ABSTRACT
An external electric field was applied on the filter to improve its collection efficiency, and the collection efficiencies of the different filters under various conditions were evaluated. Dominant electrical filtration mechanisms for each condition were investigated using experimental and theoretical approaches. Four types of air filters were used as test filters: a charged fiber filter, a low-grade filter with 50% collection efficiency in the most penetration particle size (MPPS) zone, and two high-grade filters with more than 95% collection efficiency in the MPPS zone. Three different particle charge states—neutralized, single-charged and uncharged—were considered. For neutralized particles, the external electric field led to a 14.5%p. and 2.5%p. increase in the collection efficiencies of the low-grade filter and charged fiber filter, respectively. With the electric field, the collection efficiency of the low-grade filter increased by 30%p. for single-charged particles. The electric field also affected the collection efficiencies of the charged filter and high-grade filters, but the effect was not significant. For uncharged particles, the electric field did not lead to a remarkable increase in the collection efficiencies of any of the filters. Through experimental and theoretical analysis, it was found that the polarization force imposed on the charged fiber was the dominant factor for the charged fiber filter regardless of application of the external electric field. The Coulombic force imposed on the electric field was the dominant factor for the low-grade filter, while both the Coulombic and the polarization forces affected the collection efficiency of the high-grade filter.
Copyright © 2017 American Association for Aerosol Research
EDITOR:
1. Introduction
Recently, various studies have been conducted on filters with high collection efficiency at a low pressure drop. Because both the collection efficiency and the pressure drop depend on the physical structure of the filter medium (such as the fiber diameter, porosity, and filter thickness), changing the filter's physical structure in order to increase its collection efficiency is inevitably accompanied by an increase in the pressure drop (Fisk et al. Citation2002; Ahn et al. Citation2006; Chuaybamroong et al. Citation2010).For this reason, electrical technologies such as providing a quasi-permanent electric charge to the filter fibers and applying an external electric field to the filter have been studied as a means of improving collection efficiency while retaining an almost constant pressure drop.
An electret filter made of charged fiber has been widely studied as a means of increasing the collection efficiency for charged or neutral aerosols (Yang et al. Citation2007; Chazelet et al. Citation2011; Li et al. Citation2012; Sim et al. Citation2015), and its filtration mechanism was reported by Romay et al. (Citation1998). In the latter study, it was found that the collection efficiency for particles smaller than 0.1μm was increased by the Coulombic force, while that for particles larger than 3 μm was increased by dielectrophoresis. Neutral particles larger than 0.1 μm were also influenced by the dielectric force. The two mechanisms were applied to particles in the range of 0.1–0.3 μm.
However, several research groups have reported the limitations of the electret filter. The additional capturing mechanism obtained by the charged fiber can decrease as a result of the particle loading on the filter fiber (Plopeanu et al. Citation2011; Rengasamy et al. Citation2013). Raynor and Chae (Citation2004) compared the collection efficiencies of two filters: uncharged glass fiber filters and charged polyolefin fiber filters with particle loading. The filters were installed in a ventilation system and operated for 134 days. After 134 days of use, the particle capturing ability of the charged polyolefin fiber filter was dramatically reduced, while the deposited particles on the glass fiber filter did not significantly affect the collection efficiency. Moreover, Walsh and Stenhouse (Citation1997) reported asserted that the degradation of the collection efficiency of an electret filter that occurred as a result of particle loading could be accelerated by increasing the size and charge number of the deposited particles.
To prevent the degradation of a filter's collection efficiency through dust loading, an external electric source can be applied to the filter medium to give it a permanent electric force. In the presence of an external electric field, the filter fiber and particles suspended in the electric field are polarized, and the particles are attracted to the filter fiber by the image force. Moreover, charged particles in the external electric field can be attracted to the filter fiber by the Coulombic force (Donovan Citation1985). Several studies of filtration systems containing an external electric field have been conducted. Kao et al. (Citation1987) proposed a theoretical model to predict the collection efficiency of a fibrous filter under a strong electric field and compared the calculation results with their experimental results. Thorpe and Brown (Citation2003) investigated the effects of electric field strength, size of particles and filter fiber, and filtration velocity on collection efficiency. In their study, monodisperse particles with Boltzmann charge distributions were used as test particles. Podgórski et al. (Citation1998) and Luckner et al. (Citation1998) studied the filtration abilities of filters with an external electric field using theoretical and experimental approaches, respectively. Park and Park (Citation2005) performed a numerical simulation of the particle deposition on the filter fiber in a uniform external electric field. They reported that the electrostatic attraction between an incoming particle and the particles already deposited on the filter fiber affected the particle deposition pattern.
Even though fairly extensive experimental and numerical research on the performance of filters with an external electric field has been conducted, to the best of our knowledge there remains a lack of research into the effects of the charge states of filter fibers and particles on the collection efficiency of such filters. Likewise, the dominant filtration mechanisms of filters with an external electric field under various filter and particle states have not been sufficiently studied. The influence of the external electric field may depend on the filter fiber charge state, the mechanical collection efficiency of the filter medium, and the charged state of the test particles. Moreover, the major electrical filtration mechanism may be changed by those filter and particle states. In order to better understand and design air filters using an external electric field, the effects of those filter and particle states on the collection efficiency should be examined.
This study investigated the effects of the charge state of test particles, the charge state and mechanical filtration efficiency of test filters, and the presence or absence of an external electric field on collection efficiency. Four different types of air filters were tested on neutralized, single-charged, and uncharged particles. The theoretical model was used to estimate the collection efficiency of the test filters and determine the dominant mechanisms involved.
2. Material and methods
2.1. Theoretical model
When an external electric field is applied to a filter, particles can be captured by the filter through mechanical mechanisms—gravitational settling, impaction, interception, and diffusion—and electrical mechanisms—Coulombic and polarization forces. More specifically, charged particles can be attracted to the filter fiber by the Coulombic force imposed on the external electric field or charged filter fiber. Moreover, the charged particles can lead to the polarization of the filter fiber. Thus, electrical attractive forces, called image forces, can also act on the particles. In the case of uncharged particles or fibers, polarization can be caused by the external electric field.
Generally, a single fiber model has been used to predict the collection efficiency. The collection efficiency (ηcollection) of any particle can be calculated as follows (Davies Citation1973):[1] where L is the filter thickness,
is the filter solidity, and df is the fiber diameter. ηs is the total single fiber efficiency that can be represented as the sum of the single fiber efficiencies through mechanical and electrical mechanisms. For nano-meter sized particles that can ignore gravitational settling, the ηscan be calculated as follows:
[2] where ηR, ηI and ηD are the single fiber efficiencies due to interception, impaction, and diffusion, respectively. ηE is the efficiency imposed by an electrical mechanism. Detailed descriptions of mechanical filtration mechanisms can be found in the literature (Davies Citation1973; Brown Citation1993). We focus on explaining the electrical mechanism. The ηE can be divided into three cases: the single fiber efficiencies of charged particles in the presence of an external electric field(ηE,q), the single fiber efficiencies of uncharged particles in the presence of an external field (ηE,0), and the efficiency of charged particles in the absence of an external electric field (η0,q). Each collection efficiency represents the effects of both the Coulombic and the polarization forces, and the effects of polarization forces by the external electric field and the charged particles, respectively. These efficiencies were calculated as follows (Brown Citation1993):
[3]
[4]
[5] where E is the strength of the electric field applied to the filter, q is the charge of the particle, μ is the air viscosity, dp is the particle diameter, U0 is the velocity of the air at a filter face, ϵf and ϵP are the dielectric constants of the filter fiber and particle, respectively, ε0 is the permittivity of a vacuum, and Cc is the Cunningham slip correction factor.
Because airborne aerosols are subject to an equilibrium bipolar charge distribution, called the Boltzmann charge distribution, some of the natural particles can be influenced by an electrical field when they are captured on the filter, even if their average charge state is zero. In order to consider the Coulombic and the polarization forces acting on the neutralized particles, the Boltzmann charge distribution of the particle with a diameter of dp (fcharge) was calculated, and the results were reflected in Equations (Equation3[3] ) and (Equation5
[5] ). For particles with diameters larger than 40 nm, the Boltzmann charge distribution of the particles (fcharge) can be expressed as follows (Liu and Pui Citation1974; Yeh Citation1976):
[6] where k is the Boltzmann constant, e is the elementary unit of charge, and T is the absolute temperature of the air.
2.2. Preparation of filter media
In this study, four different types of filter media were used as test specimens. The basic properties of each filter medium are summarized in . For convenience, each filter is marked using symbols. Filter A was an electrically charged filter medium consisting of three layers. In the case of filter A, the main filtration occurred in the second layer. Its first layer was approximately 150 μm and protected the second layer, which was made of melt-blown fiber and supported by the third layer. Filter B was the same as filter A, except the charges on the fiber were removed by treating it with isopropanol (IPA) following the electrostatic discharging procedure in the European Standard EN 779(2002). Filter C was a glass fiber filter medium (type A/E) manufactured by the Pall Corporation. Filter D was a laboratory-made filter medium fabricated by electrospinning a formic acid solution in which polyamide 66 (Rhodia Korea) was dissolved. Filter D might have been electrically charged during the electrospinning and handling processes. For this reason, the charges on the filter D were removed in the same way as with filter B. The mechanical collection efficiency of each filter for neutralized particles in the range of most penetration particle size (MPPS, ranging from 100 to 300 nm) was 86.0%, 49.9%, 97.2%, and 97.5 %, respectively. The field emission scanning electron microscope (FE-SEM, S-4700, Hitachi, Tokyo, Japan) images of each filters are shown in and . The images were obtained under 5 kV of accelerating voltage and 50, 500, and 5000 times magnification. shows the structures of filters A and B, which consisted of three layers (cover, middle layer, and substrate), and shows those of filters C and D. The diameter of the fiber in the second layer of filter A and filter B ranged from 2 μm to 10 μm. Filter C consisted of fibers with a diameter in the range of 0.1–1.5 μm. Filter D consisted of almost uniform fibers with a diameter of approximately 0.5 μm. The filter media were cut into circular sheets with a diameter of 50 mm for the filter test. Three filter samples for each test condition were used to reduce the error from the non-uniformity of the filter medium. Thus, all of the collection efficiency data plotted in this article were averaged from three filter samples. The filtration velocity was fixed as 5.3 m/s, which is the standard filtration velocity in the filter test (EN 1822–1).
Table 1. Fundamental properties of tested filter media.
2.3. Application of external electric field
In order to apply external electric fields to each air filter medium, two stainless steel wire meshes (woven type, wire diameter of 50 μm, width of 100 μm, and solidity of 0.31) were used as electrodes (S1). One mesh was located in front of the filter sample, while the other was located behind the filter. A Polytetrafluoroethylene (PTFE) ring with a width of 0.1 mm was located between the mesh and filter sample for insulation (). Then a high negative voltage (−1000 V) was applied to the upstream electrode, while the downstream electrode was grounded. Because the thickness of the filter samples was 0.5 mm for filters A, B, and C and 0.1 mm for filter D, their electrical strengths were 1.4 kV/mm for filters A, B, and C and 3.3 kV/mm for filter D.
2.4. Test particles
Poly-disperse aerosol particles were used to evaluate the collection efficiency of each filter according to particle size. In addition, mono-disperse particles with a conditioned charge state were used to investigate the effect of the particle charge state. In our experiment, sodium chloride (NaCl) particles were used as test particles. The test particles were generated by the atomization of a 0.1 wt% NaCl solution using a compressed-air atomizer (Model 9302A, TSI Inc., Shoreview, MN, USA), which was crystallized by being passed through a diffusion dryer and then sent to a mixing chamber. As shown in , for the case of the poly-disperse particles, two different particle charge states, a neutralized state (a state where the charge distribution of the particles followed the Boltzmann equilibrium charge distribution), and an uncharged state, were tested as follows. Along route I: NaCl particles passed through a Kr-85 aerosol neutralizer (Model 3077, TSI Inc.) to obtain neutralized particles. Along route II: NaCl particles passed through a Kr-85 aerosol neutralizer and an electrostatic precipitator (ESP) to capture the charged particles, and then provide only uncharged particles. In this study, a laboratory-made dual cylinder type ESP with an inner diameter of 25 mm, outer diameter of 30 mm, and length of 100 mm was used. When air ions were generated inside the ESP, it was difficult to expect a sufficient inlet particle concentration. Thus, the voltage applied on the ESP was determined by considering its corona onset voltage (Vonset). In the two concentric cylinder conduction configuration, the corona onset voltage could be calculated as follows (Kaiser Citation2006):[7] where Emax is the breakdown electrical strength of the air (= 3.1 × 10−6V/m), and Din and Dout are the inner and outer diameters, respectively. The corona onset voltage calculated by Equation (Equation7
[7] ) was 14 kV. Thus, the voltage applied to the ESP was set at 7 kV to capture the charged particles without air ion generation. For the case of mono-disperse particles, three different particle charge states were tested: a singly charged state, a neutralized state, and an uncharged state. A differential mobility analyzer (DMA, Model 5.700, Grimm, Ainring, Germany) with an aerosol neutralizer (Am241, Model 5.620, Grimm) were used to classify 100 nm NaCl particles with a −1 unit charge. After that, the charge states of the mono-disperse particles were controlled as follows (). Along route III: singly charged mono-disperse particles obtained from DMA were used to represent singly charged particles. Along route IV: singly charged mono-disperse particles exiting the DMA were passed through a Kr-85 aerosol neutralizer to condition their charge state to a Boltzmann equilibrium charge distribution. Along route V: neutralized mono-disperse particles obtained from route IV were passed through the ESP to remove the charged particles. For convenience, the particles passing through route III, route IV, and route V are denoted as “singly charged,” “neutralized,” and “uncharged,” respectively.
2.5. Filter test
The particle concentrations upstream and downstream of the filter were measured using a scanning mobility particle sizer (SMPS), which was composed of a classifier controller (3080, TSI Inc.), a differential mobility analyzer (DMA, 3081, TSI Inc.), a condensation particle counter (CPC, 3025A, TSI Inc.), and an aerosol neutralizer (3770A, TSI Inc.). The sampling times of each measurement, and the sheath and sampling flow rates of the SMPS, were fixed at 180 s, 3 L/min, and 0.3 L/min, respectively. The collection efficiency (ηcollect) of the filter was calculated using the measured particle number concentrations upstream (Cin) and downstream (Cout) of the filter, as follows:[8]
In the case of the mono-disperse particle tests, particles in a size range of 98.2–102 nm were used to calculate the efficiency.
3. Results and discussion
3.1. Collection efficiency for poly-disperse particles
The particle concentration and mode diameter of neutralized poly-disperse particles generated by route I were approximately 2.5 × 105 particles/cm3 and 71 nm, respectively, while those of uncharged poly-disperse particles generated by route II were approximately 1.3 × 105 particles/cm3 and 66 nm. The collection efficiencies of filter A and filter B for poly-disperse particles are shown in and , respectively. In these figures, the particle diameter on the x-axis is the mobility diameter of the particle, which is generally used in SMPS. Filter A had a typical filtration characteristic in that its efficiency curve was U-shaped. The minimum efficiency of filter A was measured as approximately 80% at dp = 85 nm for neutralized particles and approximately 63% at dp = 210 nm for uncharged particles. When the external electric field was applied to the filter, the collection efficiency of filter A increased from 85.0% to 88.2%, while the efficiency of filter B increased from 63.4% to 73.1%. The external electric field did not have a significant effect on the collection efficiency for uncharged particles.
Figure 4. Collection efficiencies of (a) filter A, (b) filter B, (c) filter C, and (d) filter D for poly-disperse NaCl particles with different charge states at external electric voltage V0 = 0 V (filled symbol) and V0 = 1000 V (open symbol).
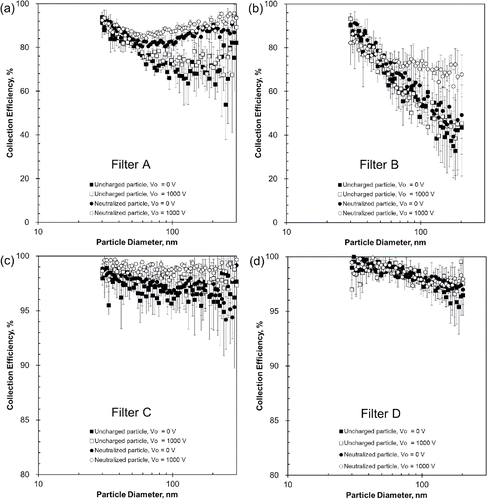
A comparison of the collection efficiencies of filters A and B for uncharged particles showed that the collection efficiency of filter A was higher than that of filter B, regardless of the application of the external electric field (square symbols in and ). This indicated that the effect of the polarization force induced by the charged filter fiber was a dominant factor in the electrical filtration mechanism for uncharged particles. Moreover, the polarization effect became more significant as the particle size increased, because the magnitude of the force was proportional to the cube of the particle size, as shown in the following equation (Brown Citation1993):[9] where Q is the unit length charge of the fiber, and r is the distance between the center of the particle and the central axis of the fiber. Generally, filter charge Q has an order of magnitude in the hundreds. Thus, the external electric field effect on the charged fiber filter was negligible compared to the polarization effect induced by the charged filter fiber, because the value of QdfE is much smaller than that of filter charge Q.
The effect of the filter fiber charge state on the neutralized particles could be found by comparing the collection efficiencies of filter A and filter B under no external electric field conditions (filled circular symbols in and ). The condition in represents the Coulombic force between the neutralized particles and charged filter fibers, while the condition in represents the polarization force imposed by the neutralized particles. Without the external electric field, the difference between the collection efficiencies of filter A and filter B was 21.1 %p. When the external electric field was applied (open circular symbols in and ), the collection efficiency of filter A increased by 3%p., while the efficiency of filter B increased by 10%p. There was no significant change to the collection efficiency of filter B caused by the particle charge state under no external electric field condition. This indicates that the neutralized particles do not lead to the polarization of neutralized filter fiber, and the application of an external electric field can cause the Coulombic force between neutralized particles and filter fibers, filter B. In addition, the effect of the Coulombic force caused by charged filter fibers is superior to that imposed by the external electric field when the filter fiber is in an electrical charge state. Consequently, the application of external electric fields leads to significant increase in the collection efficiency of a neutralized filter for atmospheric particles with a Boltzmann equilibrium charge distribution, but is not helpful in increasing the collection efficiency when the filter fiber is charged, since the major electrical filtration mechanism of the charged fiber filter is the Coulombic force imposed by charged fiber regardless of the application of an external electric field.
In order to investigate the effect of the mechanical collection efficiency of the filters, a commercial high-efficiency particulate air (HEPA) filter (filter C) and a laboratory-made electrospun filter (filter D) were used as test filters (). and show the collection efficiencies of filter C and filter D, respectively. Because filters C and D did not have electric charges on the fibers, the electrical effects on the particles entering those filters were the Coulombic and polarization forces caused by an external electric field. There was no significant effect from an external electric field on the collection efficiencies of filter C and filter D. Comparing the efficiencies of filter C and filter D with those of filter B, which has 50% of collection efficiency for neutralized particles in MPPS range, it is clear that the improvement in the collection efficiency due to the external electric field is prominent when the filter has a low-mechanical collection efficiency. However, it should be noted that the external electric field affected the collection efficiencies of filter C and filter D for neutralized particles, although the effect seemed to be negligible.
3.2. Collection efficiency for mono-disperse 100 nm particles
In order to investigate the effect of external electric fields on the collection efficiency of filters based on the particle's charge level, three different charge states for particles were tested (). The particle concentrations of singly charged (route III), neutralized (route IV) and uncharged (route V) mono-disperse particles with a diameter of 100 nm were approximately 6.3 × 103, 3.7 × 103 and 3.2 × 103 particles/cm3, respectively. summarizes the particle collection efficiencies in relation to the particle charge state and presence of an external electric field. The particle collection efficiencies of filter A and filter B for uncharged and neutralized 100 nm particles were almost the same as the results obtained by the poly-disperse particle test. There was a prominent increase in the collection efficiency of Filter A when the particles had a charge, but the effect of the external electric field was negligible. In the case of filter B, no significant differences were observed in the collection efficiency under various particle charge states without the external electric field, but the electric field led to dramatic improvement in the collection efficiency when the particles had a charge. More specifically, for singly charged particles, the collection efficiency of filter A increased by approximately 13%p. compared to that for the neutralized particles regardless of the presence of an external electric field. On the other hand, the collection efficiencies of filter B increased by approximately 20%p. compared with those for the neutralized particles when the external electric field was applied. Consequently, it was reaffirmed that the Coulombic force of the charged filter fiber was dominant for filter A, while the Coulombic force imposed by the external electric field was a dominant factor for filter B. The collection efficiency of filter D for 100 nm particles listed in shows the same trend as in . In other words, the charge state of the particles affected the collection efficiency under the external electric field, even though the effect was not significant.
Table 2. Collection efficiencies of tested filters for 100 nm particles under various electrostatic conditions.
3.3. Theoretical analysis
The dominant electrical filtration mechanisms of filters A and B were well identified by the experiment results. However, it was hard to determine the major effect of an external electric field on the filter D, which has a high mechanical collection efficiency, since the differences in the collection efficiencies obtained under each experimental condition were not clear. For this reason, theoretical analysis of filter B and filter D was conducted. The collection efficiencies of filter B and filter D with and without an external electric field were calculated using Equations Equation(1)–(6), as well as equations for the mechanical filtration mechanisms (not shown in this manuscript). To verify our theoretical analysis, the calculated results were compared with the experimental results (). The theoretically calculated results well reflected the experimental results under various conditions. shows the calculated collection efficiencies of filters B and D for uncharged and charged particles under the presence and absence of an external electric field. In these figures, the collection efficiency without the external electric field for uncharged particles can represent the effect of the mechanical filtration mechanism, while the efficiency for singly charged particles with and without the electric field represents the complex effects of the Coulombic and polarization forces and the effect of the polarization force, respectively. For filter B, the effect of the polarization force was negligible and the enhancement of the collection efficiency was caused by the Coulombic force, while both of these forces affected the particle capturing for filter D. This phenomenon could have resulted from the difference between the mechanical collection efficiencies of the two filters. The collection efficiency of filter D was much higher than that of filter B, regardless of the external electric field. This meant that the space between the filter fibers of filter D was much narrower than that of filter B. Thus, the distance between the filter fiber and the charged particle inside the filter medium was relatively small. As shown in Equation (Equation9[9] ), the polarization force obtained by an external electric field was inversely proportional to the cube of the distance between the particle and the filter fiber. However, the Coulombic force was dependent on the magnitude of the external electric fields, which may be uniform inside the filter medium. For this reason, for filter B, which had low mechanical collection efficiency, the Coulombic force was the dominant factor as the electrical filtration mechanism, while both the Coulombic force and polarization force affected the enhancement of the collection efficiency of filter D.
4. Conclusion
In this study, the effects of the presence of an external electric field on the collection efficiency of a filter were examined. The dominant filtration mechanism under each filtration condition was also investigated. The effects of a filter's mechanical collection efficiency and particle and filter fiber charge states on the collection efficiency of the filter with the external electric field were evaluated, and major electrical filtration mechanisms at each condition were analyzed using experimental and theoretical approaches.
For the charged fiber, the polarization force imposed by the charged fiber was the dominant factor in the enhancement of the collection efficiency. When an external electric field was applied to the filter, the Coulombic force also led to an increase in the collection efficiency for charged particles, but the polarization force was still superior. Consequently, the increase in the collection efficiency of the charged fiber filter caused by the external electric field was insignificant.
For the neutral fiber filter, an improvement in the collection efficiency by an external electric field was observed when the test particles were charged or neutral. When the filter had low mechanical collection efficiency, the Coulombic force was the dominant factor. For the high-grade filter, both the Coulombic and polarization forces affected the enhancement of the collection efficiency in the high-grade filter, but the effects were insignificant. Consequently, the external electric field greatly influenced the collection efficiency of the neutralized fiber filter having low mechanical collection efficiency. However, the effects were insufficient for the high-grade filter and charged fiber filter.
Because the dominant factor in the enhancement of the collection efficiency of the low-grade filter was the Coulombic force, the strength of the external electric field and charge number of the particles are key factors for improving the collection efficiency. Thus, the effect of the external electric field may be improved with a technique in prevention of an electrical short, which can occur between two electrodes. Moreover, particle charging technologies such as a unipolar charger and an air ionizer can be installed in front of the filter to increase the charge number of the particle. In the case of a high-grade filter, which is affected by both the Coulombic and polarization forces, a decrease in fiber diameter can also be considered as a means of increasing polarization force (Equation (Equation4[4] )). However, it should be noted that all of the experiments in this study were conducted using pristine filter media. In other words, the effect of dust loading on the collection efficiency was not considered. If particles are deposited on the filter, the loaded particles on the filter surface could affect the electric field inside the filter and therefore the collection efficiency of the filter. In our future studies, experiments will be needed to investigate the effects of the particle loading amount, and the particle's physical, chemical, and electrical characteristics, on the collection efficiency.
UAST_1361014_Supplementary_Information.zip
Download Zip (31.1 KB)Funding
This work was supported by Korea Ministry of Environment (MOE) as “Eco-innovation Program.”
References
- Ahn, Y. C., Park, S. K., Kim, G. T., Hwang, Y. J., Lee, C. G., Shin, H. S., and Lee, J. K. (2006). Development of High Efficiency Nanofilters Made of Nanofibers. Curr. Appl. Phys., 6:1030–1035.
- Brown, R. C. (1993). Air Filtration: An Integrated Approach to the Theory and Applications of Fibrous Filters. Pergamon Press, Oxford.
- Chazelet, S., Bemer, D., and Grippari, F. (2011). Effect of the Test Aerosol Charge on the Penetration Through Electret Filter. Sep. Purif. Technol., 79:352–356.
- Chuaybamroong, P., Chotigawin, R., Supothina, S., Sribenjalux, P., Larpkiattaworn, S., and Wu, C. Y. (2010). Efficacy of Photocatalytic HEPA Filter on Microorganism Removal. Indoor Air, 20:246–254.
- Davies, C. N. (1973). Air Filtration. Academic Press, London.
- Donovan, R. P. (1985). Fabric Filtration for Combustion Sources. Marcel Dekker, New York.
- European Committee for Standardization (CEN). (1998). Standard EN1822-1: High Efficiency Air Filters (HEPA and ULPA)-Part 1: Classification, performance testing, marking. CEN, Brussels, Belgium.
- European Committee for Standardization (CEN). (2012). Standard EN779: Particulate Air Filters for General Ventilation- Determination of the Filtration Performance. CEN, Brussels, Belgium.
- Fisk, W. J., Faulkner, D., Palonen, J., and Seppanen, O. (2002). Performance and Costs of Particle Air Filtration Technologies. Indoor Air, 12:223–234.
- Kao, J.-N., Tardos, G. I., and Pfeffer, R. (1987). Dust Deposition in Electrostatically Enhanced Fibrous Filters. IEEE Trans. Ind. Appl., IA-23:464–473.
- Kaiser, L. K. (2006). Electrostatic Discharge. Taylor & Francis, Florida.
- Li, L., Zuo, Z., Japuntich, D. A., and Pui, D. Y. H. (2012). Evaluation of Filter Media for Particle Number, Surface Area and Mass Penetrations. Ann. Occup. Hyg., 56:581–594.
- Liu, B. Y. H., and Pui, D. Y. H. (1974). Equilibrium Bipolar Charge Distribution of Aerosols. J. Colloid Interface Sci., 49:329–333.
- Luckner, H. J., Gradoń, L., Podgórski, A., and Wertejuk, Z. (1998). Aerosol Particle Filtration in the Fibrous Filters at the Presence of External Electric Field. II. Experimental Investigations. Inż. Chem. Proc., 19:891–903.
- Park, H.-S., and Park, Y. O. (2005). Simulation of Particle Deposition on Filter Fiber in an External Electric Field. Korea J. Chem. Eng., 22:303–314.
- Park, J. H., Yoon, K. Y., Na, H., Kim, Y. S., Hwang, J., Kim, J., and Yoon, Y. H. (2011). Fabrication of a Multi-Walled Carbon Nanotube-Deposited Glass Fiber Air Filter for the Enhancement of Nano and Submicron Aerosol Particle Filtration and Additional Antibacterial Efficacy. Sci. Total Environ., 409:4132–4138.
- Plopeanu, M. C., Notingher, P. V., Dumitran, L. M., Tabti, B., Antoniu, A., and Dascalescu, L. (2011). Surface Potential Decay Characterization of Non-Woven Electret Filter Media. IEEE Trans, Dielectr, Electr. Insul., 18:1393–1400.
- Podgórski, A., Luckner, H. J., Gradoń, L., and Wertejuk, Z. (1998). Aerosol Particle Filtration in the Fibrous Filters at the Presence of External Electric Field. I. theoretical model. Inż. Chem. Proc., 19:865–889.
- Raynor, P. C., and Chae, S. J. (2004). The Long-Term Performance of Electrically Charged Filters in a Ventilation System. J. Occup. Environ. Hyg., 1:463–471.
- Rengasamy, S., Miller, A., Vo, E., and Eimer, B. C. (2013). Filter Performance Degradation of Electrostatic N95 and P100 Filtering Facepiece Respirators by Dioctyl Phthalate Aerosol Loading. J. Eng. Fiber Fabr., 8:62–69.
- Romay, F. J., Liu, B. Y. H., and Chae, S.-J. (1998). Experimental Study of Electrostatic Capture Mechanism in Commercial Electret Filters. Aerosol Sci. Technol., 28:224–234.
- Sim, K. M., Park, H. S., Bae, G. N., and Jung, J. H. (2015). Antimicrobial Nanoparticle-Coated Electrostatic Air Filter with High Filtration Efficiency and Low Pressure Drop. Sci. Total Environ., 533:266–274.
- Thorpe, A., and Brown, R. C. (2003). Performance of Electrically Augmented Fibrous Filters, Measured with Monodisperse Aerosols. Aerosol Sci. Technol., 37:231–245.
- Walsh, D. C., and Stenhouse, J. I. T. (1997). The Effect of Particle Size, Charge, and Composition on the Loading Characteristics of an Electrically Active Fibrous Filter Material. J. Aerosol. Sci., 28:307–321.
- Yang, S., Lee, W.-M. G., Huang, H.-L., Huang, Y.-C., Luo, C.-H., Wu, C.-C., and Yu, K.-P. (2007). Aerosol Penetration Properties of an Electret Filter with Submicron Aerosols with Various Operating Factors. J. Environ. Sci. Heath A, 42:51–57.
- Yeh, H. C. (1976). Theoretical Study of Electrical Discharging of Self-Charging Aerosols. J. Aerosol Sci., 7:343–349.