ABSTRACT
Aerosol instrumentation based on unipolar diffusion charging has become popular in the recent years. These instruments can be made very small, making them suitable as personal monitors. In many applications, including personal monitoring, the use of flexible sampling tubes is required. We found that degassing from these sampling tubes can alter the gas composition of the aerosol, which changes the ion properties in the unipolar charger. As a result, the particle concentrations, measured with a unipolar diffusion charger are biased. The strongest effect was found with new, conductive silicone tubes, because of the degassing of siloxanes. Results obtained with one miniature diffusion size classifier unit were by a factor of approximately two too low. Partector and Nanoparticle Surface Area Monitor showed in principle the same behavior, but to a lower degree. Other tube materials were found to have even the opposite effect, i.e., the measured concentrations increased, when measuring through a tube. The largest observed increase was, however, only approximately 14%. Tygon™ tubes were found to be the best compromise considering particle losses and effect on the diffusion charger.
Copyright © 2016 American Association for Aerosol Research
EDITOR:
Introduction
Most applications of aerosol measurement techniques require the use of sampling lines to transport the aerosol from the point of measurement to the instrument. Transport of aerosols inevitably results in particle losses (Brockmann Citation2011; Tsai Citation2015) mainly due to gravitational settling (Thomas 1958), Brownian motion (diffusion losses, Gormley and Kennedy Citation1949), inertial deposition (Pui et al. Citation1987), and electrostatic losses (Liu et al. Citation1985). While mechanical losses can be minimized by using vertical sampling lines without bends and by reducing the residence time of the particles in the sampling tubes, electrostatic losses additionally depend on the tube material. Electrostatic losses arise if charged particles are exposed to an electric field inside the sampling tube. Such electric fields may stem from image charges, space charges, or from charged tube material. Liu et al. (Citation1985) showed that the effect of image and space charges on particle penetration through tubes is negligible, whereas charge islands in flexible plastic tubes can cause significant losses of charged particles. They showed that these losses are particularly pronounced in case of polyethylene and polytetrafluoroethylene (PTFE, e.g., Teflon™) tubing, whereas electrostatic losses in polyvinylchloride (PVC, e.g., Tygon™) were rather low. Electrically conductive and ideally grounded sampling lines are recommended to fully avoid electric fields inside the tubing (Brockmann Citation2011). In case of fixed laboratory installations, grounded copper or stainless steel tubes are often used. For mobile measurements, e.g., of the personal exposure to airborne particles, the tubes have to be flexible. Many aerosol instrumentation manufacturers therefore offer conductive flexible tubing. These tubes are made from silicone mixed with carbon black to increase the conductivity. Such tubes have been available for many years and are routinely used in aerosol measurements. Scanning mobility particle sizers (SMPS; Wang and Flagan Citation1990) are usually equipped with such tubes to transport the aerosol and sheath flow and to connect the differential mobility analyzer (DMA) with the condensation particle counter (CPC). While these tubes enhance the penetration of particles, they can also introduce artifacts, which in some cases bias the measurement. Yu et al. (Citation2009) reported that chemical analysis of particles sampled through conductive silicone tubing consistently revealed elevated siloxane levels. The amount of siloxane detected by gas chromatography-mass spectrometry (GC-MS) increased with increasing sampling tube length and could therefore be linked to degassing from the silicone tube. Siloxanes are functional groups in organosilicon chemistry. Polymerized siloxanes are also called silicone. Siloxanes are abundant in indoor air, stemming from, e.g., personal care products, sealants, etc. They may also degas from silicone, e.g., in case of incomplete polymerization. Yu et al.'s finding was confirmed by Timko et al. (Citation2009) who reported increased levels of particle bound polydimethylsiloxane (PDMS) on filter samples collected from aircraft engine exhaust. One meter of their in total 20 m long sampling train consisted of conductive silicone tubing which—due to the proximity to the engine—got moderately heated up to temperatures of up to 70°C. They found that besides a change in the chemical composition of the particles, siloxane vapor uptake also affected surface properties of the particles, namely, their affinity to water. They furthermore concluded that care should be taken when using silicone tubing for particle sampling on filters, because also the particle mass may be biased.
Nashimoto (Citation1989) reported about the buildup of a silicon oxide layer on top of wire electrodes inside photocopy machines in the presence of PDMS, where a positive corona discharge is used to charge toner particles. Davidson and McKinney (Citation1998) made a similar observation in electrostatic room air cleaners, using a positive corona discharge to charge particles that subsequently are electrostatically removed from the air stream. They concluded that the positive corona enhances the vapor deposition of siloxanes onto the corona electrode. The formation of silicon oxide layers has also been observed in aerosol measurement instruments using unipolar diffusion chargers. These chargers typically apply a positive high voltage between a thin wire or sharp tip and a counter-electrode to create a corona that generates air ions to charge particles. After operation for some time, the corona electrodes of these instruments are often found to be covered by a white silicon oxide layer. The layer reduces the electrical field strength at the surface of the electrode, thus reducing the ion generation and the particle charging efficiency. Commonly the instruments are designed to maintain a constant ion concentration (measured as the ion current) by adjusting the corona voltage. shows the time series of the corona voltage of the unipolar diffusion charger of two miniature diffusion size classifiers (miniDiSC; Fierz et al. Citation2011) one without tubing (straight line) and the other connected to a 5 m long conductive silicone tubing (broken line). The measurements were conducted over approximately 50 h. The short-lived spikes in the corona voltage result from the periodic zeroing of the downstream electrometer. It can be clearly seen that the corona voltage increased over time when the tube was connected. The slight decrease over time with disconnected tube was caused by the concurrent decrease in ambient air pressure (see inlay in ). The mean free path of the electrons in the corona region is inversely proportional to the gas pressure, so that they gain more energy between collisions with gas molecules and are more likely to contribute to the electron avalanche—therefore, the corona voltage for a given ion production rate is reduced at the lower gas pressure. The increase of corona voltage with a tube connected was not reversible by removing the tube. Only cleaning the corona electrode resulted in a decrease of the corona voltage. In order to reduce the cleaning/maintenance effort, some manufacturers therefore recommend not to use silicone tubing with their instruments (Fierz Citation2016).
Figure 1. Effect of the use of silicone tubing on the corona voltage of a unipolar diffusion charger.
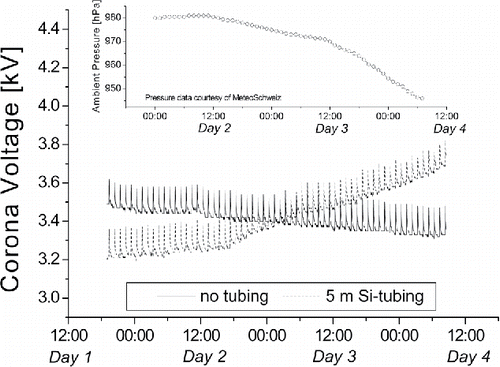
A number of aerosol instruments are available that use unipolar diffusion charging. These can be differentiated into instruments measuring the total current to infer size integrated particle concentrations and instruments that classify the particles to determine particle size distributions. The first group of instruments includes the DiSCmini (Testo AG, Titisee-Neustadt, Germany; essentially identical with miniDiSC), Partector (naneos particle solutions GmbH, Windisch, Switzerland; Fierz et al. Citation2014), nanoTracer (oxility B.V., Eindhoven, The Netherlands; Marra et al. Citation2010), and Nanoparticle Surface Area Monitor (NSAM, TSI model 3550 and Aerotrak 9000 [TSI Inc., Shoreview, MN, USA], Fissan et al. Citation2007; Shin et al. Citation2007). These instruments can all be used to measure the lung deposited surface area (LDSA) concentration with some limitations (Asbach et al. Citation2009). DiSCmini and nanoTracer additionally determine the number concentration and mean particle size. The second group of instruments includes nanoScan (TSI model 3910; Tritscher et al. Citation2013; Stabile et al. Citation2014), nanoID (PMS-Nanuem, Canterbury, UK, model NPS500), and the Fast Mobility Particle Sizer (FMPS, TSI model 3091) that all determine the number size distribution based on electrical mobility analysis as well as the Electrical Low Pressure Impactor (ELPI, Dekati, Tampere, Finland; Keskinen et al. Citation1992) that classifies particles based on inertia in a cascade impactor and determines the concentration per size class from the particle-induced current per impactor stage.
In a recent study on the field applicability of personal monitors based on diffusion charging (Asbach et al. Citation2015), we noticed significant underreporting of concentrations measured by DiSCmini when they were sampling through 75 cm long flexible, conductive silicone tubing (purchased from TSI GmbH, Aachen). In some cases, the deviation of the LDSA concentration reached a factor of three. The displayed concentration dropped almost instantaneously when the tube was connected and increased back to normal when it was disconnected. When the DiSCmini sampled directly through the inlet without a tube, no major deviations were observed. In this previous study, we furthermore noticed that the corona voltage showed a reversible 10 to 15% increase, when the tube was connected. These sudden bidirectional changes are different from the prior observations of a steady and irreversible increase of the corona voltage during sampling through silicone tubing. We assumed that this obvious artifact stems from a change in the charging efficiency and hypothesized that the known degassing of siloxanes from the silicone tubing affects the gas composition and the resulting ion species in the unipolar charger. Maißer et al. (Citation2015) investigated the mass mobility distribution of ions in air produced by a 210Po bipolar neutralizer. They found that different siloxanes with nominal masses between 371 Da and 593 Da and mobilities between 1.04 cm2/Vs and 1.27 cm2/Vs, respectively, were present in air samples, despite their use of highly pure artificial air. They concluded that the siloxanes stem from degassing system components like o-rings. The reported mass and mobility of the siloxanes are significantly different from the values typically assumed for the prediction of charging efficiencies, which usually range from 109 to 150 Da and 1.2 to 1.4 cm2/Vs (Pui et al. Citation1988). As a result they found clear deviations of the bipolar charge distribution from the Wiedensohler approximation (Wiedensohler Citation1988). An effect of siloxanes on unipolar charging is therefore to be expected, particularly when using silicone tubing for which rather strong degassing of siloxanes has previously been observed (Yu et al. Citation2009; Timko et al. Citation2009). We here present a systematic study on the effect of different types of tubing on the results produced by miniDiSC (Fachhochschule Nordwestschweiz, meanwhile commercialized as DiSCmini by Testo AG; Fierz et al. Citation2011), Partector (naneos particle solutions GmbH, Fierz et al. Citation2014), and NSAM (TSI model 3550; Fissan et al. Citation2007; Shin et al. Citation2007).
Experimental
Study on the effect of different tubes on diffusion charger instruments
Experiments were conducted in a 30 m3 test chamber, which is usually used for testing indoor air cleaners (Finger et al. Citation2015) according to the Chinese standard GB/T 18801-2008. A sodium chloride aerosol was produced by dispersing and drying a solution of 1 g/l NaCl in deionized water. The NaCl aerosol was produced outside the chamber, neutralized and fed into the room via tubing. A household fan inside the room homogeneously distributed the aerosol inside the chamber. No other ventilation was active during the experiments. Similarly to the process of testing indoor air cleaners according to the Chinese standard, the aerosol was fed into the chamber until a number concentration of approximately 1.5 *105 l/cm3 was reached. A table was placed in the middle of the chamber, which hosted the tested and the monitoring instruments. Besides the table with the instruments, a chair, and the fan the room was empty. The tested instruments include two miniDiSC (sample flow rate 1 l/min), three Partector (flow rate 0.5 l/min), and one NSAM (flow rate 2.5 l/min). An SMPS (TSI model 3936 with long DMA and UCPC model 3776) and a water-based CPC (TSI model 3787) were employed to monitor the evolution of the test aerosol. miniDiSC and Partector use in principle very similar charger designs, where the aerosol flow is separated from the corona wire electrode by a thin wire mesh. The charger operation is, however, different. The ion current in miniDiSC is 10 nA and thus higher than in Partector (2 nA). Additionally, the Partector charger gets switched on and off with a frequency of 0.5 Hz. The latter facilitates the current measurement principle of Partector based on induced currents (Fierz et al. Citation2014). NSAM uses a different charger design in which the total incoming flow is split into an aerosol flow and an ion jet flow (Medved et al. Citation2000). The ion jet flow gets filtered by a HEPA and an activated carbon filter before it passes a corona needle facing a nozzle to convectively transport the ions into a mixing chamber, where the ion jet flow and the aerosol flow are reunited.
The experimental set up was chosen since it is known that the aerosol is homogenously distributed within the chamber and therefore allows for simultaneous sampling of the same aerosol by all instruments with and without tubing. In the beginning, the test aerosol had a mean diameter of 80 nm and a number concentration of 1.5*105 l/cm3. Over the approximately 2 h sequence of an experimental run, the size distribution shifted to 115 nm and 40,000 l/cm3 mainly due to particle losses and agglomeration inside the chamber. The change of size and concentration were slow, smooth, and according to an exponential function. The charging efficiency of a unipolar charger is mainly dependent on the combination coefficients β, which are a function of the ionic flow to the particle surface and the ion concentration. For a given particle size, the ionic flow depends on the ion properties and the particle size, but not on the particle concentration, as long as the ion number concentration is much higher than the particle number concentration. The unipolar diffusion chargers used here are designed to have ion concentrations that are several orders of magnitude larger than the particle number concentrations. The particle size furthermore only negligibly changed during the 5 min measurement cycles with and without tubing, so that no extra activities were considered necessary to stabilize the size distribution.
According to the test sequence, each miniDiSC and the NSAM measured alternately 5 min with and 5 min without tube. As three Partector were available for the tests, for these devices the test sequence consisted of 5 min measurement with and 10 min without tube. The tubes were switched between the instruments by an operator who remained within the chamber for the entire test sequence. The measurements were repeated three times. LDSA concentrations were recorded with all instruments, whereas only the miniDiSC allowed for the measurements of also number concentrations and mean particle size. Only the Partector and miniDiSC software record the corona charger voltage. Seven different tubes were used in the study, i.e., a new and an aged conductive silicone tubing purchased through TSI GmbH, Aachen, Germany (inner diameter ID = 0.19” = 4.83 mm), a new and an aged conductive silicone tubing purchased through Grimm Aerosol GmbH, Ainring, Germany (ID = 6 mm), polyvinylchloride (Tygon™) tubing (ID = 4.8 mm), a silicone-free conductive tube typically used for spray varnishing applications (ID = 6 mm¸ Solvykler, Trelleborg, Sweden), and a non-flexible stainless steel tube (ID = 2.9 mm). Both aged tubes had been previously used for more than 20 h during different measurements at normal temperature and pressure conditions. The Grimm and TSI silicone tubes appear very similar, but differ in their softness. The Grimm tubing is softer, thus likely containing more phthalates than the TSI tubing. The exact same 75 cm long tubes were used for all instruments. 75 cm was chosen for the tube length as this corresponds to our previous measurements (Asbach et al. Citation2015) and is a typical tube length for personal exposure measurements, when the instrument is carried on a belt. Note that the residence time of the sampled aerosols inside the tubes differed due to the different sample flow rates of the instruments.
Study on the effect of different tubes on the gas composition of the sample aerosol
Gas chromatography–mass spectrometry (GC–MS) measurements were conducted to study a possible effect of degassing from the different tubing on the gas composition, which could affect the generation of ions in a unipolar corona charger. Air samples were collected on Tenax tubes. 178 mm long glass tubes (Gerstel GmbH, Mülheim an der Ruhr, Germany) with an inner diameter of 4 mm were packed according to the manufacturer's instructions with approximately 180 mg of Tenax TA, resulting in a packed bed of approximately 60 mm thickness. Tenax TA was chosen as it is known to adsorb larger molecules like siloxanes with very high efficiency. Room air was sampled at a flow rate of 250 ml/min for 4 min through each of the different tubes, connected to the Tenax glass tubes. Only the new flexible tubes were tested. The exact same 75 cm long sample tubes as used for studying the effect on the monitors were used for the air sampling. In addition, room air was sampled through one Tenax tube without a sample tube attached and used as reference. Upon sampling, the Tenax glass tubes were installed in an auto sampler (TDSA 2, Gerstel GmbH, Mülheim an der Ruhr, Germany), the adsorbed molecules desorbed from the Tenax in a thermo desorber (TDS 3, Gerstel GmbH), and re-focused in a cryo-trap, maintained at 10°C. The thermo desorber was initially at a temperature of 30°C that was increased at a rate of 60°C/min to 310°C, where it remained for 5 min. After the termination of the temperature profile of the thermo desorber, the retained molecules were guided from the cryotrap to the 60 m long fused silica GC column with an inner diameter of 0.320 mm (Optima 5, Macherey-Nagel GmbH & Co. KG, Düren, Germany) with 1 µm layer thickness. The GC oven (GC 6890N, Agilent Technologies Inc., Santa Clara, CA, USA) was initially maintained at a temperature of 40°C for 2 min, then heated up to a temperature of 300°C at a rate of 6°C/min and maintained at 300°C for another 10 min. The temperature profiles of both the thermo desorber and the GC oven followed established internal IUTA protocols. The chromatograms were finally recorded with a mass spectrometer (model 5973, Agilent Technologies Inc.).
Results and discussion
Study on the effect of different tubes on diffusion charger instruments
In order to estimate the influence of the different tubing on the instruments' response the test aerosol was sampled for 5 min, 10 min, respectively, in the case of Partector, without any tubing followed by 5 min measurement with tubing. As attaching/detaching the tubing might have a short-lived influence on the values reported by the instruments, e.g., because of pressure changes, the first and last 30 s of each measurement were not considered in the data evaluation. The values reported by the different instruments for the remaining 4 min were compared with the values that the instrument would have reported without the tubing. These values were determined by fitting the results from measurements without tubing to an exponential function (). Based on this function the expected values for the measurements without tubing were extrapolated.
Figure 2. Evolution of the LDSA concentration measured during an experimental run with and without tubing attached.
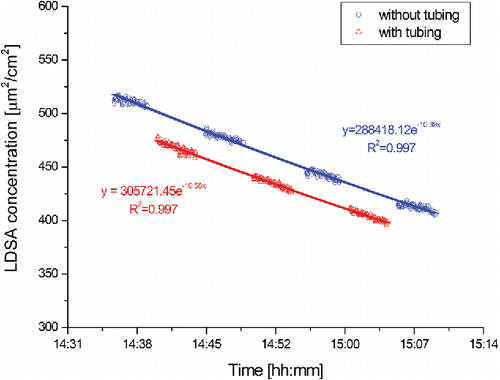
The seven different tubes had different effects on the instruments' responses. The TSI tubing lead in general to a decrease of the reported LDSA values by the instruments, coupled with an increase of the corona charger's voltage. As for NSAM the corresponding values for the corona charger's voltage are not recorded, only the influence on the reported LDSA values could be observed. shows the deviation of the LDSA concentration and corona charger voltage measured with and without new and aged TSI tubing. Errors bars indicate the minimum and maximum values. It can be seen that from the three types of instruments tested sampling through the TSI tubing had the highest influence on the miniDiSCs' response. Sampling through the new TSI tubing lead to an underestimation of the LDSA values by ∼50% by miniDiSC 1 and ∼30% by miniDiSC 2 that was associated with an increase of the corona charger voltage with ∼12% for both miniDiSCs. The absolute value of the corona voltages were 4.66 kV in case of miniDiSC 1 and 3.89 kV in case of miniDiSC 2. The corona voltage is device specific and is furthermore affected by the different age and cleanliness of the corona wire. Also the mechanical tension of the corona wire can affect the corona voltage. A tensely mounted wire will stay in place and generate a homogenous electric field and current density distribution along the wire, whereas a loosely mounted wire can form dents that cause inhomogeneities. These differences can result in different ionization and particle charging in the charger. Sampling through the aged TSI tube, previously used for approximately 20 h during different measurements, lead also to an underestimation of the LDSA concentration, but to a lower degree, ∼25% in the case of miniDiSC 1 and ∼10% for miniDiSC 2. The same trend was observed in the case of the corona charger voltage that increased only by ∼5% for miniDiSC 1, and ∼4% for miniDiSC 2, respectively.
Figure 3. Deviation of the LDSA concentration and the corona charger voltage, measured with and without new (left) and aged (right) TSI conductive silicone tubing.
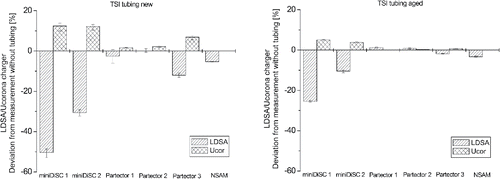
The Partectors qualitatively showed the same trend but were quantitatively less affected by the sampling manner, with or without TSI tubing. It should be noted that although their charger geometries are very similar, the Partector and the miniDiSC use a different charger concept. While the miniDiSC operates at a constant voltage and a charger current of 10 nA, the Partector periodically switches the charger on and off with a frequency of 0.5 Hz and operates at a lower current of 2 nA. The three tested specimens behaved slightly different. While the influence of the tubing on the LDSA values reported by Partector 1 and 2 was marginal, Partector 3 underestimated the LDSA concentration when sampling through both new (by ∼12%) and aged TSI tubing (by ∼2%), but to a much lesser extent than the two miniDiSCs. Also the effect on the corona charger voltage was larger for Partector 3 than for the other two, showing an increase up to ∼7% when sampling through the new TSI tube. The corona voltages of the Partectors were 3.9 kV (Partector 1), 3.85 kV (Partector 2), and 3.98 kV (Partector 3). Hence, like in the case of the miniDiSC, the effect was higher for higher corona voltages. It therefore seems that the influence of the tubes on the measured particle concentration is higher in case of higher corona voltages, which may cause a more efficient ionization of the siloxanes.
Also NSAM underestimated the LDSA values when sampling through both new (by ∼5%) and aged (by ∼3%) TSI tubing. Considering the design of the corona charger used in the NSAM, the influence of the tubing on the reported values was somehow surprising, as contrary to miniDiSC and Partector in the case of NSAM the aerosol flow does not come in direct contact with the corona needle. In the NSAM charger, the 2.5 l/min sample flow is split into a 1.5 l/min aerosol flow and 1 l/min charger flow, which is passed through an activated carbon filter that is expected to eliminate the present siloxanes and a HEPA filter before passing the corona needle to convectively transport the generated ions into a mixing chamber, where they mix with the aerosol flow (Medved et al. Citation2000). The observed effects may be explained by a decrease of the carbon filter's efficiency in eliminating these siloxanes due to the aging of the former. To confirm this theory, the carbon filter, which had been in use for more than 4 years, was exchanged and the measurements with both new and aged TSI tubing were repeated. The results, however, remained the same. It may hence be that the siloxanes not only affect the ionization process but also the charging in the mixing chamber where they can still enter with the aerosol flow. Another reason for this unexpected influence may be that the activated carbon is not sufficiently efficient in the removal of degassed siloxanes.
The behavior of the instruments when sampling through the new and aged conductive tubing purchased through Grimm Aerosol was also unexpected, considering that this is also, as the TSI tube, made from silicone. Contrary to the measurements with the TSI tubing all instruments overestimated the LDSA concentration when sampling through the Grimm tube, coupled with a decrease of the corona charger voltage, with the exception of Partector 3. The effect on the instruments' response was lower than in the case of TSI tubing and again more pronounced for the two miniDiSCs with LDSA increases of approximately 14% and 5%, respectively, and a change of the corona voltage by approximately −3.5% in case of new Grimm tubing. This effect was noticeably reduced in case of aged Grimm tubing (). The effect of both new and aged Grimm tubing on the LDSA measurement of the three tested Partectors was much lower and only around ±2%, which is within the measurement uncertainty. The reversed effect of the Grimm tubing compared with the TSI tubing cannot be explained by the presence of large ionized siloxanes in the charger. To the contrary, this effect may be caused by the presence of very small and highly mobile ions, stemming from very small degassing molecules. This will be further discussed in the next section.
Figure 4. Deviation of the LDSA concentration and the corona charger voltage, measured with and without new (left) and aged (right) Grimm conductive silicone tubing.
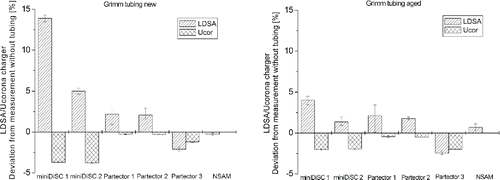
Furthermore, the response of the three types of instruments when sampling though silicone-free tubing, Tygon and stainless steel tubing was investigated, see . When sampling through both silicone free and Tygon tubing for all instruments a minor decrease in the corona charger voltage was observed, combined with an overestimation or underestimation of the LDSA values. With the exception of miniDiSC 1 that overestimated the LDSA by ∼6% when sampling through Tygon tubing, for all other instruments the deviations were quite small. Expectedly, the stainless steel tubing showed the lowest deviations, which are within the error margin of the measurements.
Figure 5. Deviation of the LDSA concentration and the corona charger voltage, measured with and without Tygon (top left), Si free (top right), and stainless steel (bottom) tubing.
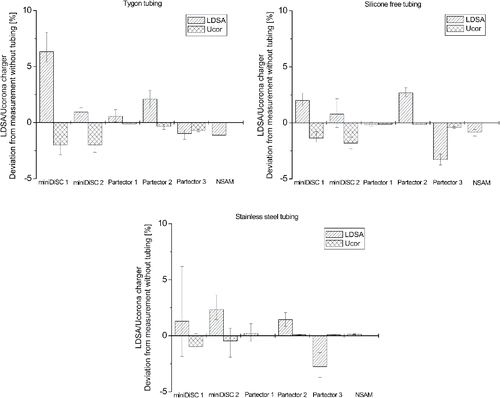
The effect of the tube materials on the measurements of the number concentration and mean particle size by miniDiSC are shown in . It can be seen that the effect of the different tubes on the measurement of the number concentration is qualitatively and quantitatively comparable to the effect on the LDSA concentration measurement with miniDiSC. The influence is highest and results in underestimation of the concentration in case of the new TSI tubing (−49% for miniDiSC 1 and −30% for miniDiSC 2), followed by the aged TSI tubing (−23% for miniDiSC 1 and −11% for miniDiSC 2). The number concentration is negligibly to moderately overestimated in case of all other tube materials. Only in the case of the new Grimm tubing the overestimation is considerable and around +12% for miniDiSC 1. The effect on the mean particle size is small for all tubes and deviations are within approximately ±2.5% for miniDiSC 1 and below ±1% for miniDiSC 2.
Study on the effect of different tubes on the gas composition of the sample aerosol
In order to better understand what processes might be responsible for the observed deviations in the particle charging, we studied the gas composition by GC-MS. Initially, the GC-MS was calibrated with a siloxane standard to quantify six different siloxanes with molar masses between 222.46 g/mol and 384.84 g/mol. Additionally, two larger siloxane molecules could be detected, but could not be quantitatively determined. A list of all covered siloxanes is given in .
Table 1. List and properties of all covered siloxanes.
Blank values, measured by thermal desorption of the gas phase collected on Tenax TA tubes without tubes attached showed no indication for the presence of siloxanes. Out of the collected samples, only those collected through new TSI tubing revealed high levels of siloxanes, as shown in , including a very high peak for the large dodecamethylcyclohexasiloxane with a molar mass of 444.92 g/mol.
Figure 7. Chromatogram of the siloxanes in the gas phase collected with new TSI silicone tubing; numbers in the figure refer to IDs in .
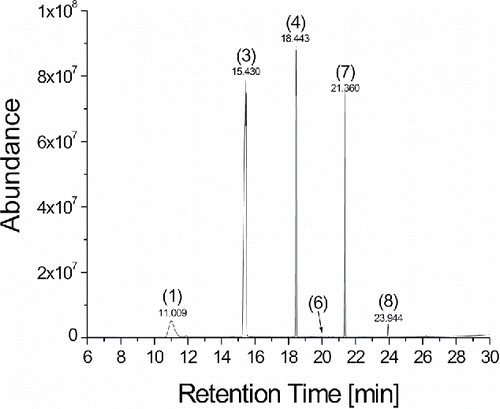
The detected siloxane concentrations for all three tubes are summarized in . The main intention of this study was to verify the presence of siloxanes, hence only the new flexible tubes were used in the experiments. While the Grimm and Tygon tubing showed the presence of some siloxanes, their concentrations were below the detection limit and could thus not be quantified. To the contrary, the concentrations of hexamethylcyclotrisiloxane and octamethylcyclotetrasiloxane measured for the samples collected with TSI tubing were too high to be quantified with this method. Furthermore, a high peak of the large dodecamethylcyclohexasiloxane molecule was detected for the TSI tube. The results prove that high amounts of siloxanes degas only from the TSI tubing. This supports the hypothesis that the presence of high siloxane concentrations in the aerosols sampled with TSI tubing changed the charging characteristic in the unipolar diffusion charger of the miniDiSC, causing the observed large discrepancies.
Table 2. Detected concentrations of siloxanes; IDs refer to ; n. d. = not detected.
The aerosol experiments also revealed that the use of Tygon and particularly Grimm tubing caused the opposite effect than the TSI tubes, i.e., the particle concentration increased, while the corona voltage decreased (). A possible reason may be that small molecules may degas from Grimm and Tygon tubes. In order to cause a higher than expected particle charging efficiency, these molecules need to be smaller than those typically assumed for describing the charging process, i.e., below 109 g/mol. A proton transfer reaction mass spectrometer (PTR-MS; model PTR-QMS 300, Ionicon, Innsbruck, Austria) was therefore used to measure the gas composition of room air, sampled directly through the same 75 cm long tubes used to sample on Tenax tubes. The PTR-MS is able to detect even minor changes in the gas phase for molar masses down to 21 g/mol. The PTR-MS sample flow rate was 40 ml/min. However, no significant change in the gas composition of the air was found for any of the investigated tubes. It can therefore be excluded that degassing of small molecules causes the increase of the charging efficiency for Grimm tubing. The actual reason hence remains unclear and requires further research.
Summary and conclusions
Sampling tubes are often required to transport the aerosol from the sampling point to a measurement instrument. It is well known that particle losses may affect the measurement accuracy. To avoid electrostatic losses, carbon impregnated, thus conductive silicone tubes are typically recommended. We have shown that siloxanes degas from some of these tubes and hence alter the gas composition of the sample aerosol. This effect was observed for tubes from TSI and was stronger for new than for aged tubes. Siloxanes are very large molecules and get preferentially ionized in unipolar diffusion chargers. The ion properties are therefore also affected and no longer match the ones during the calibration of the instruments without tubing. As a result, the charging efficiency is decreased, resulting in too low concentrations being measured with diffusion charger-based instruments. The discrepancies, caused by the choice of sampling tubes can amount to a factor of two or more in case of miniDiSC/DiSCmini. Partector showed the same effect, but to a much lower degree. This is slightly surprising since the two chargers are quite similar regarding their geometry and the electric field strength in the charging zone. However, in the partector, the charging current is five times lower than in the DiSCmini, and the charger is operated in a pulsed rather than a continuous mode. Consequently, the Partector charger has a lower current density, which is used to describe the ionization in the charger. The effect of the tube material on NSAM was only minor, because the ion jet flow gets filtered by a HEPA and an activated carbon filter that likely adsorbs most of the siloxanes.
Conductive silicone tubing from Grimm-Aerosol showed the opposite effect, i.e., the concentrations measured with two miniDiSCs increased by 14% and 5%, respectively, when new tubes were used. Measurements conducted with older Grimm tubes still principally showed the same effect, but increase was always below 5%. The reason for the different behavior remains unclear. Measurements concerning a possible effect of the tubing on the concentration of molecules with low molecular masses showed no apparent change in the gas composition. Further research is needed to better understand the effect and develop improved tube materials.
Additional measurements were conducted with stainless steel, Tygon, and a silicone-free tubing with undisclosed composition. All showed only a negligible effect on the measurements with unipolar diffusion chargers. Since stainless steel is non-flexible and the silicone-free tube is also rather stiff, the use of Tygon tubing is therefore recommended for measurements with unipolar diffusion chargers that require high flexibility of the sampling tube, e.g., in case of personal exposure measurements. This finding is in good agreement with a previous finding by Tsai (Citation2015), who measured the size distributions with an FMPS, sampling through Tygon and conductive TSI tubing. She reports that the overall concentration measured with Tygon tubing was on average around 10% higher than those measured with conductive silicone tubing and concludes that losses in Tygon tubes are lower. However, given the fact that the FMPS uses a unipolar diffusion charger to charge the particles prior to classification, the discrepancy between the measured concentrations may also stem from degassed siloxanes.
Funding
The work presented here was conducted as part of the nanoIndEx project. nanoIndEx is supported by the French National Funding Agency for Research (ANR), the German Federal Ministry of Education and Research (BMBF, IUTA grant no.: 03×0127A), the British Technology Strategy Board (TSB) and the Swiss TEMAS AG, under the frame of SIINN, the ERA-NET for a Safe Implementation of Innovative Nanoscience and Nanotechnology. The financial support is gratefully acknowledged.
References
- Asbach, C., Fissan, H., Stahlmecke, B., Kuhlbusch, T. A. J., and Pui, D. Y. H. (2009). Conceptual Limitations and Extensions of Lung-Deposited Nanoparticle Surface Area Monitor (NSAM). J. Nanopart. Res., 11:101–109.
- Asbach, C., Kaminski, H., Dahmann, D., Monz, C., Neumann, V., Plitzko, S., Meyer-Plath, A., Dziurowitz, N., Simonow, B., Fierz, M., and Todea, A. M. (2015). Genauigkeit, Vergleichbarkeit und Feldtauglichkeit Personengebundener Monitore zur Bestimmung der Exposition Gegenüber Nanopartikeln. Gefahrstoffe-Reinhaltung der Luft, 75:469–477.
- Brockmann, J. (2011). Aerosol Transport in Sampling Lines and Inlets, in Aerosol Measurement-Principles, Techniques, and Applications, P. Baron, K. Willeke, and P. Kulkarni, eds., John Wiley & Sons, Hoboken, NJ, USA, pp. 69–105.
- Davidson, J., and McKinney, P. (1998). Chemical Vapor Deposition in the Corona Discharge of Electrostatic Air Cleaners. Aerosol Sci. Technol., 29:102–110.
- Fierz, M. (2016). MiniDiSC Application Note #6: Silicon Oxide Deposits on the Corona Wire,“ [Online]. Available at http://fierz.ch/minidisc/pdf/miniDiSCApplicationNote6.pdf
- Fierz, M., Houle, C., Steigmeier, P., and Burtscher, H. (2011). Design, Calibration, and Field Performance of a Miniature Diffusion Size Classifier. Aerosol Sci. Technol., 45:1–10.
- Fierz, M., Meier, D., Steigmeier, P., and Burtscher, H. (2014). Aerosol Measurement by Induced Currents. Aerosol Sci. Technol., 48:350–357.
- Finger, H., Schneiderwind, U., and Asbach, C. (2015). Bewertung Mobiler Raumluftreinigungsgeräte. Gefahrstoffe–Reinhaltung der Luft, 75:497–502.
- Fissan, H., Neumann, S., Trampe, A., Pui, D. Y. H., and Shin, W. G. (2007). Rationale and Principle of an Instrument Measuring Lung Deposited Nanoparticle Surface Area. J. Nanopart. Res., 9:53–59.
- Fuchs, N. (1963). On the Stationary Charge Distribution on Aerosol Particles in a Bipolar Ionic Atmosphere. Geofis. Pura. Appl., 56:185–192.
- Gormley, P., and Kennedy, M. (1949). Diffusion from a Stream Flowing Through a Cylindrical Tube. Proc. R. Irish Acad., 52A:163–169.
- Keskinen, J., Pietarinen, K., and Lehtimäki, M. (1992). Electrical Low Pressure Impactor. J. Aerosol Sci., 23:353–360.
- Liu, B. Y. H., Pui, D. Y. H., Rubow, K., and Szymanski, W. (1985). Electrostatic Effects in Aerosol Sampling and Filtration. Ann. Occup. Hyg., 29:251–269.
- Maißer, A., Thomas, J., Larriba-Andaluz, C., He, S., and Hogan Jr., C. (2015). The Mass-Mobility Distribution of Ions Produced by a Po-210 Source in Air. J. Aerosol Sci., 90:36–50.
- Marra, J., Voetz, M., and Kiesling, H. J. (2010). Monitor for Detecting and Assessing Exposure to Airborne Nanoparticles. J. Nanopart. Res., 12:21–37.
- Medved, A., Dorman, F., Kaufman, S., and Poecher, A. (2000). A New Corona-Based Charger for Aerosol Particles. J. Aerosol Sci., 31:S616–S617.
- Nashimoto, K. (1989). Morphology and Structure of Silicon-Oxides Grown on Wire Electrodes by Positive Corona Discharges. J. Electrochem. Soc., 136:2320–2327.
- Pui, D. Y. H., Fruin, S., and McMurry, P. (1988). Unipolar Diffusion Charging of Ultrafine Aerosols. Aerosol Sci. Technol., 8:173–187.
- Pui, D. Y. H., Romay-Novas, F., and Liu, B. Y. H. (1987). Experimental Study of Particle Deposition in Bends of Circular Cross Sections. Aerosol Sci. Technol., 7:301–315.
- Shin, W. G., Pui, D. Y. H., Fissan, H., Neumann, S., and Trampe, A. (2007). Calibration and Numerical Simulation of Nanoparticle Surface Area Monitor (TSI Model 3550 NSAM). J. Nanopart. Res., 9:61–69.
- Stabile, L., Cauda, E., Marini, S., and Buonanno, G. (2014). Metrological Assessment of a Portable Analyzer for Monitoring the Particle Size Distribution of Ultrafine Particles. Ann. Occup. Hyg., 58:860–876.
- Thomas, J. (1959). Gravity Settling of Particles in a Horizontal Tube. J. Air Pollut. Control Assoc., 8:32–34.
- Timko, M., Yu, Z., Kroll, J., Jayne, J., Worsnop, D., Miake-Lye, R., Onasch, T., Liscinsky, D., Kirchstetter, T., Destaillats, H., Holder, A., Smith, J., and Wilson, K. (2009). Sampling Artifacts From Conductive Silicone Tubing. Aerosol Sci. Technol., 43:855–865.
- Tritscher, T., Beeston, M., Zerrath, A, Elzey, S., Krinke, T., Filimundi, E., and Bischof, O. (2013). NanoScan SMPS–A Novel, Portable Nanoparticle Sizing and Counting Instrument. J. Phys.: Conf. Ser., 429:012061.
- Tsai, C. (2015). Characterization of Airborne Nanoparticle Loss in Sampling Tubing. J. Occup. Environ. Hyg., 12:D161–D167.
- Wang, S., and Flagan, R. (1990). Scanning Mobility Particle Sizer. Aerosol Sci. Technol., 13:230–240.
- Wiedensohler, A. (1988). An Approximation of the Bipolar Charge Distribution for Particles in the Submicron Size Range. J. Aerosol Sci., 19:387–389.
- Yu, Y., Alexander, M., Perraud, V., Brins, E., Johnson, S., Ezell, M., and Finlayson-Pitts, B. (2009). Contamination From Electrically Conductive Silicone Tubing During Aerosol Chemical Analysis. Atm. Environ., 43:2836–2839.