ABSTRACT
The influence of volatilization from mainstream tobacco smoke particles in annular denuders on the gas-particle distribution was investigated by using multiple annular denuders coupled with a filter pack method (multiple-AD-FP method) under low flow volume conditions (1.05 and 1.65 L/min). Smoking experiments and model experiments using poly styrene latex (PSL) particles and generated gases were conducted to measure the particle loss ratio and gas collection efficiency in the denuders. In this research, we focused on the influence of XAD-4 slurry concentrations and particle volatility including tobacco smoke from a cigarette and vapor products on the volatilization from particles in the denuders, and have proposed a measurement method for considering volatilization. Our experimental results showed that volatilization depends on the particle volatility, targeted components, denuder length, particle concentration, and adsorbent amount, thereby suggesting the importance of selecting the proper measurement conditions and taking volatilization into account, so as to evaluate the appropriate gas-particle distribution. The gas-phase nicotine ratio obtained from calculating the volatilization rate in the multiple-AD-FP method agreed with previous research. This means that the calculation method was appropriate for the evaluation of gas-particle distribution. Furthermore, the gas-particle ratio determined through the multiple-AD-FP method was compared to that determined through a filter-impinger method that uses a glass fiber filter. The results revealed that most of the gases in the filter-impinger method were not collected in the impinger due to the condensation of gases on the surface of the filter fibers and collected particles.
© 2017 American Association for Aerosol Research
EDITOR:
Introduction
Accurate measurements of gas-particle distribution are important for semi-volatile components so as to investigate the transport phenomena. Previous research has conducted a wide variety of measurements, including the use of a filter-absorbent method and a denuder-filter method. Recently, a tube denuder method (Forbes et al. Citation2012) and a membrane denuder method (Liu et al. Citation2012) have been reported for the sake of saving costs. In the case of using filters, the gas amount depends on the collection positions before or behind the filters, due to the condensation of gas components on the surface of the filter fibers and collected particles. The condensation has been commonly known as one of the sampling artifacts (Volcknes and Leith Citation2003). Denuder methods that trap the gas components before the filters can effectively prevent the artifact; hence, a large number of atmospheric samplings have been implemented with denuders. In particular, the Annual Denuders coupled with a filder pack (AD-FP) method that employs single or multi-channel annular denuders, a filter pack, and an optional cyclone with various cut-off diameters has spread widely. The AD-FP method is mainly used for atmospheric samplings of aerosols to measure the gas-particle distribution with low concentrations over a long time. Conversely, the AD-FP method has rarely been applied for the sampling of aerosols with high concentrations over a short time. Examples of such aerosols with high concentrations include mainstream tobacco smoke. The denuder method was utilized to measure the gas-particle distribution of tobacco smoke, while previous research used the glass of single tube denuders. Lewis et al. (Citation1994) used glass tube denuders coated with oxalic acid. The volatilization rate from the tobacco smoke particles with respect to tube length was obtained in the method, and the initial gas amount at the inlet of the denuders was calculated based on the volatilization rate. A number of researchers have used glass tube denuders (Lewis et al. Citation1995; Hager and Niessner Citation1996; Mariner and Frost Citation1998; Ingebrethsem et al. Citation2001; Cochran et al. Citation2003; Lipowicz and Piade Citation2004; Katayama et al. Citation2007). These research efforts took the influence of volatilization from the particles into consideration and analyzed the gas-particle distribution based on calculations; however, gas collection efficiencies and particle loss ratios were not sufficiently discussed in the papers. As the measurable components capable of reacting with the coated solution were limited, and a glass tube at least one meter long is needed due to the low gas collection efficiency and capacity, the method has low versatility from the standpoint of operation. In contrast, these research efforts showed that the gas amount is influenced by volatilization from the particles in the denuders under low flow volume conditions. As the flow volume of tobacco smoking conditions are 1.05–1.65 L/min, the denuder collection on the direct measurements must be performed under the low flow volume conditions in the view point of analysis. AD-FP method has never been applied at the flow volume, thus the applicability to a low flow volume still remains unclear. Kamens and Coe (Citation1995) used five-channel annular denuders under the condition of 5–20 L/min and mentioned that the influence of volatilization from the particles in annular denuders depends on the targeted components and experimental conditions. In their report, the volatilization was described as off gassing, and the influence was significant, especially at 5 L/min. Although Koutrakis et al. (Citation1989) and Gundel et al. (Citation1995) measured the gas-particle distribution for environmental tobacco smoke by using the AD-FP method, the influence of volatilization from the particles was not taken into account.
Previous research using annular denuders concerned a breakthrough of the adsorbent and conducted experiments under excessive adsorbent conditions. Gas collection efficiencies and particle loss ratios have been extensively reported in literature, while there have been few reports about the influence of volatilization from particles in the denuders. It seems that multi-channel annular denuders have high gas collection efficiency and capacity, although the influence of volatilization from the particles must be significant because the flow rate at each channel decreases with respect to the number of denuder channels.
The purpose of this research is to investigate the influence of volatilization from mainstream tobacco smoke particles in annular denuders under low flow volume conditions, and to present a method of measuring the gas-particle distribution that takes the influence of volatilization into account. In the measurements, the direct introduction of mainstream tobacco smoke to the annular denuders under regulated smoking conditions was performed as the first attempt, and the influence of the XAD-4 slurry concentrations and the volatility of particles on the gas-particle distribution were examined. In addition, the results of the gas-particle distribution derived by the multiple-AD-FP method were evaluated for a comparison with the results obtained by the previous method (filter-impinger method). Given that smoking conditions in this research are a low flow volume (1.05 and 1.65 L/min), and the particle diameter of tobacco smoke peaks at around 200 nm on a number basis (Katayama et al. Citation2005), deposition on the wall surface in the denuders must be considered due to the diffusion effect of the particles. Considerable attention has been paid to high flow volume conditions (Koutrakis et al. Citation1990; Ye et al. Citation1991; Roussel et al. Citation2004a; Sekiguchi et al. Citation2009); however, there have been limited studies about low flow volume conditions. For the gas collection efficiencies, Possanzini et al. (Citation1983) conducted experiments using single channel annular denuders within the range of 1.2–40 L/min, and Febo et al. (Citation1986) conducted experiments within the range of 0.5–2.5 L/min. Other research (Roussel et al. Citation2004b; Sekiguchi et al. Citation2009) assessed performance under high flow volume conditions (16.7 and 40 L/min). Few studies have attempted to evaluate gas collection efficiencies under low flow volume conditions with multi-channel annular denuders. Therefore, this research focused on low flow volume conditions with multi-channel denuders from the perspective of particle loss ratio and gas collection efficiency.
Experimental
Samples of a cigarette and vapor products
lists the samples used in this research. Cigarette A contained menthol in part of the cut-fillers (12,000 ppm on a weight basis against the net tobacco weight) and in the filter (9.5 mg). For vapor product B and C, we used Ploom produced by Japan Tobacco (Tokyo, Japan) as an atomizer to generate the volatile particles. Ploom has a small aluminium container called a pod with the cut-fillers and aerosol substrates in it. The pod was heated at 170°C and then the aerosol substrate was vaporized and cooled to generate the volatile particles. In this research, the pod originally consisted of aerosol substrates and cut fillers at a proportion of three to two on a weight basis. The cut-fillers consisted of burley pulverized with a commercially available mixer (Iwatani, Osaka, Japan, IFM-800) and sieved to less than 0.5 mm. Each aerosol substrate consisted of glycerin or a mixture of glycerin and propylene glycol with the same amount, and with the menthol added at a concentration of 2% against the total weight of the cut-fillers and aerosol substrates. The cut-fillers, aerosol substrates, and menthol were mixed well and pasted into the inner surface of the pod.
Table 1. Samples specs and components' amount in the mainstream tobacco smoke.
Preparation of the denuder coating
The three-channel annular denuders (URG, Chapel Hill, USA, URG-2000–30×100–3CSS [effective denuder length: 8 cm], URG-2000–30×150-3CSS [effective denuder length: 13 cm]), which have high gas collection efficiency were used in this research, where the effective denuder length means the length of the surface processed in a frosted glass state. The denuders can be connected together to make them longer, and XAD-4 (SIGMA-Aldrich Japan, Tokyo, Japan, Amberlite Particle size 20–60 mesh (wet)) was selected as an adsorbent. XAD-4 was put into the stainless container (Irie Shokai, Tokyo, Japan, 60 mmφ, 110 mL) that had 15 stainless balls with a diameter of 11 mm in it to be crushed by a ball mill (Irie Shokai, V-1ML) at 200 rpm for 72 h (the effect of the crushing time on the size distribution is shown in the online supplemental information [SI] Figure S1). Subsequently, XAD-4 was subject to ultrasonic cleaning in dichloromethane, and then to suction drying with methanol. The crushed XAD-4 was sonicated for 30 min in hexane, and the slurry was dropped into the denuders. The coating was performed by hand-shaking for 1 min, and then dried with nitrogen at 0.5 L/min for 2 min so as to evaporate the hexane and adhere XAD-4 to the inner surface of the denuders. Both coating and drying were repeated five times, thereby stabilizing the coated amount and preventing variations in the amounts of gas measured. We referred the preparation method of Gundel et al. (Citation1995) except for the number of coating times for the time-saving (see the reference for further details). In this research, six levels of XAD-4 slurry concentrations were prepared (0, 0.2, 0.6, 1.0, 2.0, and 4.0 mg/mL) to examine the influence of adsorbent amount on the volatilization from the particles. The hexane volume was changed to match the slurry concentration against the inner surface of the denuders at 10 mL (8 cm) and 16 mL (13 cm). The coating efficiency can indirectly be evaluated by measuring the weight loss of XAD-4 before and after coating. The efficiency was 82 ± 4% (N = 12, Slurry concentration 2.0 mg/mL) in this experimental condition.
Extraction and gas chromatograph analysis
One target component in this research is menthol, which is known as one of the flavor and taste components having gas-particle distribution. In addition, nicotine (for which previous research measured the gas-particle distribution by using glass tube denuders) was also selected to verify the validity of our measurement method. Given the large amounts of our target components that are included in tobacco smoke, and thanks to the uncomplicated analytical methods available, high precision measurements can be performed. XAD-4 attached to the inner surface of the denuders was extracted by ultrasonic processing for 20 min in cyclohexane. We confirmed in advance that the initial extraction efficiency of the denuders exceeded 96%, which was utilized to correct the measurement results. The efficiency was derived by the continuous extraction and calculated by the ratio of the concentration (first extraction/ (first + second) extraction). A Cambridge filter (Borgwaldt, Hamburg, Germany, 44 mmφ) in the filter pack was extracted in methanol with 40 min of shaking at 175 rpm. The extraction solvents were filtered and analyzed by gas chromatograph (GC, Agilent, California, USA, 7890A) as described by Ishikawa and Sekiguchi (Citation2016). Menthol, nicotine, and glycerin can be measured in the method at the same time.
Smoking experiments using the multiple-AD-FP method and filter-impinger method
The smoking experiments were conducted by using a smoking machine (Borgwaldt, RM-26), and the denuders were set vertically to minimize particle loss as shown in . Smoking was performed under ISO condition (puff: 35 mL/2 s, interval: 58 s) for cigarette A and Health Canada Intensive condition (HCI, puff: 55 mL/2 s, interval: 28 s) for vapor product B and C. The solenoid valve turns on and immediately switches the air pathway to discharge the smoke at the same flow rate as that of the smoking conditions (ISO condition: 1.05 L/min for 25 s and HCI condition: 1.65 L/min for 15 s) after puffing so as to prevent smoke from remaining in the denuders. In the smoking experiments, the number of puffs on the cigarette and vapor products was fixed at 6 and 10, respectively. Two cigarettes and two pods were subsequently smoked, and the measurements were repeated four times. We checked the smoke temperature of samples at the inlet of the first denuder. There was no difference with a room temperature (22°C); therefore, the influence of the smoke temperature on the gas-particle distribution was negligible in this research.
The filter-impinger method was used to compare the gas-particle distribution results with those of the multiple-AD-FP method. In the experiments, components in the particle-phase were trapped by a Cambridge filter made of glass fibers in the former stage, while components in the gas-phase were collected by the impinger in the latter stage. The impinger has 10 mL of methanol that was cooled with ethanol added dry ices to suppress the volatilization.
Measurements for particle size distributions of tobacco smoke from the cigarette and vapor products
The measurements for particle size distributions were performed by Spraytec (Mulvern, Worcestershire, UK, model STP5321). The smoke after puffing was exposed to He-Ne laser (632.8 nm) in a small cell box (70 cm3) and then the size distributions were determined. The measurements were made three times for each puff, and the size distribution results were averaged. We exploited and averaged the results within the range of transmitted laser intensity (20–60% at 0.1 s intervals) during each puff. Based on the principles of the device, the results obtained from Spraytec are output as weight frequency distribution, and the measurement results were converted to the number frequency distribution by calculation. The purpose of measuring the particle size distribution was to confirm that there was not significant difference between the cigarette and vapor products.
Experimental method for particle loss ratio and gas collection efficiency
For the measurement of particle loss ratio, PSL particles (JSR, Ibaraki, Japan, Size Standard Particles 55, 101, 202 nm) were generated by using an atomizer (TSI, St. Paul, MN, USA, Model 3079), a diffusion dryer (TSI, Model 3062), and a neutralizer (Kanomax, Osaka, Japan, Am - 241), and then the particles were passed through the denuders at 1.05 or 1.65 L/min. It can be assumed that particle loss is dominant in small diameter particles that are influenced by the diffusion effect. The smoke in our samples had a peak diameter at 100–200 nm on a number basis. Accordingly, the diameters of PSL particles (55, 101, 202 nm) were selected and generated as monodispersed particles in this research. The particle loss ratio was calculated by the number of particles gap between before and after passing through the denuders. The number of particles was measured by Scanning Mobility Particle Sizer (SMPS, TSI, CPC; Model 3022A, DMA; Model 3081). The measurement was repeated five times, and the scanning time was 5 min (2 min for up-scan, 1 min for down-scan, and a 2-min interval). The experiments for the particle loss ratio were performed without XAD-4 coating in the denuders, as Ye et al. (Citation1991) reported that the influence of XAD-4 coating on particle loss was negligible. In addition to the model particle experiments, we conducted experiments for the actual particle loss ratio under the smoking conditions. Glycerin was selected as a common low volatile component included in the tobacco smoke from the cigarette and vapor products to evaluate the actual particle loss ratio. The components, which have a low volatility and are mainly distributed in the particle-phase, must be utilized as an indicator of the particle loss ratio.
For the measurement of gas collection efficiency, a reagent of 0.2 mg of nicotine (SIGMA-Aldrich Japan, N3876) and 0.2 mg of L-menthol (Wako Pure Chemical Industries, Osaka, Japan, 136–03755) was set on the quartz board, and then heated by an electronic furnace (Asahi Rika Manufacturing, Chiba, Japan, ARF-30K) at 100°C to generate the gas-phase nicotine and menthol. The PTFE filter (Tokyo Roshi, Tokyo, Japan, T020A047A) was set in the experimental line after the furnace to eliminate the particles generated in gasification. The amounts of nicotine and menthol were expected to slightly exceed the gas amount in the smoke of our samples according to previous research related to nicotine (Lewis et al. Citation1994). Both gasification and collection were carried out for 10 min through the denuders and impinger at 1.05 and 1.65 L/min. The excessive conditions of the gas amount were also conducted with 1.0 mg of nicotine and 1.0 mg of L-menthol to confirm the breakthrough of XAD-4. The gas collection efficiency was calculated by the ratio of the amount in each denuder to the total amount, including the impinger. In the experiment, the slurry concentration of XAD-4 was set at 1.0 mg/mL, and the denuder length was fixed to 68 cm (combinations: 13, 13, 13, 13, 8, and 8 cm). The schematic diagrams of the experimental setups for particle loss ratio and gas collection efficiency are shown in Figure S2.
Method of calculating gas-particle distribution with the influence of volatilization taken into account
The measurements of gas-particle distribution in consideration of the influence of volatilization from the particles were performed to obtain the volatilization rate, so as to change the denuder length. On the assumption that the initial gas in the smoke at the denuder inlet was completely trapped by the first denuder, the gases collected in the latter denuders correspond to volatilized gases from the particles. Furthermore, assuming that the adsorption rate of the gases in the denuders is faster than the volatilization rate from the particles, the volatilization rate is expressed by the pseudo-first-order equation below.[1]
The equation is transformed and integrated with respect to C and L from C0 to Ci and from 0 to Li, respectively. Equation (Equation2[2] ) can be given by the conversion of the formula as follows:
[2] where K (cm−1) is the volatilization rate from particles, L (cm) is the denuder length, i is the number of denuders, C0 is the initial particle ratio at the inlet of the first denuder, and Ci is the particle ratio at the outlet of the denuders. The equation can be expressed by Equation (Equation3
[3] ) because the total amount of the gas and particles show constant unity. As the amount of gas collected by each denuder contained not only the initial gas and volatilized gas from the particles but also particle loss, Equation (Equation3
[3] ) considered the particle loss ratio as determined by previous experiments on the actual particle loss ratio under the smoking conditions:
[3]
Equation (Equation3[3] ) means that volatilization rate K is obtained experimentally to plot the relationship between the natural logarithm of values by subtracting the amount of gas collected by the denuders from 1 in consideration of particle loss and the denuder length. If the target components have the initial gas amount, the pseudo-first-order straight line does not cross the origin. We can calculate initial gas ratio C0 (gas) from the intercept of the pseudo-first-order straight line.
Results and discussion
Particle size distributions of tobacco smoke from the cigarette and vapor products
shows the particle size distributions of the tobacco smoke from the cigarette and vapor products at each puff on a number basis. The tobacco smoke from the cigarette had a peak diameter at 200 nm; the smoke from the vapor products had a peak at 100–200 nm. The figure also reveals subtle differences in the size distributions at each puff. From the size distribution results, the effect of differences in size distributions on particle volatility and particle loss in the denuders among our samples was regarded as being negligible.
Particle loss ratio in the annular denuders
shows the results of the particle loss ratio with PSL particles. The particle loss ratios at diameters of 100 and 200 nm were lower than the ratio at a diameter of 55 nm. When comparing the results at 1.05 L/min and 1.65 L/min, it stands to reason that there would be a higher percentage of particle loss at 1.05 L/min. The results of the experiments were compared with the calculated ratio by using experimental Equations (Equation4)–(6) below proposed by Hind (Citation1982) for particle loss in annular denuders:[4]
[5]
[6] where ηL is the particle loss ratio, D (cm2/s) is the diffusion coefficient of particle diameter d, L (cm) is the denuder length, Q (cm3/s) is the flow volume, h (cm) is the annular space of the channel: (h = (d1 − d2) / 2), W (cm) is the mean perimeter of the channel: (W = π (d1 + d2) / 2)), d1 is the outer diameter of the annulus, and d2 is the inner diameter of the annulus. As the experimental results mostly agreed with the calculated results obtained by Equations (Equation4)–(6), the applicability of the equations to multi-channel denuders under low flow volume conditions should pose no problems. The particle size distributions on the mass basis of samples peak at around 400 nm, and the particle loss ratio is expected to be 0.01% per denuder (effective denuder length: 13 cm) according to Equations (Equation4)–(6). It consequently seems reasonable to conclude that the particle loss ratios in a steady flow were not an issue with our experimental conditions.
Figure 3. (a) Results of the particle loss ratios of the denuders in the PSL experiments (N = 5) and (b) glycerin loss ratios in substitution for PSL under the smoking conditions (N = 5). Both experiments were conducted without XAD-4 coating. The error bars in the figures show the standard deviation (1σ).
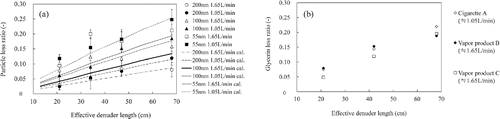
The particle loss ratio under the smoking conditions was also measured to examine the actual loss ratio of particles without XAD-4 coating in the denuders. It is clear from that the loss ratios of glycerin passed through the denuders (68 cm) were roughly 20% regardless of the samples. Although the smoking conditions of the cigarette and vapor products were slightly different, the particle size distributions of the smoke were identical. Thus, it is appropriate that the glycerin loss ratios of the smoke were at almost the same level among the samples. The actual particle loss ratio per a denuder can be calculated as follows:[7]
From Equation (Equation7[7] ), the particle loss ratio per denuder (13 cm) under the smoking conditions was 4%, which matched the results of PSL 55 nm in a steady flow. The ratio was beyond the scope of our assumption as the particle size distribution peaks at 100–200 nm on a number basis, and 400–500 nm on a weight basis, respectively. We think that an unsteady state of airflow in the denuders could be attributed to higher particle loss under the smoking conditions. In our experiments, smoke is introduced in the denuders by the smoking machine, and then the smoke is discharged and passes through the denuders to switch the air pathway by using the solenoid valves (with details shown in ). Therefore, the residual smoke that temporarily remains in the denuders during switching of the air pathway could lead to a higher particle loss ratio. Consequently, the particles loss ratios determined through the glycerin experiments were utilized as the results of the smoking experiments in order to remove particle loss from the amount collected by the denuders (Equation (Equation3
[3] )).
Gas collection efficiency of the denuders for menthol and nicotine
shows that the first denuder collected nearly 100% of menthol and nicotine gases in the simulated concentrations. In contrast, the collection efficiencies of the first denuder in excessive concentrations (Table S1) were decreased, and that the gases passed through the latter denuders. In the experiments, we confirmed in advance that desorption of gases collected from the denuders was negligible after 10 min of air ventilation. These results suggest that selecting the adsorbent amount in consideration of the concentrations of gases is essential for denuder collection. The results were compared to the calculated efficiencies obtained by Equation (Equation8[8] ) proposed by Possanzini et al. (Citation1983).
[8] where D is the diffusion coefficient (cm2/s), F is the flow volume (cm3/s), L (cm) is the denuder length, C0 is the concentration at the denuder inlet, and C is the concentration at the denuder outlet, d1 is the outer diameter of the annulus, and d2 is the inner diameter of the annulus. The diffusion coefficient of nicotine was referred to as 0.065 cm2/s (Lewis et al. Citation1994). As the diffusion coefficient of menthol has not been previously reported, 0.066 cm2/s (calculated by Graham's law on the basis of nicotine) was used in the calculation. From the calculation results of Equation (Equation8
[8] ), the gas collection efficiencies of menthol and nicotine under all the conditions were nearly 100%, which was in good agreement with the experimental results obtained for the simulated concentrations. These results show that the initial gas in the smoke of our samples can be trapped by the first denuder under a sufficient adsorbent condition. Accordingly, it can be assumed that the amount of gas collected by the latter denuders is derived from volatilization from the particles. In our results, the gas collection efficiency of menthol tended to be lower than that of nicotine. The reason for the difference is the ease of adsorption on the surface of the denuder wall and XAD-4. Van Loy et al. (Citation1998) reported that the nicotine in environmental tobacco smoke was largely deposited on various surfaces in chamber experiments, thereby suggesting the presence of other factors aside from the gas diffusion coefficient, which affect gas collection efficiency.
Table 2. Gas collection efficiencies of nicotine and menthol with respect to effective denuder length under the conditions of simulated concentrations.
Influence of volatilization from the particles in annular denuders on the gas-particle distribution
Influence of the XAD-4 slurry concentrations on volatilization from the particles
and show the ratios of menthol and nicotine collected with the denuders in the various XAD-4 slurry concentrations. The collected ratios were increased with respect to the denuder length, and the ratios in high slurry concentrations were higher than those in lower slurry concentrations. Menthol was almost collected in the gas-phase at a denuder length of more than 40 cm due to the higher volatility as compared to nicotine. These results reveal that the denuder length and adsorbent amount have great impact on the gas-particle distribution under low flow volume conditions. The results of the ratios were applied to Equations (Equation1)–(3) in order to calculate the volatilization rate from the particles. The result without XAD-4 depicts that the ratios were nearly zero, indicating the suitability of our calculation for particle loss in Equation (Equation3[3] ). Since menthol was collected in the early stage of the denuders as previously stated, the volatilization rate was calculated within the possible range of the pseudo-first-order rate.
Figure 4. Gas-phase ratio of (a) menthol (N = 4) and (b) nicotine (N = 4) in the tobacco smoke and the relationships between -ln(1-C) of (c) menthol and (d) nicotine calculated by Equation (Equation3[3] ) and effective denuder length with various XAD-4 slurry concentrations. The gas ratio was subtracted by the particle loss ratio determined through the experiments of glycerin. The error bars in the figures show the standard deviation (1σ).
![Figure 4. Gas-phase ratio of (a) menthol (N = 4) and (b) nicotine (N = 4) in the tobacco smoke and the relationships between -ln(1-C) of (c) menthol and (d) nicotine calculated by Equation (Equation3[3] ) and effective denuder length with various XAD-4 slurry concentrations. The gas ratio was subtracted by the particle loss ratio determined through the experiments of glycerin. The error bars in the figures show the standard deviation (1σ).](/cms/asset/498971b8-ba3e-4876-8bf7-104e66c6a54e/uast_a_1290213_f0004_b.gif)
From and , it can be seen that the approximate straight lines do not cross the origin and have the intercept that shows the initial gas ratio at the inlet of the denuders. In case of an insufficient slurry concentration (0.2 mg/mL), the approximate straight line crossed near the origin because XAD-4 was saturated with the gases and every denuder has the same gas amount. In contrast, the initial gas amount was reflected in the results of sufficient slurry concentrations (0.6–4.0 mg/mL) that have the intercept of the approximate line. As has been observed in the results of menthol, it should be noted that highly volatile components are easily distributed to the gas-phase at the former stage of the denuders under excessive adsorbent conditions (2.0 and 4.0 mg/mL). As for the volatilization rate, the rate of menthol was 7–9 times higher than that of nicotine, indicating the influence of the components on volatilization. Therefore, it is important for the measurement of gas-particle distribution to select the appropriate adsorbent amount corresponding to target components. In the results, the optimum slurry concentration that enables an accurate measurement of the volatilization rate of both menthol and nicotine was 1.0 mg/mL, as volatilization did not terminate until the last denuder. In the concentration, all the data having different denuder lengths can be calculated as the pseudo-first-order rate.
Influence of particle volatility on volatilization from the particles
A comparison between tobacco smoke from the cigarette and vapor products was conducted to investigate the influence of particle volatility on volatilization from the particles. The slurry concentration was 1.0 mg/mL based on the previous experiments as described earlier. shows the results of the volatilization rates (and Figure S3 shows that of gases ratios). As the vapor products had a higher ratio of gases volatilized from the particles than the cigarette along with the denuder length, the volatilization rates of the tobacco smoke from the vapor products were two to six times higher than those of the smoke from the cigarette. Tobacco smoke from cigarettes is generated from combustion and contains a large number of components that include organic acids and low volatile oily substances (Dube and Green Citation1982), thereby resulting in low volatility of the particles. Conversely, tobacco smoke from the vapor products, consisting of glycerin and propylene glycol, has high volatility compared with the smoke from cigarettes. Accordingly, the differences of the volatilization rate must derive from the dissimilarity of component composition in the particles. The gap between the volatilization rates of vapor product B and C can be attributed not to the difference in particle size distribution but to the particle concentration as the size distributions were virtually the same. Vapor product C containing only glycerin has a lower total particulate matter (TPM) amount than vapor product B that contains propylene glycol having a low boiling point. The difference in particle concentration leads to the gap in volatilization rates due to the accelerated equilibrium from particle to gas. Given these comparison results, the influences of volatilization from the particles must be considered in the case of particles with higher volatility and/or a low particle concentration.
Figure 5. The relationships between –ln (1-C) of (a) menthol and (b) nicotine calculated by Equation (Equation3[3] ) and effective denuder length with the three kinds of tobacco smoke particles.
![Figure 5. The relationships between –ln (1-C) of (a) menthol and (b) nicotine calculated by Equation (Equation3[3] ) and effective denuder length with the three kinds of tobacco smoke particles.](/cms/asset/a4a7f40f-107a-4a9c-9b76-5e8f3ad4b24f/uast_a_1290213_f0005_b.gif)
Comparison between the multiple-AD-FP method and filter-impinger method
A comparison of the gas-particle distribution results was made between the multiple-AD-FP method and filter-impinger method used with the Cambridge pad, as previously used in tobacco research. shows the results of the comparison. The initial gas ratios of the multiple-AD-FP method were determined through calculation by using Equations (Equation1)–(3). There were very few gas ratios of all samples with the filter-impinger method in comparison with the multiple-AD-FP method. These results indicate that the amounts of gas collected by the filter-impinger method were underestimated due to the condensation of gases on the surface of the filter fibers and collected particles, especially under low flow volume conditions. The gas ratio of nicotine determined through the multiple-AD-FP method was about 1.4% in mainstream tobacco smoke with cigarette A. Our results are appropriate because the ratio of nicotine agreed closely with the results obtained by Lewis et al. (Citation1995) and Cochran et al. (Citation1995). Given that the gas ratio of nicotine is measured without considering volatilization, the ratio depends on the denuder length (10–30% with details shown in Figure S3b) and largely differs from that of the method with considering volatilization. Generally, denuders are used with two or three stages to prevent a breakthrough. Permanent gas components are not an issue with the use of denuder connection; however, such connections accelerate volatilization from the particle for semi-volatile components. Therefore, we would like to emphasize the need for confirmation of the influence of volatilization in the denuders as one of the fundamental evaluations.
Table 3. Gas-particle distribution of menthol and nicotine in tobacco smoke from the cigarette and vapor products with the multiple-AD-FP method and filter-impinger method.
With the filter-impinger method, there are concerns about gas condensation on the surface of the filter fibers and collected particles, and volatilization from the collected particles due to the preceding collection of particles by the filters. The volatilization from the collected particles was expected to have an impact on the gas-particle distribution in atmospheric samplings at a high flow volume over a long time, whereas the influence was negligible in our experimental conditions due to the short collection time. Therefore, the influence of gas condensation on the surface of the filter fibers and collected particles was reflected in the results, having a significant impact on the gas-particle distribution.
Conclusion
The multiple-AD-FP method was applied to mainstream tobacco smoke, and the influence of volatilization from the particles in annular denuders was investigated. The particle loss ratio and gas collection efficiency under low flow volume conditions agreed with the results of experimental equations reported from previous research. Our results showed that the influence of volatilization depended on the particle volatility, targeted components, denuder length, particle concentration, and adsorbent amount. Highly volatile components and particles had a significant impact on volatilization. Therefore, it is essential to select the proper coating conditions and take volatilization into account in consideration of the target components and particle volatility, in order to accurately measure the gas-particle distribution with the denuders under low flow volume conditions. It can be assumed that the influence of volatilization from the particles would be reduced in proportion to the volume of airflow; however, volatilization must be considered in atmospheric samplings, particularly under low flow volume conditions. Given the fact that volatilization occurred even from tobacco smoke particles generated from combustion, volatilization from the particles in the atmosphere must also occur. The results of the multiple-AD-FP method in this research agreed with the results of previous research; however, the results of the filter-impinger method did not agree due to the condensation of gases on the surface of the filter fibers and collected particles. Therefore, our proposed method is useful in accurate measurements of the gas-particle distribution and volatilization rate in the denuders.
UAST_1290213_Supplemental_File.zip
Download Zip (221 KB)References
- Cochran, E. W., Joseph, M. J., Stinson, S. L., and Summers, S. S. (2003). Application of a Diffusion-Denuder Method for the Investigation of the Effects of “Smoke pH” on Vapor-Phase Nicotine Yields from Different Types of Cigarettes. Beitr. Tabakforsch. Int., 20(6):365–372.
- Dube, M. F., and Green, C. R. (1982). Method of Collection of Smoke for Analytical Purposes. Rec. Adv. Tob. Sci., 8:42–102.
- Febo, A., Dipalo, V., and Possanzini, M. (1986). The Determination of Tetraalkyl Lead in Air by a Denuder Diffusion Technique. Sci. Total Environ., 48:187–194.
- Forbes, P. B. C., Karg, E. W., Zimmermann, R., and Rohwer, E. R. (2012). The Use of Multi-Channel Silicone Rubber Traps as Denuders for Polycyclic Aromatic Hydrocarbons. Anal. Chim. Acta, 730:71–79.
- Gundel, L. A., Lee, V. C., Mahanama, K. R. R., Stevens, R. K., and Daisey, J. M. (1995). Direct Determination of the Phase Distributions of Semi-volatile Polycyclic Aromatic Hydrocarbons Using Annular Denuders. Atmos. Environ., 29(14):1719–1733.
- Hager, B., and Niessner, R. (1996). On the Distribution of Nicotine between the Gas and Particle Phase and Its Measurement. Aerosol Sci. Technol., 26:163–174.
- Hinds, W. C. (1982). Aerosol Technology: Properties, Behavior, and Measurements of Airborne Particles. Wiley, New York, pp. 150–170.
- Ingebrethsen, B. J., Lyman, C. S., Risner, C. H., Martin, P., and Gordon, B. M. (2001). Particle-Gas Equilibria of Ammonia and Nicotine in Mainstream Cigarette Smoke. Aerosol Sci. Technol., 35:874–886.
- Ishikawa, N., and Sekiguchi, K. (2016). Experimental Study on Mechanical Filtration through Tobacco Columns: Influence of Cut-filler Shape and Size Distribution on Filtration Efficiencies. Aerosol Sci. Technol., 50:521–533.
- Kamens, M. R., and Coe, L. D. (1995). A Large Gas-Phase Stripping Device to Investigate Rates of PAH Evaporation from Airborne Diesel Soot Particles. Environ. Sci. Technol., 31:1830–1833.
- Katayama, K., Miura, K., Kitao, S., Shimada, M., and Okuyama, K. (2005). Measurement of Property Change in Cigarette Main-Stream Smoke Using Laser Light Scattering Method. Earozoru Kenkyu, 20:345–351 ( in Japanese).
- Katayama, K., Miura, K., Shimada, M., and Okuyama, K. (2007). Measurement of Evaporation Property of Cigarette Main-Stream Smoke Using Denuder Tube Method. Earozoru Kenkyu, 22:322–326 ( in Japanese).
- Koutrakis, P., Fasano, A. M., Slater, J. L., Spengler, J. D., McCarthy, J. F., and Leaderer, B. P. (1989). Design of a Personal Annular Denuders Sampler to Measure Atmospheric Aerosols and Gases. Atmos. Environ., 23(12):2767–2773.
- Koutrakis, P., Wolfson, J., Brauer, M. M., and Spengler, D. (1990). Design of a Glass Impactor for an Annular Denuder/Filter Pack System. Aerosol Sci. Technol., 12:607–612.
- Lewis, D. A., Colbeck, I., and Mariner, D. C. (1994). Diffusion Denuder Method for Sampling Vapor-Phase nicotine in Mainstream Tobacco Smoke. Anal. Chem., 66:3525–3527.
- Lewis, D. A., Colbeck, I., and Mariner, D. C. (1995). Dilution of Mainstream Tobacco Smoke and Its Effects upon the Evaporation and Diffusion of Nicotine. J. Aerosol Sci., 26(5):841–846.
- Lipowicz, P. J., and Piade, J. J. (2004). Evaporation and Subsequent Deposition of Nicotine from Mainstream Cigarette Smoke in a Denuder Tube. J. Aerosol Sci., 35:33–45.
- Liu, S., Alex, T., Chang-Yu, W., Yu-Mei, H., and Brian, B. (2012). Development of a Novel Porous Membrane Denuder for SO2 Measurement. Aerosol Air Qual. Res., 12:1116–1124.
- Mariner, D. C., and Frost, B. F. (1998). Determination of Nicotine Evaporation from Mainstream Smoke Using Denuder Tubes. in Proceedings of Papers Presented at the Joint Meeting of the Smoke and Technology Groups (CORESTA), pp. 167–170.
- Possanzini, M., Febo, A., and Liberti, A. (1983). New Design of a High-Performance Denuder for the Sampling of Atmospheric Pollutants. Atmos. Environ., 17(12):2605–2610.
- Roussel, B., Monod, A., Massiani, C., and Wortham, H. (2004a). Evaluation of an Annular Denuder Tubes for Atmospheric PAH Partitioning Studied-2: Evaluation of Mass and Number Particle Losses. Atmos. Environ., 38:1925–1932.
- Roussel, B., Monod, A., Massiani, C., and Wortham, H. (2004b). Evaluation of an Annular Denuder Tubes for Atmospheric PAH Partitioning Studied-1: Evaluation of the Trapping Efficiency of Gaseous PAHs. Atmos. Environ., 38:19213–1924.
- Sekiguchi, K., Kim, K. H., Kudo, S., Sakamoto, K., Otani, Y., Seto, T., Furuuchi, M., Yoshikawa, F., Ehara, K., Hirasawa, M., Tajima, N., and Kato, T. (2009). Evaluation of Multichannel Annular Denuders for a Newly Developed Ultrafine Particle Sampling System. Aerosol Air Qual. Res., 9(1):50–64.
- Van Loy, D. M., Nazaroff, W. W., and Daisey, M. J. (1998). Nicotine as a Marker for Environmental Tobacco Smoke Implications of Sorption on Indoor Surface Materials. J. Air Waste Manage. Assoc., 48:959–968.
- Volckens, J., and Leith, D. (2003). Effects of Sampling Bias on Gas-particle Partitioning of Semi-volatile Compounds. Atmos. Environ., 37:3385–3393.
- Ye, Y., Tsai, C. J., Pui, D. Y. H., and Lewis, C. W. (1991). Particle Transmission Characteristics of an Annular Denuder Ambient Sampling System. Aerosol Sci. Technol., 14:102–111.