equations of particle motion in the velocity field found from the solution of fluid flow equations. The aspiration efficiency of the sampler inlet and the collection efficiences of the impactor stages are calculated as a particle diameter function.
FORMULATION OF THE PROBLEM
In order to compare the available experimental data, a RespiCon sampler with a circular inlet head with radius Rs
and outer height H is considered (). The air is assumed to be stagnant far from the sampler orientated vertically and particles move in X-axis direction by settling with velocity (g is the gravity acceleration). Particle relaxation time τ is expressed as τ=d
2ρ
p
/18μ (d and ρ
p
are the particle diameter and density, respectively, and μ is the air viscosity). Due to axial symmetry, it is sufficient to consider only the half of the meridian plane (X,R) (X,R are the axial and radial coordinates). The X-axis coincides with the axis of symmetry of the sampler.
FIG. 2 (a) RespiCon sampler scheme. (b) Mesh of calculation domain outside the sampler. (c) Virtual impactor stage scheme.
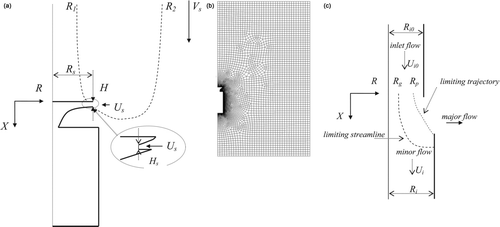
The circular slit inlet has a converging form with the narrowest width Hs. A fraction of the settled particles is aspirated by suction through the sampling inlet slit with the mean velocity Us (sampling velocity). By tracing the particle paths, it is possible to find the limiting particle surface separating the particles that are aspirated from those that are not ().
Let Sp be the horizontal area of the cross-section of the limiting particle trajectory surface far from the sampler. Due to the symmetry, the area of the circular annular region, Sp , can be expressed through the values of the initial radial coordinates, R 1 and R 2, of the inner and outer limiting trajectories, Sp =π(R 2 2−R 2 1). Using the known surface area, Sp , and flow rate through the inlet, Q=Us 2πRsHs , the aspiration efficiency A is calculated as
According to EquationEquation (1), calculation of the aspiration efficiency is reduced to that of the limiting particle trajectories by solving numerically the equations of particle motion in the given air velocity field.
By neglecting the influence of particles on the carrier phase due to their small concentration, the air velocity field is described by the system of Navier–Stokes equations of a laminar flow of an incompressible fluid

The equality of pressure and atmospheric pressure is taken as a boundary condition far from the sampler. The velocity no-slip conditions on the sampler walls and the symmetry conditions on the X-axis are used. The value of the sampling velocity Us is given at the inlet cross-section.
Under the assumption that the Stokes law of aerodynamic drag is valid, the equations of motion of aerosol particles are written in dimensionless form as follows:
By solving the Cauchy problem for EquationEquations (3) with the initial conditions at t = 0
To determine the collection efficiency Ev of the virtual impactor stages, the method of limiting particle trajectories is also applied (Marple and Chien Citation1980). The value of the initial ordinate Rp of the trajectory that limits the flux of particles moving with the major flow () is used to calculate Ev from the formula

NUMERICAL RESULTS
The RespiCon sampler has an inlet head radius Rs = 0.04 m and an outer height H = 0.003 m. Samples are taken at a flow rate of Q = 3.1 l/min through the annular slit and then passed to the first impactor stage. The sampling efficiency of the annular inlet is independent of the wind direction; thus, the instrument can also be used as a static aerosol monitor. In addition, annular slit inlets have the potential to follow the inhalable definition curve quite closely at least for particles smaller than 30 μm (Gao et al. Citation1997). The slit has a converging form with a narrowest width H s = 200 μm. The sampling velocity in the cross section of the slit is Us = 2.5 m/s for the flow rate Q = 3.1 l/min. Beyond the inlet slit, particles continue their accelerated curvilinear motion, reaching velocity of order 10 m/s. The centrifugal acceleration acting on the particles is larger than the gravitational acceleration, thus preventing even large particles from being deposited on the inner walls of the sampling inlet. Losses inside the sampling head are, therefore, expected to be small. The three internal flow rates Q1 , Q2 , and Q3 result in ratios of minor flow rate to total flow rate of the impaction stage of α1=0.143 in the first stage and of α2=0.25 in the second stage. The values of air velocity at the nozzle outlet of the impactor stages were calculated using the values of flow rates given earlier.
The Navier–Stokes equations are solved by the finite volume method using the CFD software FLUENT (version 6.3.26, Fluent Inc., NH, USA). The size of the calculation domain is to be so large that a further increase does not affect the numerical solution. The calculation domain was meshed by an unstructured triangular mesh (). Meshing provides the convergence of the numerical solution with the relative residual of 10−8. The obtained gas velocity field is used to solve EquationEquations (3) with initial conditions (4). To integrate the equations of particle motion, the axial and radial components of air velocity are determined by interpolating the velocity field obtained from the solution of the Navier–Stokes equations. Examples of particle trajectories in the vicinity of the inlet and on the first impactor stage are shown in . It can be seen that the particles which started close to the symmetry axis will impact on the outer wall of the sampler head (). The fraction of settling particles is sampled through the inlet. The values of the initial radial coordinates, R 1 and R 2, of the inner and outer trajectories that limit the trajectories of aspirated particles allow the aspiration efficiency to be calculated by formula (1).
FIG. 3 Examples of trajectories of particles with d = 4 μm in the vicinity of the (a) inlet and on the (b) impactor stage.
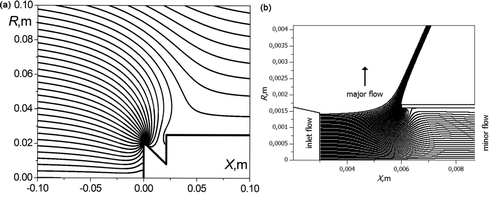
The inlet stage of the virtual impactor was modeled as a velocity inlet and both the minor and major flow channels were modeled as outflow. Simulation results of particle motion in the virtual impactor are shown in . Part of the particles that is separated from the minor flow is collected on the filter surface around the impactor minor tube. The value of the ordinate of the trajectory that limits the flux of particles moving with the major flow is used to calculate the impactor efficiency by formula (5).
It is known that during the sampling process aerosol particles can impact the sampler wall. Some of the particles impacted on the outer wall will bounce and enter the sampler depending on particle and wall properties or hydrodynamics conditions. “Primary” and “secondary” aspiration definitions are discussed, for example, by Grinshpun et al. (1990). Our calculations show that at the average value of the Stokes number, some of the particles impact on the inner wall of the slit (). After bouncing from the slit wall, these particles can penetrate into the sampler. Therefore, two types of aspiration efficiencies were calculated. The purely inertial aspiration efficiency Ai is determined using the initial ordinate of the limiting particle trajectory that hits the slit of the outlet edges. The overall aspiration efficiency Ae is calculated taking into account the rebound of particles from the inner wall of the slit (secondary aspiration).
The aspiration efficiencies Ai and Ae of the inlet and the collection efficiencies Ev of the impactor stages as a function of particle diameter are shown in . The experimental data from the investigations carried out by Koch et al. (Citation1999) are shown by symbols. Dashed curves correspond to the dust fraction defined by the European Standard 481 (CEN Citation1993). Aspiration efficiencies Ai and Ae overestimate the experimental data and inhalable fraction curve in the range of particle sizes 10 μm < dp < 40 μm; however, they correctly predict the value of maximal size of aspirated particles. The sampling efficiencies obtained experimentally and theoretically are below the inhalable fraction definition curve for dp > 50 μm. The difference between Ai and Ae appears in the range of particle sizes where the sampling efficiency decreases. The maximal size value of the sampled particles is larger for overall aspiration efficiency. The lower values of the experimentally found aspiration efficiency for dp = 13 μm and dp = 33 μm can be explained partially by particle loss on the inner surface of the inlet head and possible distortion by the calm-air conditions. These effects are increased with the increasing particle inertia. Therefore, the effect is most prominent for the large particles. Particle losses inside the RespiCon sampler under the moving-air conditions were studied by Li et al. (Citation2000).
The calculated collection efficiencies Ev as a function of particle diameter agree quite well with experimental data and conventional curves in the thoracic and respirable fraction ranges. The results of the present numerical studies and previous experiments confirm that the size classification by the virtual impactor of RespiCon enables separation of dust particles according to the thoracic and respirable defini- tions.
To extend the range of application of the RespiCon sampler toward outdoor and workplace measurements at conditions with low dust burden and low concentration standards, a new device with a higher sampling flow rate was designed. The nozzle sizes of the virtual impactor and the inlet slit were modified so as to account for the higher value of the flow rate. The width of the inlet was increased to maintain the same inlet velocity. The impactor nozzles were enlarged in order to keep the separation diameter the same as for the low-flow version. The working principle and all other geometrical dimensions were identical to the low-flow version of the RespiCon. The rescaled device was studied numerically by calculating the flow field and particle trajectories. shows a comparison of the numerical results of both RespiCon samplers. The cutoff sizes of the two virtual impactor stages practically coincides with those of the low-flow RespiCon. Since in the high-flow version, the inlet velocity at the inlet slit was kept constant by enlarging the width of the slit, the impaction Stokes number controlling particle losses in the inlet decreases at fixed values of particle diameter. Therefore, the cutoff curve is shifted toward larger values of particle diameter (). Overall, it can be seen that the new RespiCon enables sampling and classification of dust particles approximately in agreement with the definition of inhalable, thoracic, and respirable particles and can be used for measurements of aerosol concentrations in indoor workplaces at higher flow rates.
CONCLUSIONS
A mathematical model of the RespiCon sampler operating in the calm air has been developed. The air flow was described by the Navier–Stokes equations of axisymmetric stationary viscous flow of incompressible fluid that were numerically integrated by the CFD software FLUENT. Particle trajectories were determined by numerical solution of equations of particle motion in the calculated velocity field of the air flow. The aspiration efficiency of the sampler inlet and the collection efficiencies of the virtual impactor stages were calculated by the limiting trajectory method. It was shown that the obtained dependences of efficiencies on particle diameter agree well with experimental data and curves of the European standards of the thoracic and respirable dust fractions. The aspiration efficiencies determined by the numerical model overestimate the experimental data in the range of particle sizes 10 μm < dp < 40 μm; however, they correctly predict the value of maximal size of aspirated particles. A new design of the RespiCon sampler with a higher volume flow rate was developed. The numerical study of its performances has shown that the new RespiCon sampler provides the required separation of dust particles according to the definition of inhalable, thoracic, and respirable particles and can be used for the measurement of aerosol concentrations in indoor workplaces at higher flow rates.
Acknowledgments
This work was supported by DAAD (PKZ: A/09/03629-Ref.325) and RFBR (No. 12-01-00333).
REFERENCES
- Agarwal , J. K. and Liu , B. Y. H. 1980 . A Criterion for Accurate Aerosol Sampling in Calm Air . Am. Ind. Hyg. Assoc. J. , 41 : 191 – 197 .
- American Conference of Governmental Industrial Hygienists (ACGIH) . 2007 . Threshold Limit Values for Chemical Substances and Physical Agents and Biological Indices , Cincinnati , OH : ACGIH .
- Baldwin , P. E. J. and Maynard , A. D. 1998 . A Survey of Wind Speeds in Indoor Workplaces . Ann. Occup. Hyg. , 42 ( 5 ) : 303 – 313 .
- Belyaev , S. P. and Kustov , V. T. 1980 . Sampling from Calm Air . Trudy IEM , 25 : 102 – 108 . (in Russian)
- Chung , I. P. and Dunn-Rankin , D. 1993 . The Effects of Bluntness and Orientation on Two-Dimensional Samplers in Calm Air . Aerosol Sci. Technol. , 19 : 371 – 380 .
- Dunnett , S. J. 1992 . A Mathematical Study of the Sampling Characteristics of a Thin-Walled Sampler in Calm Air . Aerosol Sci. Technol. , 17 : 93 – 104 .
- Dunnett , S. J. , Wen , X. , Zaripov , S. K. , Galeev , R. S. and Vanunina , M. V. 2006 . A Numerical Study of Calm Air Sampling with a Blunt Sampler . Aerosol Sci. Technol , 40 : 477 – 489 .
- European Committee for Standardization (CEN) . 1993 . Workplace Atmospheres—Size Fraction Definitions for Measurement of Airborne Particle, CEN standard EN 481 , Brussels : CEN .
- Feather , G. A. and Chen , B. T. 2003 . Design and Use of a Settling Chamber for Sampler Evaluation Under Calm-Air Conditions . Aerosol Sci.Technol , 37 : 261 – 270 .
- Galeev , R. S. and Zaripov , S. K. 2003 . A Theoretical Study of Aerosol Sampling by an Idealized Spherical Sampler in Calm Air . J. Aerosol Sci. , 34 : 1135 – 1150 .
- Gao , P. , Dillion , H. K. and Farthing , W. E. 1997 . Development and Evaluation of an Inhalable Bioaerosol Manifold Sampler . Am. Ind. Hyg. Assoc. J. , 58 : 196 – 206 .
- Gibson , H. and Ogden , T. L. 1977 . Some Entry Efficiencies for Sharp-Edged Samplers in Calm Air . J. Aerosol Sci. , 8 : 361 – 365 .
- Grinshpun , S. A. , Lipatov , G. N. and Sutugin , A. G. 1990 . Sampling Errors in Cylindrical Nozzles . Aerosol Sci. Technol. , 12 : 716 – 740 .
- Grinshpun , S. A. , Lipatov , G. N. and Semonyuk , T. I. 1989 . A Study of Sampling of Aerosol Particles from Calm Air into Thin-Walled Cylindrical Probes . J. Aerosol Sci. , 20 : 1561 – 1564 .
- Kaslow , D. E. and Emrich , R. J. 1974 . Particle Sampling Efficiencies for an Aspirating Blunt Thick-Walled Tube in Calm Air , PA : Department of Physics, Lehigh Univerisity . Technical Report 25
- Koch , W. , Dunkhorst , W. and Lodding , H. 1999 . Design and Performance of a New Personal Aerosol Monitor . Aerosol Sci. Technol. , 31 : 231 – 246 .
- Koch , W. , Dunkhorst , W. , Lodding , H. , Thomassen , Y. , Skaugset , N. P. Nikanov , A. 2002 . Evaluation of the RespiCon as a Personal Inhalable Sampler in Industrial Environments . J. Environ. Monit. , 4 : 657 – 662 .
- Li , S.-N. , Lundgren , D. A. and Rovell-Rixx , D. 2000 . Evaluation of Six Inhalable Aerosol Samplers . AIHAJ , 61 : 506 – 516 .
- Marple , V. A. and Chien , C. M. 1980 . Virtual Impactors: A Theoretical Study . Environ. Sci. Technol. , 14 : 976 – 985 .
- Schmees , D. K. , Wu , Y. H. and Vincent , J. H. 2008 . Experimental Methods to Determine Inhalability and Personal Sampler Performance for Aerosols in Ultra-Low Windspeed Environments . J. Environ. Monit. , 10 : 1426 – 1436 .
- Su , W. C. and Vincent , J. H. 2002 . New Experimental Method to Directly Measure Aspiration Efficiencies of Aerosol Samplers in Calm Air . J. Aerosol Sci. , 33 : 103 – 118 .
- Vincent , J. 2007 . Aerosol Sampling: Science, Standards, Instrumentation and Applications , 616 John Wiley& Sons . , New York
- Yoshida , H. , Uragami , M. , Hasuda , H. and Iinoya , K. 1978 . Particle Sampling Efficiency in Still Air . Kagaku Kogaku Ronbunshu , 4 : 123 – 128 . (HSE translation N 8586)
- Zaripov , S. K. , Gilfanov , A. K. and Maklakov , D. V. 2010 . Numerical Study of Thin-Walled Sampler Performance for Aerosols in Low Windspeed Environments . Aerosol Sci. Technol. , 44 : 152 – 160 .