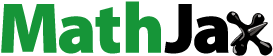
Abstract
The goal of this study is to evaluate the filtration efficiency and breathability of improvised filtration and commonly available mask materials, as well as to assess their reusability. Materials readily available to the general public such as cotton, fragrance and additive-free dry baby cleaning wipes, and those abundantly available in the hospital setting, such as sterilization wraps, were chosen for testing, amongst others. In the COVID-important 2–5 µm particle range, two-layers of cotton provided filtration efficiency between 34%–66%. Amongst potential filter materials, 300-weight sterilization wraps provided approximately 80% filtration efficiency and are readily available in the healthcare setting. The addition of sterilization wrap to cotton fabrics brought the filtration efficiency to above that of the sterilization wrap (80%–90%) at the expense of added pressure drop. Four-layers of dry baby wipes performed very well with a filtration efficiency of 85% and a reasonable pressure drop (1/3 of procedure mask). Since the material is advertised as pure spunlace polypropylene and designed to contact the skin during cleaning, it would appear generally safe as a filter insert. Of improvised filters, polypropylene electrostatic HVAC filters performed the best with filtration efficiencies of >99%, but are not recommended due to the risk of confusion with glass-based HVAC filters and uncertainty regarding trace materials used in the filter. The filtration efficiency of two-layers of cotton fabrics with one-layer of sterilization wrap slightly improved over 10 laundry cycles, while the performance of other non-wovens, like dry baby wipes, degraded more rapidly and should be considered disposable. In summary, we found that two-layers of cotton fabric can provide a comfortable, breathable and reusable option. The addition of a sterilization wrap or four-layers of pure spunlace fragrance free dry baby wipes can significantly improve filtration and block expiratory aerosols at the expense of an added pressure drop.
Copyright © 2021 American Association for Aerosol Research
EDITOR:
Introduction
SARS-CoV-2, the virus that causes COVID-19 is spreading within the human population through expiratory aerosols and droplets from infected individuals (“Modes of transmission of virus causing COVID-19” Citationn.d.; Furukawa, Brooks, and Sobel Citation2020). Individuals are thought to be most contagious when they are symptomatic. However, people may be contagious when they are pre-symptomatic during the incubation period of approximately 5 days (median, 5.1 days; 95% CI: 4.5–5.8 days) (Lauer et al. Citation2020). Analysis of cases in Wuhan has shown that as many as 86% of people infected with COVID-19 did not present symptoms, but more than half of them were contagious (55%). That contagious population resulted in 79% of new infections (Li et al. Citation2020). Breathing naturally, speaking, shouting, singing and coughing generate respiratory droplets that can transmit COVID-19 (Hamner Citation2020; Asadi et al. Citation2019).
The use of face masks primarily serves to protect others by reducing the number of expiratory droplets and aerosolized particles that may contain COVID-19. Although the mechanisms of COVID-19 spread continue to be investigated, patients sick with four common non-COVID-19 coronaviruses, exhaled virus containing droplets with a mean diameter of 5 µm (Leung et al. Citation2020). Moreover, procedure masks block four types of non-COVID-19 coronavirus exhalations in actively sick patients (Leung et al. Citation2020). Additionally, studies have shown that the most common droplet size threshold has a minimum of 5 to 10 μm (Howard et al. Citation2020; Morawska et al. Citation2009; Duguid Citation1946). So, capturing particles in the smaller 2–5 μm range before these droplets become aerosol particles due to drying is advantageous. If the large majority of the general population have access to comfortable, breathable and reusable face masks that can block expiratory aerosols and droplets, the wearers could potentially protect the public and reduce the spread of the virus (Eikenberry et al. Citation2020; van Doremalen et al. Citation2020; Wang and Du Citation2020).
Community protection provided by face masks from COVID-19 is based upon both the efficiency of the face masks at reducing the number of expiratory droplets and aerosolized particles and the percent of the total number of people in that community wearing a face mask. A study of SARS-CoV-1 patients in Hong Kong determined that frequent use of masks in social settings lowered the risk of transmitting the virus by 64% (Lau et al. Citation2004) making a strong case for universal use of face masks during the current pandemic (Eikenberry et al. Citation2020; Feng et al. Citation2020). Coupled into this benefit is the bidirectional filtration of a mask, providing community protection by filtering exhalation, and self-protection by filtering inhalation.
Three organizations within the United States regulate the requirements for face masks. Whereas the Centers for Disease Control and Prevention/National Institute for Occupational Safety and Health (CDC/NIOSH) detail tests that qualify particulate respirators (e.g., N95, N99, N100) (“CDC − 42 CFR Part 84 Respiratory Protective Devices” Citation2020), the American Society for Testing and Materials (ASTM) and the Food and Drug Administration (FDA) detail standards to qualify surgical masks (“ASTM Standards & COVID-19” Citationn.d.). Based on ASTM F2100, medical face mask material performance is established via testing for bacterial filtration efficiency, differential pressure, sub-micron particulate filtration efficiency, resistance to penetration by synthetic blood, and flammability (“ASTM Standards & COVID-19” Citationn.d.). However, the FDA Guidance for face masks during the COVID-19 public health emergency has waived compliance to such regulatory requirements for “medical face masks that are NOT Intended to Provide Liquid Barrier Protection”, as long as no claim is made regarding particulate filtration efficiency, antimicrobial or antiviral protection of such face masks (Center for Devices and Radiological Health Citation2020; F23 Committee Citationn.d.).
The ideal mask during this COVID-19 pandemic would have high filtration efficiency, low pressure drop or flow resistance, and would be washable and reusable. The goal of this study is to quantify the filtration and breathability of different commonly available face mask materials, along with their reusability. Our tested materials were drawn from those available to the general public and within hospitals. Filtration Efficiency (FE) and resistance to flow were measured. This information is anticipated to add to the understanding of different improvised mask materials, especially by studying the reusability of improvised materials for masks.
Prior works on improvised materials found that common fabrics (sweatshirts, t-shirts, towels and scarfs) can block 10–60% percent of particles in the 0.2–1 µm range (Rengasamy, Eimer, and Shaffer Citation2010) and improves to 90% for the larger particle ranges (>1 µm) due to being in the inertial impaction regime (Konda et al. Citation2020). Other studies have since examined various non-fabric filters, including HVAC filters, vacuum bags, tea towels, and sterilization wraps (300-wt) and found filtrations of between <60–87% at 0.3 um, 5–75% at 0.3 um, 36% at 2.75 um and 95% at 2.75 um, respectively (Pei et al. Citation2020; Rogak et al. Citation2020; Davies et al. Citation2013).
Experimental methods
Face masks and filter inserts
We studied the FE and the breathability of single and multiple layers of different materials. Because face masks intended for use by the general public should be durable and washable, 100% cotton fabric was chosen for the inner and outer layers. High thread count, tight weave and reasonable weight, characterize a cotton mask that is effective in both particulate filtration and breathability. Table S1 highlights all the different cotton fabrics chosen for the testing. The cotton fabric was tested both in single-layer and in dual-layer (see Figure S1). The WHO recommends a combination of materials that includes a hydrophilic inner layer and a hydrophobic middle and outer layer (“When and how to use masks” Citationn.d.). Hydrophilic layers can better capture water droplets, while hydrophobic layers can increase comfort and provide liquid barrier protection. It is not our intention to create medical face masks that meet liquid barrier protection (e.g., protection against liquid blood squirts). While measuring the contact angle of these materials is outside the scope of this study, the cotton fabrics were all hydrophilic, and the polymeric filter materials (PP & PET) were hydrophobic.
Figure 1. (a) Schematic of the filtration efficiency test apparatus. (b) Schematic of the pressure drop test apparatus.
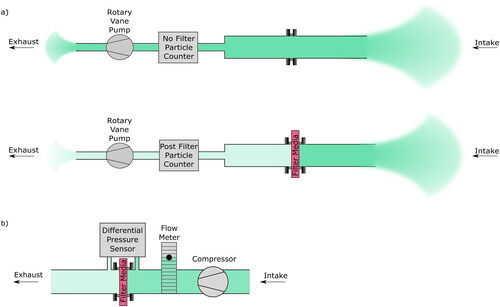
Table 1. Summary of cotton fabrics.
Improvised face masks can be designed to accommodate a permanent or removable nosepiece and filter insert. Various filter media were sourced and tested to assess their FE scores and their breathability ( and S1). Nonwoven polypropylene, as used in N95 respirators and HVAC filters, is a commonly used filter medium and it can be meltblown into fine fibers. In addition, nonwoven polypropylene’s large fiber surface area can be charged during manufacturing to electrostatically trap and/or mechanically filter particles. The high demand for N95 masks has disrupted the manufacturing stream of polypropylene filter material during the COVID-19 pandemic. While nonwoven polypropylene is commonly used in numerous everyday residential and medical applications (baby wipes, household HVAC filters, medical sterilization wraps, reusable grocery bags), and can be easily sourced and repurposed, many lack the electret charge that contributes to their high filtration capabilities. Some of these readily available materials may undergo intentional charging (e.g., polymeric HVAC filters), while other consumer products may undergo anti-static processing and/or contain anti-static additives. Materials chosen include those with unproven biocompatibility like unscented dry floor wipes and HVAC filters (polymeric, non-glass) amongst others (Table S1) as well as generally regarded as biocompatible materials like sterilization wraps (SW300) and fragrance and additive free baby wipes. These materials intended for filter inserts were tested in single-layer and when appropriate based on the pressure drop, in multiple-layers. Both the face mask and filter insert materials were tested for particulate filtration efficiency, breathability and reusability.
Setup for filtration particle count
The test used in this study to determine particulate filtration efficiency of face mask materials was adapted from ASTM F2299 (F23 Committee Citationn.d., 23) (). The FE was measured at a face velocity of 2 cm/s (within velocity range specified by ASTM F2299 of 0.5–25 cm/s). Increasing the face velocity results in higher FE for larger particles (>∼0.5 μm) due to increased deposition by impaction, so the 2 cm/sec face velocity will provide a conservative FE for the 2–5 μm range most of interest. For smaller particles (<∼0.5 μm), the trend is opposite, with slower particles having higher FE at lower speeds due to greater time for particulate diffusion and adsorption onto the filter (Drewnick et al. Citation2021). Air is drawn through the filter media via a high-speed rotary pump (Thomas, G3/04EB). The number of particles before and after the sample filter was sensed with a 6-bin particle analyzer (HAL-HPC60, Hall Technology, Fontana, CA, USA).
All the samples considered for the experiment were tested four times, each with a 3-min observation of ambient air, filtered air and ambient air, in that order (12 min for each). The number of ambient air particles counted between 0.3–0.5 µm, 0.5–1.0 µm, 1–2 µm, 2–5 µm, and 5–10 µm, >10 µm over a 12 min observation were approximately 270,000, 40,000, 7000, 4650, 2550, 1000 particles, respectively.
Filtration efficiency was calculated by comparing the quantity of particles in the incoming air to the quantity of particles in the post-filtered air according to the following equation:
The uncertainty in the mean was calculated using a 95% two-tailed confidence interval based on a student's t-distribution. The confidence limits for FE were calculated via two methods, and the greater of the two has been reported: (1) A student's t-distribution was used to estimate the confidence limit as where
is the measured mean FE, n is the number of observations, and t is the student's t-distribution statistic based on n-1 degrees of freedom. (2) The arrival of particles were assumed to obey a Poisson distribution, where a 95% confidence interval in the number of particles pre-filter and post-filter are
and lower confidence limit
where C is the observed number of counts,
is the confidence interval, and
is the Chi-squared function (O'Shaughnessy and Schmoll Citation2013).
The challenge particles for these experiments are naturally occurring particles in indoor ambient air. Although ambient air provides less control and standardization over the input particle size and composition compared to latex or NaCl challenge particles (“CDC − 42 CFR Part 84 Respiratory Protective Devices” 2020; F23 Committee Citationn.d., 42), the filtration of naturally occurring particles should be a representative placeholder for respiratory particles and is widely used for quantitative mask fitting (e.g., TSI PortaCount). Filtration tests with latex microparticles (1.1 µm) were performed on select samples and compared with the naturally occurring aerosol measurements. The ambient particle FE percent error relative to the latex challenge particles was 0.03% for the N95 and 5.5% for the SW300 at 1–2 µm (see SI for complete details).
Resistance to flow
While a relatively slow face velocity was used to obtain conservative filtration measurements, a faster velocity of 6.8 cm/s, representative of breathing through mask, was used to measure resistance to flow (Tang et al. Citation2013) (). The resistance to flow of the fabrics was assessed by sending air from a dry diaphragm compressor (DOA-P704, Gast Manufacturing, Inc., Benton Harbor, MI, USA) across a 19.5 cm2 circular sample, while measuring the pressure drop with a differential pressure sensor (ADM-850L, Shortridge Instruments, Inc., Scottsdale, AZ, USA). The flow was controlled using a flowmeter/controller (RMA-22, Dwyer Instruments, Inc., Michigan City, IN, USA) at 8.0 ± 0.5 L/min. All the samples had their pressure drop measured at least 10 times, 1 s apart. The uncertainty of the pressure measurements was estimated with where
is the standard deviation and n is the number of measurements. The percentage uncertainty for all masks was less than 4%.
Reusability
Samples were washed and dried in residential clothes laundries by four individuals using their choice of detergent, wash cycle, and dryer cycle, except for the dry baby wipes, dry floor wipes, and HVAC filters, that were washed by one individual. The washing procedure follows the World Health Organization and the Centers for Disease Control recommendation that masks for laypeople be disinfected using household laundry (CDC and http: Citation2020a; “When and how to use masks” Citationn.d.). It is well known that the surfactancy of soap can inactivate the capsid coating of viruses (Liu et al. Citation2010; Grayson et al. Citation2009; Contreras et al. Citation1999). Samples were then tested for particulate filtration efficiency after 5 and 10 cycles. The resistance to flow was re-assessed after the materials were washed.
Results
We assessed the FE in ambient air of selected cotton fabric and improvised nonwoven polymer filters (Table S1). Materials were chosen based on the availability to the general public and within hospitals, the recommendations of the CDC (Citation2020b) relative durability and reusability.
presents cotton fabrics and other biocompatible filter materials tested while presents the tested filter materials of unproven biocompatibility. All the materials tested are readily available in the hospital setting and/or to the public. To capture the variability for each category of materials, the maximum to minimum FE ranges are presented against particle size for single and double-layer cotton fabrics, single-layer sterilization wrap (CH300; Cardinal Health, Inc., Dublin, OH, USA), fragrance and additive free dry baby wipes (Medline Ultrasoft1013), unscented dry floor wipes (Swiffer, P&G, Cincinnati, OH, USA), and polymeric HVAC (Heating, Ventilation, and Air Conditioning) filters (3 M FiltreteTM 1500 & 1900, St. Paul, MN, USA). presents the FE range of all tested materials across the broad particle size of 0.3–10.0 µm.
Figure 2. Comparison of filtration efficiency vs particle bin size for (a) single-layer cotton fabrics, sterilization wrap material samples (SW300), and multiple layers of spunlace polypropylene (fragrance and additive free dry baby wipes), and (b) for various commonly available materials with unproven biocompatibility (polymeric HVAC filters, dry floor sweeping wipes). Measurements were performed at a face velocity of 2 cm/s. Numbers next to the material refers to the number of layers. Refer to for additional information on the materials.
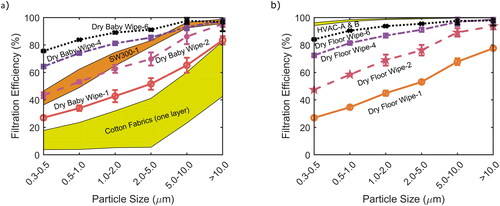
The range of FE for single-layer cotton fabrics varied across different particle sizes (). The performance was thought to be influenced by fabric weight, thread count and variable porosity of fabric weaves. Though trends were not readily visible, a recent study by Kondo et al. suggested that cotton performs better at higher thread count (Konda et al. Citation2020). The FE of these fabrics increased with increasing particle size because the capture physics are in the inertial impaction and interception regime (Konda et al. Citation2020; Davies et al. Citation2013; Rengasamy, Eimer, and Shaffer Citation2010; Hinds Citation1999). Similar trends were also observed when the cotton fabric was tested in double-layers ().
Figure 3. Comparison of filtration efficiency vs particle bin size for two layers of cotton and combinations of cotton with a sterilization wraps (SW300), four layers of dry baby wipes (Medline Ultrasoft1013), or two layers of dry floor wipes, as well as an N95 Respirator (3 M Particulate Respirator 8210) and an ASTM F2100-11 Class-1 Procedure Mask (McKesson Procedure Mask Earloop, MFR# 91-2002).
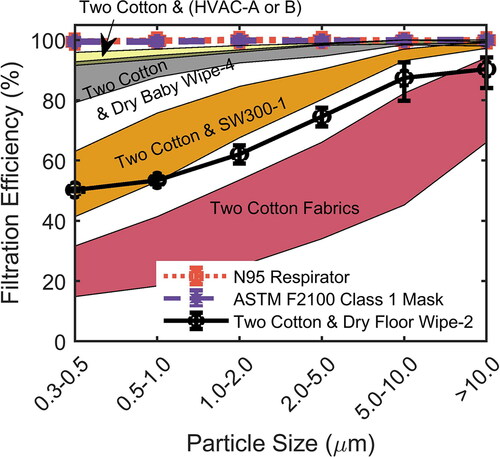
The FE for a single-layer of improvised filter material (SW300) was higher than the cotton fabrics tested. The FE of dry baby wipes was also significantly higher than the single-layer cotton fabric and the FE continued to increase with subsequent increase in the layers of material (; ). Amongst the unproven biocompatible filter materials, HVAC filters had the highest FE, followed by unscented dry Swiffer floor wipes ().
Table 2. FE’s of all materials tested (not stratified), * indicates unproven biocompatibility.
illustrates the filtration efficiency stratified by particle size for various combinations of two cotton layers by themselves, two cotton layers with a sterilization wrap, two layers of cotton with four-layers of dry baby wipes, as well as for N95 respirator (3 M Particulate Respirator 8210) and procedure mask (McKesson Procedure Mask Earloop (MFR# 91-2002) ASTM F2100-11 Level-1). FE was tested for two cotton layers (representing a cotton face mask) and two cotton layers with one filter layer (SW300, representing a cotton face mask with a filter). The FE with two cotton layers improved compared to single-layer cotton fabric. The performance of two cotton layers varied widely. The addition of any of the tested filter materials to two cotton layers increased the FE significantly in comparison to two cotton layers alone. The N95 respirator and procedure masks exhibited >99% FE at all evaluated particle bin sizes. At smaller than tested particle bins (<0.3 µm), the filtration efficiency should increase, as diffusional filtration becomes more efficient at smaller particle sizes (Balazy et al. Citation2006).
illustrates the filtration efficiency and breathability of various mask and filter combinations, in the form of plots of FE versus drop in pressure exiting the mask (face velocity) at particle bin sizes of 2.0–5.0 µm (where the FE was taken at 2 cm/s and the pressure drop was taken at 6.8 cm/s face velocities). The dashed lines show constant quality factors (Rogak et al. Citation2020; Huang et al. Citation2013). The FE versus pressure drop for other bin sizes is included in (Figure S1). Of interest, the increase in performance when sterilization wrap is added to the highest FE cotton pair vs the lowest FE cotton pair is modest and at the expense of breathability (pressure drop). Moreover, the filtration efficiency per unit pressure drop is greater for the filter materials than the cottons. The pressure drop, filtration and comfort of these materials in a mask depends on the particular mask design and its fitment to the user, which are outside the scope of this study. Nonetheless, these materials will have lower FE and lower pressure drop due to the leakage. The work of Davies et al. (Citation2013) establish that procedure masks have a leakage of about 20% and improvised masks will likely be worse.
Figure 4. Representation of FE vs pressure drop at particle bin sizes of 2.0–5.0 µm, with the FE measured at a face velocity of 2 cm/s, and pressure drop measured at a face velocity of 6.8 cm/s; Unproven biocompatible materials are denoted by red asterisks (*). Figure S1 shows the results for other bin sizes from 0.3–10 µm. Refer to Table S1 for details on the materials, including microscopy.
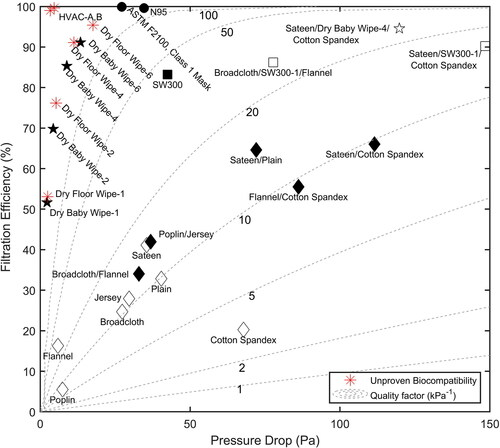
FE results of various unwashed, and washed and dried samples are illustrated in which shows similar progression in filtration efficiency for cotton samples with one filter layer. Noteworthy is that larger FE was noted for sterilization wrap with two-layers of cotton after 10 washing and drying cycles. A comparison of the pressure drop in washed and unwashed samples (Table S2) shows no significant change in the breathability of woven and non-woven materials with washing and drying.
Figure 5. Comparison of filtration efficiency change vs particle bin size for various unwashed, and washed and dried fabric samples; each sample contained two-layers of cotton fabric and one-layer of 300 weight sterilization wraps.
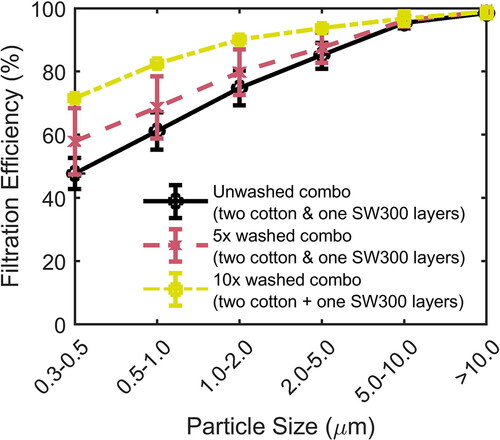
The washing study for the baby wipes, polymeric HVAC filters, and dry floor wipes was performed only in one household’s washer and dryer, so it has lower statistical power, however, some useful observations were made in this limited study (Figure S2). Electrostatic polymeric HVAC filters reduced performance by nearly half at the smallest particle size upon washing. Four-layers of baby wipes (Medline dry cleaning wipes, ULTRASOFT1013) diminished in performance after 5 cycles, and the HVAC Filters, dry baby wipes, and unscented floor wipes all degrade significantly by 10 cycles, so they should be considered disposable.
Discussions
The present need for acceptable materials for use in face masks worn by the general public and by medical workers is unprecedented. The materials must adequately filter expiratory aerosols and droplets that might contain SARS-CoV-2 and, by so doing, decelerate the spread of COVID-19. Optimal materials have high particulate filtration efficiency and—ideally—are comfortable, are washable without filtration degradation, and have good breathability.
The expected shortage of such “filtering facepiece respirators” in the event of a pandemic was articulated 10 years ago by Rengasamy, Eimer, and Shaffer (Citation2010) where they tested improvised materials (cloth masks, sweatshirts, t-shirts, towels and scarfs), and the values generally agree with our observed values for cloth masks. Rengasamy et al. found filtration efficiencies for fabric materials of 3–33% at the 1 µm particle size, which agrees relatively well with our results. Recently, Konda et al. (Citation2020) found very good filtration with some cotton fabrics at small particle sizes, i.e., <5 µm (many FE >90% in the 1–5 µm range), unlike our tests. Our testing focused on readily available cotton fabrics with relatively low thread counts that demonstrated good qualitative breathability and washing and drying performance, but demonstrated lower filtration performance for small aerosols (<5 µm). This accounts for the variability seen with the different cotton fabrics used in our testing.
Charged meltblown polypropylene (1–1.5 µm diameter) is the primary filtration layer for medical-grade masks, as the trapped electrostatic charge, called an electret, helps capture particles and aerosols (Tsai, Schreuder-Gibson, and Gibson Citation2002; Tsai and Wadsworth Citation1995). We chose materials that were known to be made of polypropylene and that were readily available, like sterilization wraps and HVAC filters (the latter being polymeric and not the type containing glass fibers), and polypropylene spunlace additive free baby wipes. As noted in , materials like unscented dry Swiffer wipes, 3 M FiltreteTM HVAC filters are accessible to the general public, but have unproven biocompatibility.
The most promising hospital-available material we tested was polypropylene sterilization wraps, which are designed to have both air permeability and microbial barrier functionality (Kiang Citation2018). As such, sterilization wraps are presumed to be both functionally similar, brand-to-brand, and are available and stocked in most hospitals for wrapping surgical instruments during sterilization and protecting instrument sterility following removal from the autoclave (“Choosing a Sterilization Wrap for SurgicalPacks” Citationn.d.). Sterilization wraps today are generally made of spunbond-meltblown-spunbond laminates (SMS) and have a fine layer of spunbond polymer that acts as a fine filter (Brock et al. Citation2006). The sterilization wraps tested in the current study were Cardinal Health CH300, because large quantities of these wraps were readily available at Intuitive Foundation. As all sterilization wraps have to keep medical instruments sterile after packaging, must meet certain regulatory standards (Kiang Citation2018; “FDA Recognized Consensus Standards for Containment devices for reusable medical device sterilization” Citationn.d.; “CFR - Code of Federal Regulations Title 21, 880.6850 - Sterilization wrap” Citationn.d.) and are generally made of similar non-woven SMS material, similar levels of performance would be expected from other brands of similar weight. The SW300 wraps come in double-layer configuration, but the two separate layers were functionally identical and differed only in color.
Another promising solution is several layers of additive free dry baby wipes (spunlace polypropylene filter). These had very high filtration performance and relatively small pressure drop. Because this material is already safe for skin contact and marketed as free of fragrances and additives, it would generally appear safe to use between two cotton layers in a mask. The spunlace process takes a fine web of loose fibers and bonds them with a high-powered water jet through the resulting friction (Evans Citation1969). In a limited washing and drying study, the dry baby wipes FE degraded and should be treated as disposable.
Unscented dry floor wipes (Swiffers) are primarily polyethylene, as determined by FTIR (fourier-transform infrared spectroscopy), and develop an electrostatic charge upon rubbing on a dissimilar material like cellulose (e.g., the triboelectric effect) (Dyer et al. Citation2011; “Electrostatic Forces: The Secret to Swiffers’ Swiftness | Trent’s Physics” Citationn.d.). The filtration efficiency of the dry floor wipes increased immediately and significantly after rubbing on a wood/cellulosic surface for about a minute, but all tests shown in the text are for wipes that have not been charged for at least 4 h. If there were a face mask design that could continually triboelectrically charge the Swiffer due to rubbing of a cotton liner with the Swiffer, the efficiency would further increase. The polymeric electrostatic HVAC filters performed very well, are made primarily of polypropylene (FTIR) and are thought to have an embedded electret from electrospraying the polymer or corona discharge treatments (Tsai, Schreuder-Gibson, and Gibson Citation2002; Tsai and Wadsworth Citation1995).
Electrostatic HVAC filters had a large degradation with washing and drying, especially at the smallest particle size. This is hypothesized to be due to the neutralization of electrostatic charge on the fiber network (Seugnet Citation1978). Dry baby wipes degraded as well, but due to changes in the network morphology. Dry floor wipes seemed to maintain performance for 5 wash and dry cycles, but suffered degradation, even at larger bins with subsequent washing. This was attributed to pilling and deformation of the non-woven cloth which created gaps that allowed particles to pass. Because the reusability study for the dry baby wipes, dry floor wipes and HVAC filters was conducted in just one household’s washer and dryer without other household laundry, unlike the cotton mask and sterilization wrap reusability study that was conducted in four households with regular laundry, we cannot as accurately anticipate the degradation behavior of these materials, and consider them disposable at this time.
Although unscented dry floor wipes and polymeric HVAC filters appear to be primarily PET (polyethylene) and PP (polypropylene), respectively, the possibility of trace materials or that the fine fiber forms of these materials that could be irritating or hazardous prevents us from making a recommendation for these materials at this time. If the material is proven biocompatible and used as a filter in a face mask, the material should be held within a liner to avoid direct skin contact. Moreover, because some HVAC filters are made of glass fibers, the use of HVAC filters for face mask applications are not recommended.
Although certified N95 respirators and ASTM F2100 procedure masks are preferred to improvised masks due to their standardized high filtration efficiency, improvised masks can provide relatively good filtration performance and can significantly reduce aerosols in the >2 µm range. The cottons tested had a wide range of filtrations, and the filtration efficiency was not readily predictable based on the specifications provided from retailers and suppliers. If a filter is going to be used with a cotton mask, low flow resistance cotton layers that keep the pressure drop close to or below procedure masks is recommended, as the filtration efficiency is dominated by the filter.
Conclusions
In this work, the filtration efficiency, breathability and reusability of commonly available fabrics were studied. The key observations from our testing include: two-layers of cotton fabric can provide a comfortable, breathable and reusable option that blocks many expiratory droplets and possibly aerosols from wearers, potentially protecting the public and reducing the spread of the virus. The addition of a filter layer such as a sterilization wrap can significantly improve filtration and block expiratory aerosols at the expense of an added pressure drop. Pairing the filter layer with low flow resistance cottons would be most beneficial to maintaining a low pressure drop and a high FE. Of the improvised filter materials tested, sterilization wraps present better filtration performance compared to cotton fabrics alone and are biocompatible. Cotton fabrics and sterilization wraps can be washed and dried at least 10 times without impacting FE or breathability. Several layers of dry additive free baby wipes (spunlace polypropylene) performed well, and should have a good safety profile, as they are designed for contacting skin, but are not as reusable. While very effective, polymeric HVAC filters and dry floor wipes cannot be recommended at this time due to an incomplete picture of their safety profile.
Author contributions
The manuscript was written through contributions of all authors. All authors have given approval to the final version of the manuscript.
Supplemental Material
Download PDF (1.8 MB)Acknowledgments
We gratefully acknowledge Mimi Wainwright for writing and editorial support, Dr. Anju Sharma of Binghamton University for her FTIR material analyses, Shawn Wagoner of the Binghamton University’s Cleanroom for lending us particle analyzers, Professor Bahgat Sammakia and his team for their helpful equipment loans, Dr. Zach Kurtz for statistical advice, and the Vestal Volunteer Fire Department for lending us a quantitative mask tester, and the Intuitive Foundation. We acknowledge financial support from Intuitive Foundation, Intuitive Surgical and Binghamton University’s TJ Watson School of Engineering’s COVID-19 Response Fund.
Additional information
Funding
References
- Asadi, S., A. S. Wexler, C. D. Cappa, S. Barreda, N. M. Bouvier, and W. D. Ristenpart. 2019. Aerosol emission and superemission during human speech increase with voice loudness. Sci. Rep. 9 (1):1–10. doi:https://doi.org/10.1038/s41598-019-38808-z.
- ASTM Standards & COVID-19. n.d. Accessed March 28, 2020. http://www.astm.org/COVID-19/.
- Balazy, A., M. Toivola, T. Reponen, A. Podgórski, A. Zimmer, and S. A. Grinshpun. 2006. Manikin-based performance evaluation of N95 filtering-facepiece respirators challenged with nanoparticles. Ann. Occup. Hyg. 50 (3):259–69. doi:https://doi.org/10.1093/annhyg/mei058.
- Brock, T., M. Cupta, B. Forbes, J. Lassig, and V. Smith. 2006. Spunbond-meltblown-spunbond laminates made from biconstituent meltblown materials. US20060141886A1.
- CDC. 2020a. How to wash cloth face coverings. Centers for Disease Control and Prevention. Accessed June 15, 2020. http://www.cdc.gov/coronavirus/2019-ncov/prevent-getting-sick/how-to-wash-cloth-face-coverings.html.
- CDC. 2020b. Use of cloth face coverings to help slow the spread of COVID-19. Centers for Disease Control and Prevention. Accessed May 16, 2020. http://www.cdc.gov/coronavirus/2019-ncov/prevent-getting-sick/diy-cloth-face-coverings.html
- CDC – 42 CFR Part 84 Respiratory Protective Devices. 2020. Accessed May 16, 2020. http://www.cdc.gov/niosh/npptl/topics/respirators/pt84abs2.html.
- Center for Devices and Radiological Health. 2020. Enforcement policy for face masks and respirators during the coronavirus disease (COVID-19) public health emergency (Revised). FDA-2020-D-1138. FDA. https://www.fda.gov/media/136449/download.
- CFR - Code of Federal Regulations Title 21, 880.6850 - Sterilization wrap. n.d. Accessed May 17, 2020. http://www.accessdata.fda.gov/scripts/cdrh/cfdocs/cfcfr/cfrsearch.cfm?fr=880.6850.
- Choosing a Sterilization Wrap for SurgicalPacks. n.d. Infection Control Today. Accessed May 14, 2020. http://www.infectioncontroltoday.com/environmental-hygiene/choosing-sterilization-wrap-surgicalpacks.
- Contreras, P. A., I. R. Sami, M. E. R. Darnell, M. G. Ottolini, and G. A. Prince. 1999. Inactivation of respiratory syncytial virus by generic hand dishwashing detergents and antibacterial hand soaps. Infect. Control Hosp. Epidemiol. 20 (1):57–8. doi:https://doi.org/10.1086/501550.
- Davies, A., K.-A. Thompson, K. Giri, G. Kafatos, J. Walker, and A. Bennett. 2013. Testing the efficacy of homemade masks: Would they protect in an influenza pandemic? Disaster Med Public Health Prep 7 (4):413–8. doi:https://doi.org/10.1017/dmp.2013.43.
- Drewnick, F., J. Pikmann, F. Fachinger, L. Moormann, F. Sprang, and S. Borrmann. 2021. Aerosol filtration efficiency of household materials for homemade face masks: Influence of material properties, particle size, particle electrical charge, face velocity, and leaks. Aerosol Sci. Technol. 55 (1):63–79. doi:https://doi.org/10.1080/02786826.2020.1817846.
- Duguid, J. P. 1946. The size and the duration of air-carriage of respiratory droplets and droplet-nuclei. J. Hyg. (Lond.) 44 (6):471–9. doi:https://doi.org/10.1017/s0022172400019288.
- Dyer, T. J., M. R. Lostocco, D. J. Nickel, T. M. Runge, K. J. Zwick, M. T. Goulet, J. J. Timm, P. H. Clough, and M. J. Rekoske. 2011. Wiping products having enhanced cleaning abilities. US7879191B2.
- Eikenberry, S. E., M. Mancuso, E. Iboi, T. Phan, K. Eikenberry, Y. Kuang, E. Kostelich, and A. B. Gumel. 2020. To mask or not to mask: Modeling the potential for face mask use by the general public to curtail the COVID-19 pandemic. Infect. Dis. Model. 5:293–308. doi:https://doi.org/10.1016/j.idm.2020.04.001.
- Electrostatic Forces: The Secret to Swiffers’ Swiftness | Trent’s Physics. n.d. Accessed May 14, 2020. http://trdye.wordpress.com/2013/01/21/electrostatic-forces-and-the-secret-to-swiffers-swiftness/.
- Evans, F. J. 1969. Textile-like patterned nonwoven fabrics and their production. US3485706A.
- F23 Committee. n.d. Specification for performance of materials used in medical face masks (No. ASTM F2100). West Conshohocken, PA: ASTM International.
- FDA recognized consensus standards for containment devices for reusable medical device sterilization. n.d. https://www.accessdata.fda.gov/scripts/cdrh/cfdocs/cfStandards/detail.cfm?standard__identification_no=38435
- Feng, S., C. Shen, N. Xia, W. Song, M. Fan, and B. J. Cowling. 2020. Rational use of face masks in the COVID-19 pandemic. Lancet. Respir. Med. 8 (5):434–6. doi:https://doi.org/10.1016/S2213-2600(20)30134-X.
- Furukawa, N. W., J. T. Brooks, and J. Sobel. 2020. Early Release - Evidence Supporting Transmission of Severe Acute Respiratory Syndrome Coronavirus 2 While Presymptomatic or Asymptomatic. Emerg. Infect. Dis. 26 (7):e1–e6. doi:https://doi.org/10.3201/eid2607.201595.
- Grayson, M. L., S. Melvani, J. Druce, I. G. Barr, S. A. Ballard, P. D. R. Johnson, T. Mastorakos, and C. Birch. 2009. Efficacy of soap and water and alcohol-based hand-rub preparations against live H1N1 influenza virus on the hands of human volunteers. Clin. Infect. Dis. 48 (3):285–91. doi:https://doi.org/10.1086/595845.
- Hamner, L., P. Dubbel, I. Capron, A. Ross, A. Jordan, J. Lee, J. Lynn, A. Ball, S. Narwal, S. Russell, et al. 2020. High SARS-CoV-2 attack rate following exposure at a choir practice — Skagit County, Washington. MMWR. Morb. Mortal. Wkly. Rep. 69 (19):606–10. doi:https://doi.org/10.15585/mmwr.mm6919e6.
- Hinds, W. C. 1999. Aerosol technology: Properties, behavior, and measurement of airborne particles. 2nd ed. Wiley.com. Accessed January 15, 2021. http://www.wiley.com/en-us/Aerosol+Technology%3A+Properties%2C+Behavior%2C+and+Measurement+of+Airborne+Particles%2C+2nd+Edition-p-9780471194101.
- Howard, J., A. Huang, Z. Li, Z. Tufekci, V. Zdimal, H.-M. van der Westhuizen, A. von Delft, A. Price, L. Fridman, L.-H. Tang, et al. 2020. Face masks against COVID-19: An evidence review. Med. Pharmacol. 118 (4):1–12. doi:https://doi.org/10.1073/pnas.2014564118.
- Huang, S.-H., C.-W. Chen, Y.-M. Kuo, C.-Y. Lai, R. McKay, and C.-C. Chen. 2013. Factors affecting filter penetration and quality factor of particulate respirators. Aerosol Air Qual. Res. 13 (1):162–71. doi:https://doi.org/10.4209/aaqr.2012.07.0179.
- Kiang, T. 2018. Section 510(k) premarket notification: K181174.
- Konda, A., A. Prakash, G. A. Moss, M. Schmoldt, G. D. Grant, and S. Guha. 2020. Aerosol filtration efficiency of common fabrics used in respiratory cloth masks. ACS Nano. 14 (8):10742–3. doi:https://doi.org/10.1021/acsnano.0c03252.
- Lau, J. T. F., H. Tsui, M. Lau, and X. Yang. 2004. SARS transmission, risk factors, and prevention in Hong Kong. Emerg. Infect. Dis. 10 (4):587–92. doi:https://doi.org/10.3201/eid1004.030628.
- Lauer, S. A., K. H. Grantz, Q. Bi, F. K. Jones, Q. Zheng, H. R. Meredith, A. S. Azman, N. G. Reich, and J. Lessler. 2020. The incubation period of coronavirus disease 2019 (COVID-19) from publicly reported confirmed cases: Estimation and application. Ann. Intern. Med. 172 (9):577–82. doi:https://doi.org/10.7326/M20-0504.
- Leung, N. H. L., D. K. W. Chu, E. Y. C. Shiu, K.-H. Chan, J. J. McDevitt, B. J. P. Hau, H.-L. Yen, Y. Li, D. K. M. Ip, J. S. M. Peiris, et al. 2020. Respiratory virus shedding in exhaled breath and efficacy of face masks. Nat. Med. 26 (5):676–80. doi:https://doi.org/10.1038/s41591-020-0843-2.
- Li, R., S. Pei, B. Chen, Y. Song, T. Zhang, W. Yang, and J. Shaman. 2020. Substantial undocumented infection facilitates the rapid dissemination of novel coronavirus (SARS-CoV-2). Science 368 (6490):489–93. doi:https://doi.org/10.1126/science.abb3221.
- Liu, P., Y. Yuen, H.-M. Hsiao, L.-A. Jaykus, and C. Moe. 2010. Effectiveness of liquid soap and hand sanitizer against Norwalk virus on contaminated hands. Appl. Environ. Microbiol. 76 (2):394–9. doi:https://doi.org/10.1128/AEM.01729-09.
- Modes of transmission of virus causing COVID-19: Implications for IPC precaution recommendations. n.d. Accessed May 10, 2020. http://www.who.int/news-room/commentaries/detail/modes-of-transmission-of-virus-causing-covid-19-implications-for-ipc-precaution-recommendations.
- Morawska, L., G. R. Johnson, Z. D. Ristovski, M. Hargreaves, K. Mengersen, S. Corbett, C. Y. H. Chao, Y. Li, and D. Katoshevski. 2009. Size distribution and sites of origin of droplets expelled from the human respiratory tract during expiratory activities. J. Aerosol Sci. 40 (3):256–69. doi:https://doi.org/10.1016/j.jaerosci.2008.11.002.
- O'Shaughnessy, P. T., and L. H. Schmoll. 2013. Particle count statistics applied to the penetration of a filter challenged with nanoparticles. Aerosol Sci. Technol. 47 (6):616–25. doi:https://doi.org/10.1080/02786826.2013.778954.
- Pei, C., Q. Ou, S. C. Kim, S.-C. Chen, and D. Y. H. Pui. 2020. Alternative face masks made of common materials for general public: Fractional filtration efficiency and breathability perspective. Aerosol Air Qual. Res. 20 (12):2581–91. doi:https://doi.org/10.4209/aaqr.2020.07.0423.
- Rengasamy, S., B. Eimer, and R. E. Shaffer. 2010. Simple respiratory protection-evaluation of the filtration performance of cloth masks and common fabric materials against 20-1000 nm size particles. Ann. Occup. Hyg. 54 (7):789–98. doi:https://doi.org/10.1093/annhyg/meq044.
- Rogak, S. N., T. A. Sipkens, M. Guan, H. Nikookar, D. V. Figueroa, and J. Wang. 2020. Properties of materials considered for improvised masks. Aerosol Sci. Technol. 55 (4):398–413. doi:https://doi.org/10.1080/02786826.2020.1855321.
- Seugnet, M. 1978. Fabric softener and anti-static compositions. US4118327A.
- Tang, J. W., A. D. Nicolle, C. A. Klettner, J. Pantelic, L. Wang, A. B. Suhaimi, A. Y. L. Tan, G. W. X. Ong, R. Su, C. Sekhar, et al. 2013. Airflow dynamics of human jets: Sneezing and breathing - Potential sources of infectious aerosols. PLoS One. 8 (4):e59970. doi:https://doi.org/10.1371/journal.pone.0059970.
- Tsai, P. P., H. Schreuder-Gibson, and P. Gibson. 2002. Different electrostatic methods for making electret filters. J. Electrost. 54 (3–4):333–41. doi:https://doi.org/10.1016/S0304-3886(01)00160-7.
- Tsai, P. P., and L. C. Wadsworth. 1995. Method and apparatus for the electrostatic charging of a web or film. US5401446A.
- van Doremalen, N., T. Bushmaker, D. H. Morris, M. G. Holbrook, A. Gamble, B. N. Williamson, A. Tamin, J. L. Harcourt, N. J. Thornburg, S. I. Gerber, et al. 2020. Aerosol and surface stability of SARS-CoV-2 as compared with SARS-CoV-1. N. Engl. J. Med. 382 (16):1564–7. doi:https://doi.org/10.1056/NEJMc2004973.
- Wang, J., and G. Du. 2020. COVID-19 may transmit through aerosol. Ir. J. Med. Sci. 189 (4):1143–1144. doi:https://doi.org/10.1007/s11845-020-02218-2.
- When and how to use masks. n.d. Accessed June 15, 2020. http://www.who.int/emergencies/diseases/novel-coronavirus-2019/advice-for-public/when-and-how-to-use-masks.