ABSTRACT
The interplay between canister, valve design, formulation, and environmental temperature is crucial to dose retention in metered dose inhalers (MDIs). Previous studies that have utilized MDIs with polymeric capillary retention valves, have shown that exposure to environmental changes can create a temporary temperature gradient between the formulation retained in the metering chamber and the formulation reservoir in the metal canister, which can cause inconsistencies in the dose delivered to the patient. The purpose of this study was to more fully quantify these effects. This was achieved by deliberately varying the temperature difference between inhalers and environment within ranges representative of routine usage, and assessing the resulting loss of prime effect via shot weight and delivered dose testing.
The shot weights delivered by three fixed-dose commercial MDIs—Foster®, flutiform® and Seretide®, were investigated under different experimental conditions. Exposure to temperature changes of up to 15°C did not appear to affect unprimed shot weights (USW) or subsequent doses from the Foster product. In contrast, flutiform maintained prime at a temperature differential of 8.6°C, but delivered a low USW following exposure to a ΔT of 15°C under both realistic and controlled conditions. Seretide exhibited loss of prime at lower temperature differentials (ΔT 8.6°C) and a reduction in USW. The results suggest that the inclusion of ethanol in a solution-based formulation may inhibit loss of prime, leading to more robust performance in the face of temperature variations.
Delivered dose testing was carried out to assess the effect of loss of prime on the device ability to deliver a dose to within 80–120% of the label claim. The results suggest that the drainage of propellant from the metering chamber of suspension MDIs leaves active pharmaceutical ingredient (API) residue, causing an increase in subsequent doses once the prime has been restored. Taken together, the results provide valuable insight into the likely performance of MDIs subjected to routine daily use, highlighting design and formulation strategies that could be applied to make performance more robust.
EDITOR:
1. Introduction
1.1. The use of capillary retention valves in metered dose inhalers (MDIs)
The performance of an MDI is quantified using metrics such as: shot weight, the weight of a single metered dose; delivered dose, the amount of formulation delivered by the device in a single actuation; and aerodynamic particle size distribution, the size of particles delivered to the patient. These parameters are dependent on the properties of the formulation and the design features of the device—the hardware employed. The vaporization characteristics of the formulation are particularly important since they influence atomization behavior, when the device is actuated. The phasing out of chlorofluorocarbons (CFC) propellants has necessitated extensive reformulation over recent decades with more environmentally friendly hydrofluorocarbons (HFC) now the propellant of choice for many products (Myrdal et al. Citation2014). Ensuring that the formulation is optimized in terms of, for example, surface tension and vapor pressure is crucial for effective dose delivery. Ethanol is routinely incorporated as a cosolvent to further improve performance.
The key hardware components of an MDI are: the canister, which holds a reservoir of formulation; the metering valve, which ensures that a consistent amount of formulation is delivered to the patient with each actuation; and the actuator mouthpiece, which atomizes the dose and provides an interface between the MDI and the patient. Significant innovations to MDI hardware have been introduced to address the requirements of new formulations and, more generally, to improve product performance with the metering valve; the focus of significant attention due to its pivotal role in ensuring consistent dose delivery (Stein et al. Citation2014). Newer metering valve designs are specified to, for example, promote faster filling/draining to directly address the issue of loss of prime.
Commercial MDIs typically make use of capillary retention valves (), to pre-meter the dose immediately following actuation, in readiness for subsequent use. Liquid formulation from the reservoir in the canister is driven through capillaries A and B and into the metering chamber, by the differential pressure across the valve stem orifice ports. Consistent delivery is essential for effective treatment and depends upon each dose volume being retained within the metering chamber by equilibrium capillary forces established at the capillary ports until release by subsequent MDI actuation (Lewis et al. Citation2011).
Figure 1. Illustrative schematic of Bespak BK 357 MDI capillary valve (diameters of capillaries A & B = 0.6 mm).
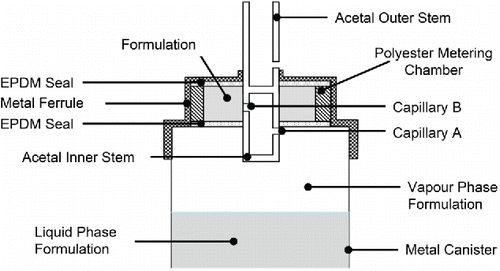
Although the scientific basis for utilizing capillary retention valves in this way is sound, the Rayleigh-Taylor theory suggests that if the orifice of capillary A approaches the critical wavelength (λcrit) for de-stabilization of the liquid vapor phase interface, then opposing gravitational force may exceed the capillary retention force (Sharp Citation1984; Fradley and Hodson Citation2008). This can cause drainage of the formulation from the metering chamber back into the canister resulting in erratic dosing unless the MDI is re-primed prior to use (Fradley and Hodson Citation2008; TheilCitation1996; Newman and Peart Citation2009) or consistently stored valve down.
Other factors have also been identified to cause or exacerbate the loss of prime in valve designs, as shown in . These include temperature fluctuation and MDI agitation, which can influence the balance of gravitational, interfacial and pressure differentials across the capillary channels within the valve stem, (Thiel Citation1996; Newman and Peart Citation2009; Church et al. Citation2011). Lewis et al. demonstrated that the materials selected for the canister and valve can play a crucial role in determining the impact of temperature fluctuations, for certain types of formulation. The combination of a high thermal conductivity metal canister with insulating polymeric valve components can facilitate the evolution of short-term thermal differences, causing the content of the metering chamber to develop a greater vapor pressure than that within the canister, resulting in drainage of the formulation from the metering chamber (Lewis et al. Citation2011).
A further study by Church et al. involving the construction of a 2D model, allowed the visualization of formulation drainage from a capillary retention system, during temperature fluctuations. The results showed that a temperature differential of just 1°C was sufficient to overcome surface tension forces and drain HFA 134a across the capillary interface. However, the addition of 15%w/w ethanol to the formulation impeded this drainage. This effect has been attributed to preferential evaporation of the volatile HFA at the liquid-vapor interface, associated ethanol enrichment and the inhibition of further HFA loss from the metering chamber (Gamayunov et al. Citation1984). As a result, drainage of the ethanol formulation from the metering chamber was observed to be very slow and only initiated when ΔT = 15°C, which is an unrealistic temperature differential to develop across two parts of the same MDI, regardless of external temperature influences (Church et al. Citation2011; Lewis et al. Citation2011).
As patients go about their daily activities and move through a variety of environments, the exposure of MDIs to temperature fluctuations is inevitable. When an MDI is exposed to higher environmental temperatures, the liquid formulation in the canister heats up more rapidly than the formulation in the thermal insulated metering chamber. The resulting vapor pressure difference may contribute to dose retention. Whereas, when an MDI is exposed to a cooler environment, the vapor pressure of the liquid formulation within the canister becomes lower than in the metering chamber, which can cause the pre-metered dose to drain back into the reservoir (Church et al. Citation2011; Lewis et al. Citation2011).
In this study, commercial MDIs with different formulations were tested to investigate dose retention following isothermal storage and temperature cycling. The aim was to assess the effect of any loss of prime associated with varying environmental conditions on the delivered dose. An additional aim was to assess whether the evaporation of the propellant from solution or suspension formulations could result in an increased concentration of active pharmaceutical ingredients (APIs) within the metering chamber, which could potentially be transferred to the next dose. The implications and relevance of the observations were evaluated in relation to real-world applications, and the potential impact on the patient.
2. Materials and methods
Experiments were carried out to investigate the response to temperature fluctuations of three fixed-dose commercial products; Foster® (Chiesi), flutiform® (Mundipharma), and Seretide® (GlaxoSmithKline). All of the products tested contained an inhaled corticosteroid (ICS) and a long-acting beta2 agonist (LABA) and had capillary retention valves, but differed with regards to their API(s), excipient and propellant content (). In addition, Foster is a solution formulation where the drug is homogenously mixed within the propellant, whereas flutiform and Seretide are suspension-based formulations.
Table 1. Summary of the container closure and formulation characteristics of the three commercial MDIs tested. Reproduced with permission from Lewis et al. (Citation2016).
Five units of each MDI product, all within their use-by-dates, were obtained from a pharmacy. Each product was subjected to five dose firings for each of the three different test schedules (n = 5). The products were actuated, according to guidance in the patient instruction leaflets (PILs), into dose unit sampling apparatus (DUSA), to establish baseline standard shot weights (SSWs). The DUSA were operated at a flow rate of 28.3 L/min, in accordance with test methods specified in the United States Pharmacopoeia (USP Citation2014). Prior to use, the MDIs were equilibrated to laboratory temperature and primed by firing the appropriate number of actuations through the device as stated in the PILs. Individual weights for two doses were averaged to give the SSW for each product.
Three experimental schedules were used to assess the effect of temperature variation on delivered shot weight, and each schedule involved cycling the MDI five times between two different environments. MDIs were stored for 30 min per cycle in the warmer environment, and 5 min in the cooler environment during both schedules. Time periods were selected to ensure equilibrium of the MDI (30 min; Lewis et al. Citation2011) prior to a short exposure (5 min) to the lower temperature. All MDIs were evaluated from first use (when the formulation mass in each canister was at a maximum) to minimize temperature and induced pressure gradients and ensure best-case dose retention scenarios. Furthermore, during experimental conditions, all MDIs were consistently stored valve upwards.
Schedule 1 involved cycling MDIs between an indoor environment at a higher temperature, and an indoor environment at a lower temperature. Schedule 2 involved cycling MDIs between an indoor environment at a higher temperature, and an outdoor environment at a lower temperature. Finally, in a further schedule MDIs were cycled under controlled conditions with the MDI stored valve-up for 30 min in an incubator at 20°C, followed by 30 min in an incubator at 5°C. As with schedule 1 and 2, this cycle was also repeated 5 times. In these controlled conditions, the storage period was 30 min for both temperatures to account for fluctuations introduced when incubator doors were opened in order to relocate the MDIs. Average temperatures for each environment are presented in .
Table 2. Temperature conditions for the environments used in all schedules (mean ± standard deviation, n = 5). Reproduced with permission from Lewis et al. (Citation2016).
Following the temperature cycle schedules, unprimed shot weights (USW) were measured for all MDIs. Dose retention values were then calculated as the percentage ratio of the USW to the associated SSW. In addition to evaluating shot weights, delivered dose analysis of both the ICS and LABA was carried out for five consecutive doses after storage. Unfortunately, LABA data was not determined for Seretide. Each dose was delivered into a separate clean DUSA, quantitatively rinsed and the API content determined by ultra-performance liquid chromatography (UPLC-MS) (ACQUITY UPLC System, Waters Limited, London, UK). To enable comparison between the products, these delivered dose values were calculated as a percentage of the reference label dose.
3. Results
3.1. Dose retention values
Exposure to temperature changes of up to 15°C did not appear to affect the initial unprimed USW/SSW or subsequent doses from the Foster product (, ). The performance of the flutiform product remained constant following Schedule 1 (ΔT = 8.6°C, ); where a USW/SSW of 99 ± 1% was recorded. However, when the temperature differential increased to 15°C, the USW/SSW fell to 46 ± 22% during Schedule 2 and 39 ± 40% during Schedule 3, respectively. For Seretide USW/SSW reduced to around 86% under the conditions imposed by Schedule 1, decreasing further to approximately 10% and 54%, respectively, when the temperature differential was increased (ΔT = 15°C), under realistic and controlled conditions.
Table 3. % dose retention (USW/SSW) following different temperature cycling schedules (mean ± standard deviation, n = 5). Reproduced with permission from Lewis et al. (Citation2016).
3.2. Delivered doses values
When the delivered dose of the API was measured, no significant difference was found for ICS dose delivery from the Foster solution formulation (t test, t ≥ 0.2); all doses were within 80–120% of the label claim. In contrast, the unprimed delivered ICS doses from the suspension based products; flutiform and Seretide were recorded as 52 ± 66% and 65 ± 38%, respectively, falling substantially below the label claim (). These values are slightly higher than the corresponding %USW/SSW (39 ± 40% and 54 ± 25%, respectively, see and ), suggesting that the API is left in the chamber after loss of prime. The LABA data obtained for Foster and flutiform followed the same trend. Delivered doses for flutiform and Seretide also took more than one shot to return to standard values (). The delivered dose values for the second shot after the Schedule 3 delivered a greater mass of ICS than the label claim: 135 ± 3% for flutiform and 120 ± 21% for Seretide.
Figure 4. Delivered dose of ICS and LABA following Schedule 3 as a % ratio of the standard delivered dose as specified on the product label (mean ± standard deviation, n = 5).
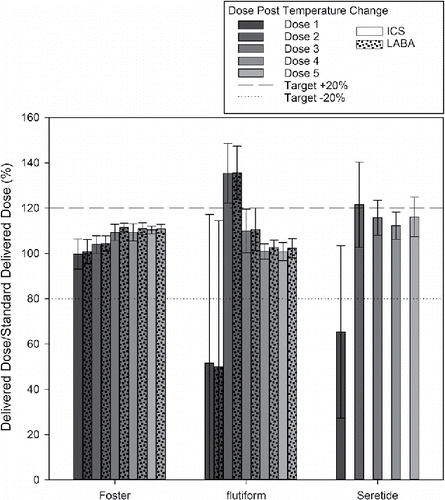
For flutiform and Seretide, ten and eight doses, respectively, fell outside of the 80–120% label claim. Furthermore, eight doses of the flutiform MDIs, and five of the Seretide MDIs fell outside of the 75%–125% label claim ().
Table 4. Number of ICS and LABA doses measured outside of label claim following controlled cycling (Schedule 3). Reproduced with permission from Lewis et al. (Citation2016).
4. Discussion
4.1. Dose retention values
Out of the three commercial products tested, the Foster product proved most robust to the impact of temperature cycling. Foster contains the most ethanol (12% w/w), which could be the reason for the observed stability. This is consistent with the results observed for the other commercial products. The flutiform formulation contains 1.3% w/w ethanol; which was sufficient to maintain the dose at a temperature difference of 8.6°C. However, under realistic and controlled conditions involving a temperature differential of 15°C, 1.3%w/w ethanol was not sufficient to prevent dose drainage, and consequently USW reduction. Seretide contains no ethanol within the formulation, and under all conditions, dose drainage was observed.
These results are in agreement with previous studies, suggesting that the inclusion of ethanol within an MDI formulation prevents temperature fluctuations from inducing dose drainage from the metering chamber. This is because evaporation of the propellant leads to ethanol enrichment at the metering chamber's capillary liquid-gas interface; inhibiting further hydrofluroalkane (HFA) evaporation, and facilitating dose retention (Lewis et al. Citation2011; Church et al. Citation2011).
The dose retention values measured after realistic and controlled temperature cycles were significantly different, suggesting that measuring dose retention under controlled conditions may underestimate the extent of dose drainage that occurs in a realistic situation. Clearly this reflects the importance of laboratory testing that reflects real-world performance.
4.2. Delivered doses values
The ICS and LABA doses delivered from the Foster solution formulation were consistent with the label claim, in accordance with the maintenance of prime. However, the unprimed delivered ICS doses from flutiform and Seretide fell short of the label claim. LABA data for flutiform suggested that delivery of the API was similarly compromised. These results are consistent with the loss of prime observed in the shot weight measurements, the delivered dose testing indicated a larger delivered dose than would be expected based on the loss of prime data alone. This suggests that due to evaporation the concentration of APIs in the formulation remaining in the metering chamber after loss of prime was higher than the concentration in the bulk formulation in the canister.
It is considered likely that formulation drainage from the metering chamber may leave behind API particles, a finding supported by this data. Re-suspending the API in the propellant remaining in the metering chamber before delivery of the unprimed dose, caused increased delivered dose values, which consequently were higher relative to their corresponding USWs. Furthermore, API particles which are not delivered may also re-suspend when the chamber is filled with the next and subsequent doses (Colombani et al. Citation2010). The results from this study support this hypothesis since the API delivered dose immediately following unprimed firing is substantially higher for both flutiform and Seretide, before falling back to the reference label value.
Following all schedules, the delivered dose values for flutiform and Seretide, fell outside the label claim (). The Food and Drug Administration guidelines recommend that no more than 1/10 delivered doses should be outside of the 80–120% label claim, for acceptable dose uniformity (FDA Draft Guidance Citation1998). The results highlight the necessity of priming doses to achieve acceptable product performance.
In this case the patient is instructed to fire up to four (flutiform) or two (Seretide) priming doses before taking their medication (120 dose label claim). Interestingly, the flutiform PIL recommends priming not only before using the inhaler for the first time or if the inhaler has not been used for three days or more, but also after exposing to freezing or refrigerated conditions (Flutiform Prescribing Conditions Citation2016). These recommendations are in agreement with the results obtained in this study. Seretide instructions for use do not provide specific repriming instructions following exposure to cold temperatures. However ICS/LABA combinations are intended to be taken regularly twice a day, and so dose retention issues may be much less of an issue (provided patients are compliant) when compared to sparingly used rescue inhalers that are constantly carried with the patient.
5. Conclusions
During normal daily life, the exposure of MDIs to modest temperature changes is inevitable. The results from this study show that transitioning between warm and cool environments can have a detrimental effect on dose drainage from the metering chambers of MDIs. Consequently, this can impact the dose of drug received by a patient.
Furthermore it was found that measurement of dose retention under controlled conditions may underestimate the extent of dose drainage that can occur in a realistic situation. This underlines the importance of carrying out appropriate testing in the laboratory to ensure that data is representative of what might be expected to occur during routine use, to avoid compromising the efficacy of treatment.
The loss of prime observed as a result of temperature cycling has further consequences than simply causing a reduced shot weight. For suspension formulations in particular, propellant drainage appears to leave behind API in the metering chamber, which can cause two problems: a reduction in the unprimed dose and/or an increase in subsequent doses, as remaining particles suspend in the re-filled chamber. It can take numerous shots to return to a dose that is consistent with the label claim.
A further conclusion is that the inclusion of ethanol in a solution formulation appears to provide some protection from the loss of prime caused by temperature cycling. This evidence, in combination with advances in device design, provides a basis for the future development of MDIs that more robustly answer to patient requirements and rely less heavily on instructions and appropriate use to deliver clinical efficacy.
Loss of dose retention can be solved by carefully considering the formulation and the closure system. Solution formulations contain ethanol to dissolve the API, and in some cases suspension formulations, such as Airomir® (3M) or Clenil®Compositum (Chiesi) also utilize moderate levels of ethanol as an excipient. Alternatively, metal container closure systems (canister and valve) can engender uniform metering chamber and canister thermal acclimatization. Finally, it is important to mention fast fill/fast drain valves, which are an alternative to the traditional capillary retention valve design, and they have the potential to eliminate loss of prime from MDIs (Topliss et al. Citation2006; Wilby et al. Citation2006). Although the designs have been available for many years, none have yet reached the US or European markets.
References
- Church, T.K., Hargrave, G.K., Johnson, R., Lewis, D.A., O'Shea, H., and Versteeg, H.K. (2011). Visualising Dose Retention: Fluctuation on a pMDI Capillary Retention Model. Respir. Drug Deliv., 317–320.
- Colombani, A.C., Chambers, F., Jansen, R., LeeK., and Hodson, D.J. (2010). Understanding the Cause of Increasing Dose Through Life from a Pressurized Metered Dose Inhaler (pMDI), in Respiratory Drug Delivery, R. N. Dalby, P. R. Byron, J. Peart, S. J. Farr, J. D. Suman, and P. M. Young, ed., Davies Healthcare International Publishing; River Grove, IL, pp. 425–430.
- Fradley, G., and Hodson, D. (2008). Optimisation of Fluid Flow in pMDI Valves, in Respiratory Drug Delivery, R. N. Dalby, P. R. Byron, J. Peart, S. J. Farr, J. D. Suman, and P. M. Young, ed., Davies Healthcare International Publishing, River Grove, IL, vol. 2, pp. 329–332.
- FDA Draft Guidance. (1998). Guidance for Industry (Draft). Metered dose inhaler (MDI) and dry powder inhaler (DPI) drug products: Chemistry, manufacturing, and controls documentation. Available at http://www.fda.gov/downloads/drugs/guidancecomplianceregulatoryinformation/guidances/ucm080584.pdf (Accessed 4 October 2016).
- Flutiform Prescribing Information. (2016). Accessed May 09 Available from http://www.flutiform.com/sites/default/files/FLUTIFORM_MDI_PIL_UK.pdf (Accessed 9 May 2016).
- Gamayunov, N.I., Uvarova, L.A., Malyshev, V.L., and Fel'dblyum, A. S. (1984). A Theory of Binary Liquid Mixture Evaporation from Capillaries. J. Eng. Phys. Thermophys., 46(3):317–321.
- Lewis, D.A., O'Shea, H., and Church, T.K. (2011). Prediciting HFA-MDI dose Retention Properties: Engineering the Marriage between Canisters, Valves and Formulations. Respir. Drug Deliv. Eur., 2011(1):89–99.
- Lewis, D. A., O'Shea, H., and Mason, F. (2016). Extending Metered Dose Inhaler Valve Prime Theory: The Effects of Formulation and Temperature Shock, in Respiratory Drug Delivery Europe, R. N. Dalby, P. R. Byron, J. Peart, S. J. Farr, J. D. Suman, P. M. Young, and D. Traini, eds., DHI Publishing, River Grove IL, pp. 71–78.
- Myrdal, P. B., Sheth, P., and Stein, S. W. (2014). Advances in Metered dose Inhaler Technology: Formulation Development. AAPS Pharm. Sci. Tech., 15(2):434–455.
- Newman, S., and Peart, J. (2009). Pressurized Metered dose Inhalers, in Respiratory Drug Delivery: Essential Theory and Practice, S. Newman, ed., Davies Healthcare International Publishing, River Grove, IL, Chapter 6.
- Sharp, D.H. (1984). An Overview of Rayleigh-Taylor Instability. Physica D, 12:3–18.
- Stein, S., Sheth, P., Hodson, P.D., and Myrdal, P. (2014). Advances in Metered dose Inhaler Technology: Hardware Development. AAPS Pharm. Sci. Tech., 15(2):326–338.
- Thiel, C.G. (1996). From Susie's Question to CFC Free: An Inventors Perspective of Forty Years of MDI Development and Regulation, in Respiratory Drug Delivery V, R. N. Dalby, P R. Byron, and S. J. Farr, eds., Interpharm Press, Buffalo Grove, IL, pp.115–124.
- Topliss, P., Ward, D., Southall, J.P., and Purewal, T.S. (2006). Pharmaceutical Performance of a Valve for Metered dose Inhalers (MDIs) Designed to Eliminate Loss of prime. Respiratory Drug Delivery, R.N. Dalby, P.R. Byron, J. Peart, S.J. Farr, and J.D. Suman, eds., DHI Publishing, River Grove, IL, pp. 499–502.
- United States Pharmacopoeia. (2014). USP 37/NF32 Chapter 601: Inhalation and Nasal Drug Products: Aerosols, Sprays and Powders—Performance Quality Tests. USP Convention, Rockville, MD.
- Wilby, M., Purkiss, G., Tilley, K., and Fradley, G. (2006). Novel Valve Designs to Eliminate Loss of Prime, In Respiratory Drug Delivery, R.N. Dalby, P.R. Byron, J. Peart, S.J. Farr, and J.D. Suman, eds., DHI Publishing, River Grove, IL, pp. 373–376.