Abstract
Reference Material (RM) 8785 Air Particulate Matter on Filter Media is intended primarily for use in the evaluation of analytical methods used to characterize the carbon composition of atmospheric fine particulate matter (PM) for national air quality monitoring programs. This RM consists of a fine fraction (nominally < 2.5 μ m aerodynamic diameter) of standard reference material (SRM) 1649a Urban Dust resuspended in air and filtered onto quartz-fiber filters. RM 8785 will also provide the atmospheric-chemistry and ocean-sciences communities with a means to intercompare methods and laboratories for the measurement of elemental (black) carbon. RM 8785 has values assigned for total carbon, elemental carbon, and organic carbon mass fractions measured according to two thermal–optical methods: the Interagency Monitoring of Protected Visual Environments (IMPROVE) and Speciation Trends Network–National Institute of Occupational Safety and Health (STN–NIOSH) protocols. Each filter is uniquely identified by its air particulate matter (APM) number and its gravimetrically determined mass of fine SRM 1649a. We will discuss the method used to produce RM 8785 and the results of an intermethod and interlaboratory comparison that provided assigned values for the above analytes.
INTRODUCTION
For years researchers in the atmospheric sciences have recognized that filter-based particulate matter (PM) reference materials that represent different source mixtures were needed to calibrate and intercompare instruments that measure total carbon (TC), elemental carbon (EC), and organic carbon (OC) of PM2.5 at air-monitoring sites throughout the U.S. Around 1995, the National Institute of Standards and Technology (NIST) and SRI International began a collaboration to evaluate the SRI dust generation and collection system for resuspending milligram quantities of particulate matter on quartz-fiber and Teflon® filters (CitationKlouda et al. 1996). Previously, SRI routinely produced filters containing micrograms of silica (CitationAnderson 1983) or asbestos fibers (CitationTurner et al. 1992) for proficiency testing.
Initially, a “prototype” PM reference material was produced with SRI's system using standard reference material (SRM) 1649a Urban Dust (CitationBagley et al. 1992; CitationCurrie et al. 2002; CitationNIOSH 1999; CitationPeterson and Richards 2002; CitationPineiro-Iglesias et al. 2003; CitationWaterman et al. 2000; CitationWise et al. 1996, 1999). With a target loading of 3.5 mg per filter, the variability of dust across filters was as much as 20%. Visual observations of the loaded filters suggested that the air-monitoring cassettes used did not always provide an adequate seal and that occasionally filters contained large agglomerates of dust that had failed to be dispersed by the SRI system. As a result, specially designed filter packs by URG Corp. (Chapel Hill, NC, USA) were purchased to size-segregate particles by impaction, thereby allowing nominally < 2.5 μ m particles to reach the filters. Test runs with the new filter packs using SRM 1649a and a coalmine dust indicated that the PM is uniformly distributed within filters at the 10% level. However, the across-filter variability in the loading remained high at 15–20%. Therefore, it was determined that the particle-mass loading must be determined for each filter.
To expedite the production of a reference material of air particulate matter on filter media (RM 8785), SRM 1649a was selected as the feedstock even though it was collected in Washington, DC during 1976–1977. Today SRM 1649a has remained a valuable material for intercomparison of methods and laboratories. The advantage of using SRM 1649a was its immediate availability, proven stability over time, and known carbon composition. Through interlaboratory and intermethod comparisons SRM 1649a was certified for total carbon, and values were assigned for select trace-carbon species and carbon isotopes (NIST Certificate of Analysis, SRM 1649a). SRM 1649 has been shown to remain stable for polycyclic aromatic hydrocarbons (PAHs) from the time of its first issue (1982) to its recertification as SRM 1649a (CitationWise et al. 1996, 1999). Since 1999, there has been no indication that the material is changing over time based on measurements of PAHs (M. Schantz, personal communication 2004).
RM 8785, a fine fraction of SRM 1649a on a quartz-fiber filter, was expected to have a similar chemical composition as its raw material. In an earlier experiment of a trial resuspension deposited on PTFE (Teflo®) filters, X-ray fluorescence analysis showed that for several elements the resuspended material (on filter) was comparable to the bulk (original) SRM 1649a (). The following discussion will provide details of the production of RM 8785 and the results of an intermethod and interlaboratory comparison for assignment of carbon reference and information values.
EXPERIMENTAL
SRI Dust Generation and Collection System
Test atmospheres of particulate were dynamically generated in a dust generation and collection system engineered and built by SRI (CitationBaron and Deye 1987). An operational flow diagram of the generation and collection system is shown in . The method involves filtering the constituents of interest from air. The critical parameters are the homogeneity of the constituent in the mobile phase and the volume of air being filtered. Given a particular concentration in the mobile phase, the loading on the filter can be varied by changing the air sampling time. SRI's dust generation and collection system incorporates the following components to generate an aerosol:
• | Dust feeder–delivers a continuous stream of aerosol material | ||||
• | Sonic velocity disperser–breaks up agglomerates and dilutes the aerosol | ||||
• | Settling tower–removes large particles that survive the sonic airflow | ||||
• | Sampling collection chambers–houses 320 filter packs to simultaneously collect the aerosol on 320 filters under matched flow conditions |
FIG. 2 SRI dust generation and collection system: (a) operational flow diagram, (b) dust feeder, and (c) sample collection chamber.
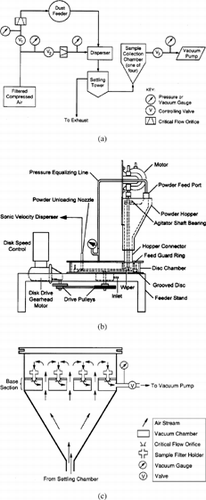
An in-line filter is installed on the compressed air supply with a rated efficiency of 99.99% for 0.6 μ m particles. The filter train consists of a Hankison refrigerated compressed air dryer, a grade-3, high-efficiency, oil removal filter, and grade-1, oil-vapor-removal filter. With the large volumes of dry air flowing throughout the system, static charge effects are minimized using Curastat in-line model SE I380® ionizers installed at several points in the system, including the dust feeder and dilution air lines. The absence of static charge is verified using an electrostatic field meter.
An SRI-constructed dust feeder () is used to introduce particulates into the collection system. The feeder can be used to generate particulate atmospheres of virtually any free-flowing powder. Particulate matter is metered by a grooved disk that rotates at a known rate.
The following is a brief discussion of the system's operational characteristics. Powder is loaded into the top of the powder hopper through the powder feed port. The powder then drops down into the hopper connector, where it is pushed into the groove of the disk by rubber wipers attached to the bottom of the agitator shaft. A spring-loaded guard ring surrounds the hopper connector and scrapes the disk to prevent the disk from carrying away excess powder. The rotation of the disk continuously carries the powder in the groove to the unloading nozzle, where it is removed pneumatically by compressed air. The powder feed rate is determined entirely by the rotation speed of the disk and the size of the groove. The loading of powder on sample filters is further adjusted by varying the collection time.
The air stream from the dust feeder carries the aerosol to the sonic velocity disperser at a flow rate of 60 l min−1. Dilution air is also delivered to the sonic velocity disperser, where it deagglomerates the aerosol under the action of an on-line static eliminator and high air velocity at a flow rate of 580 l min−1. The aerosol then enters the settling tower, whereby the linear velocity is reduced and the larger particles settle out to the base of the tower. The diluted aerosol is then divided uniformly among four equivalent collection chambers.
Figure 2c is a schematic of a collection chamber and its airflow characteristics. Each collection chamber consists of eighty sampling ports situated in an 8 × 10 matrix arrangement. Downstream from each port is a critical flow orifice. The mounting sheet in which the 80 critical flow orifices are embedded forms the upper section of a vacuum chamber, so that a vacuum to this chamber creates the necessary pressure differential to operate the orifices. Aerosol enters the collection chambers through 20 symmetrically located passages. The 320 orifices (80 for each of four sample collection chambers) all have the same diameter and were calibrated at the time the system was constructed to ensure that all the ports sample at the same flow rate. The orifices form a matched set, with a maximum flow rate of 2 l min−1 through each filter pack in the system. The collection chambers can be opened from the top by removing a cover. Filter packs are connected to the sampling port by a Luer® fitting.
RM 8785 Production
RM 8785 Air Particulate Matter on Filter Media was produced at SRI International by resuspending SRM 1649a in air and by collecting the aerosol on 320 quartz-fiber filters (tissue quartz 2500 QAT-UP; Pall Life Sciences®, Ann Arbor, MI, USA) in each of seven batches for a total of 2240 filters. To remove adsorbed organics, the manufacturer baked the filters at 500°C. Each quartz-fiber filter was housed in a URG-2000® filter pack (), specially designed to pass only particles nominally less than 2.5 μ m aerodynamic diameter and to provide a reliable seal around the filter by sandwiching the filter between two Teflon® gaskets (filter holder) to minimize potential loss of quartz fiber from the filter. Each impactor plate, positioned just beyond the filter pack inlet to remove particles greater than 2.5 μm aerodynamic diameter, was coated with 50 μl of a silicone oil solution prepared from Dow Corning 704® and toluene at a concentration of 10 mg ml−1 to minimize particle bounce. Quality control measures included checking each orifice flow rate with a digital flow meter before sample generation and analyzing for background levels to prevent carryover contamination.
Process blanks were prepared for possible chemical analysis. Prior to the first resuspension (batch), a background run was conducted for a period of 43 min to generate sample blanks representative of the process without dust present. The system was thoroughly cleaned before this run to remove all traces of particulate from earlier runs. The run consisted of 320 unweighed quartz-fiber filters referred to as process blanks. A previous background run of the SRI system over a 1 h duration with quartz-fiber filters showed that the average and the standard deviation of the blank were 3.3 μ g cm− 2 and 0.3 μ g cm− 2 (n = 4), respectively, with no EC detected.
Between each batch run, filter packs were disassembled and cleaned to prevent buildup of impacted dust from contaminating filters during subsequent runs. The cleaning process included sonicating the impaction plate, spiking the plate with fresh collection oil, and wiping of excess dust from the filter pack surfaces.
RM 8785 Gravimetric Measurements
The mass of urban dust on each filter was determined gravimetrically by weighing each filter before and after the resuspension and collection process. Prior to gravimetric analysis, quartz-fiber filters were conditioned in an average temperature of 21.5°C within 1.5°C and a relative humidity of 35% within 5% (absolute) controlled clean room for a minimum of 24 h. Each filter was visually examined for pinholes and creases as part of filter acceptance testing. Gravimetric analyses were conducted on a Mettler Toledo MT5® microbalance with a sensitivity of 1 μg. The filters were hand carried from Desert Research Institute's (DRI) facility in Reno, NV to SRI International prior to and after resuspension sampling to minimize particle losses during transit.
Quartz-fiber filters are friable, and although they were carefully placed in and removed from the filter holder, some material might have been lost from some filters. Filters also adsorb organic vapors that can increase their mass. Each filter was inventoried according to its filter identification number, mass loading, batch, chamber, and position within the chamber.
The distribution of PM mass loadings with respect to chamber position, chamber, and batch appeared quite variable. After nine outlier filters with negative loadings and (or) apparent damage were removed, 2231 filters remained. The average mass, the standard deviation, and the standard uncertainty of the mean (CitationTaylor and Kuyatt 1994; CitationISO 1993a) were 1.064 mg, 0.414 mg, and 0.009 mg, respectively. This corresponds to a relative standard deviation of 38.9% and a relative standard uncertainty of the mean of 0.9%. We note that the median of the 2231 filters was 1.022 mg, with a standard uncertainty (via bootstrapping) of the median of 0.009 mg (0.9%). Also, note that there is little difference between the mean (1.064 mg) and the median (1.022 mg); for simplicity we have chosen the mean for the final reported value. See for a histogram and summary statistics.
Filter controls were weighed to estimate potential bias in the gravimetric measurement. Gravimetric analysis of so-called field blanks, control filters that were placed into and taken out of filter holders without exposure to the dust, showed that the average and the standard deviation of 34 differences between the final weighing and the initial weighing was 39 μg and 63 μg, respectively. Blank filters used as gravimetric controls only during the weighing process showed that (based on 106 observations) the average and the standard deviation of the change in weights were 49 μg and 63 μ g, respectively. Blank filters, designated as replacements, if any filters were compromised before sampling, showed that (for 20 observations) the average and the standard deviation of the change in weights was 80 μg and 54 μg, respectively. Although relative standard deviations are large (> 100%) for each of the filter blank types, central tendencies of these blanks suggest a pattern. Field blanks, those handled more than gravimetric controls or replacement filters, have less of a difference between the initial and final weighings than do nearly virgin filters. This suggests a weight difference loss due to quartz fibers lost during handling, which would negate to some degree a positive bias due to adsorbed organic carbon.
INTERMETHOD AND INTERLABORATORY COMPARISON
The objective of the intermethod comparison was to obtain value assignments (CitationMay et al. 2000; CitationISO 1993b) and uncertainties (CitationTaylor and Kuyatt 1994; CitationISO 1993a) for EC, OC, and TC content (in g g−1 dust) and for the EC/TC ratio of RM 8785 filters using two specific thermal optical analysis (TOA) methods: the Speciation Trends Network–National Institute of Occupational Safety and Health (STN-NIOSH) and the Interagency Monitoring of Protected Visual Environments (IMPROVE). The STN-NIOSH is the NIOSH Method 5040 (CitationNIOSH 1999; CitationBirch and Cary 1996) with a slightly modified temperature profile for the EPA PM2.5 Chemical STN, and it was applied to an original thermal–optical transmission (TOT) instrument and to the new version of the TOT instrument. Analyses were performed by NIST and Sunset Laboratory Inc. (SLI), respectively ().
TABLE 1 STN–NIOSH method (TOT laboratories)
The IMPROVE method (CitationChow et al. 1993) was applied to the original (DRI/OGC) thermal–optical reflectance (TOR) instrument and a new (commercial) version of the TOR instrument (DRI Model 2001®). Both of these analyses were performed by DRI (). The IMPROVE method was previously compared to the STN–NIOSH method by measuring PM2.5 in ambient and source samples using DRIs new thermal–optical instrument that incorporates both reflectance and transmission measurements (CitationChow et al. 2001). Also, a more recent study has focused on optimizing TOA instruments for measuring atmospheric EC by studying the response surface of a TOT instrument (CitationConny et al. 2003). A further refinement in the analysis was to compare the relative behavior of the laboratories (instruments); details of the design are given below.
TABLE 2 IMPROVE method (TOR laboratory)Footnote a
Experimental Design and Filter Selection
In order to intercompare methods and laboratories, the RM 8785 filter population was divided into eight mass intervals, considering that the relative standard uncertainty of mass loading was 39%. The mass intervals were defined by ordering all 2231 filters from the least-loaded filter to the heaviest loaded over the entire mass range, nominally 0.09–2.9 mg per filter, divided by eight (octile). The octiles are listed in with their summary statistics: number of filters (n), minimum, maximum, median, range, average, standard deviation of the data, and relative standard deviation. Each octile contains 279 filters, except for the eighth octile, which contains 278.
TABLE 3 RM 8785 mass interval (octile) summary
From each octile two filters were randomly selected for each laboratory. An additional filter was randomly selected from each of the eight octiles for round-robin distribution, and one punch per filter was sent to each of the four laboratories. (Originally, four laboratories were identified for the intercomparison, two TOR laboratories and two TOT laboratories. Later, one TOR laboratory was replaced by DRI using the new version TOR instrument. Laboratories were selected based on experience and methods used at NIST for value-assignment (CitationMay et al. 2000).) For round-robin filters only, TOT laboratories were sent square punches of 1.0 cm2 and TOR laboratories were sent circular punches of 0.50 cm2 (0.80 cm diameter). All filters and filter punches were sent blind with respect to their air particulate matter (APM) identification number and to their corresponding mass of fine SRM 1649a. The filter selection represented a balanced design with replication and round-robin sampling. For this design, 72 filters (i.e., 8 octiles × 2 replicates × 4 instruments + 8 round-robin filters) were consumed for value assignment. All filters, filter punches, and reference solutions (identified below) were shipped to laboratories with a cold pack in a styrofoam container by overnight airfreight. The variability in the data will therefore include any losses of particulate matter that may have resulted during shipping.
Reference Solutions
Each laboratory received two reference solutions and high performance liquid chromatography (HPLC) water (blank) contained in 4.5 ml Certan® capillary bottles: a urea solution of 0.203 μgC μl−1 and a sucrose solution of 4.078 μgC μl−1. Microliter water-type syringes were used to transfer solution volumes from the Certan® vial onto an unused prefired (900°C) quartz-fiber filter punch of an area specified by the method. These so-called control-filter punches were defined as follows:
• | C1 was 5 μl of 0.203 μ gC μl−1 urea; | ||||
• | C2 was 5 μl of 4.078 μ gC μl−1 sucrose; | ||||
• | C3 was 25 μl of 0.203 μ gC μl−1 urea; | ||||
• | C4 was 20 μl of 4.078 μ gC μl−1 sucrose; and | ||||
• | C5 was HPLC water blank used to prepare the solutions. |
For the IMPROVE method, C4 was actually defined as 10 μl of 4.078 μ gC μl−1 sucrose because 20 μl saturated the detector. Control filters were prepared the day of the analysis, and they provided a means to evaluate the calibration of each instrument daily over the range of carbon concentrations expected.
Instrument Calibration
Each laboratory provided the results of daily analysis of reference solutions as identified above. The measured carbon values were compared to the expected carbon values and fit to a linear curve. shows the combined results of all calibration controls analyzed throughout the intercomparison for each of the laboratories and methods. The standard uncertainty for the coefficients represents within and across day variability.
TABLE 4 Linear regression analysis of calibration controls (reference solutions)
Laboratories, Methods, and Thermal–Optical Analyses
A set of RM 8785 filters was analyzed by DRI for TC, EC, and OC using the DRI/OGC TOR instrument and applying the IMPROVE method (CitationChow et al. 2001). A second set of RM 8785 filters, originally targeted for analysis by a second laboratory also having a DRI/OGC TOR instrument, was also sent to DRI and analyzed using the DRI Model 2001® thermal/optical analyzer (Atmoslytic Inc., Calabasas, CA, USA), which differs somewhat from the DRI/OGC design (CitationChow et al. 2001). Both sets of measurements were performed at DRI and are designated as follows: DRI = DRI/OGC TOR and DRI2 = DRI Model 2001® TOR.
A third set of RM 8785 filters was analyzed by SLI for TC, EC, and OC using the new version TOT instrument and applying the STN–NIOSH method. A fourth set of RM 8785 filters was retained by NIST and analyzed using an original TOT instrument and also applying the STN–NIOSH method. TOT laboratories are designated as follows: NIST = original TOT and SLI = new version TOT.
Each laboratory was instructed to measure each filter in triplicate according to a strict measurement protocol summarized below, except for round-robin filters, of which only one punch per filter was analyzed by each laboratory method. If there appeared to be an outlier among the triplicate measurements, laboratories were instructed to analyze a fourth aliquant. The total number of carbon measurements across all laboratories' methods amounted to 232.
Measurement Protocol
For each day, each laboratory was instructed to analyze RM 8785 filter (F) and control-filter (C) punches according to a specific measurement protocol (sequence) outlined in that uses a block design for control-filter punches and randomization of RM 8785 filter punches. RM 8785 filter and control-filter punches were measured over the course of nine days. Seven of the days were assigned measurement of eight RM 8785 filter punches and six control-filter punches per day, C1–C4 plus C5 in duplicate. The pattern (CCFFFFC) was designed to split the daily workload into two half-day sessions if necessary, with four randomly ordered filters (F), one water blank (C5), one low control (C1), and one high control (C3) for the morning. In the afternoon, the block-of-four filters (F) were also randomized, and the controls C2, C4, and C5 were analyzed. This sequence of measuring controls was the same for each day the filters were analyzed. It was strongly suggested that all measurements in a single day be made without significant interruption. The maximum of 14 analyses per day was chosen to avoid exceeding a typical workday.
TABLE 5 RM 8785 filter (F) and control (C) filter measurement protocol (sequence of analyses)
The remaining two days, days one and nine, were devoted entirely to measuring control-filter punches C1–C5 in duplicate. Water blanks (C5) were the first and last measurements of the day. Like RM 8785 filters, the two half-day blocks of four measurements each consisted of randomized C1–C4. For each control-filter punch, the specified microliter (μl) volume was transferred to a prefired quartz-fiber filter punch, dried, and analyzed in the sequence as described.
RESULTS AND DISCUSSION
RM 8785 Thermal–Optical Results
Laboratories reported carbon concentrations in units of μ g cm− 2, not corrected for the filter blank. Within-laboratory variations of TC, EC, and OC in μ g cm− 2 were explored as a function of the mass of fine particulate SRM 1649a. The slope and intercept determined for each regression analysis is reported along with its expanded uncertainty (U), i.e., twice the standard deviation of the coefficient (). The residual (Res.) standard deviation (SD) is reported in columns 6, 11, and 16 for each regression analysis. Considering the expanded uncertainty of the slope of each regression, the carbon variability ranges from 8 to 16%. The results of all four instruments show residual standard deviations (Res. SD) ranging from 3.6 to 5.0 μ g cm− 2 for TC, 1.7 to 2.1 μ g cm−2 for EC, and 1.8 to 4.2 μ g cm−2 for OC.
TABLE 6 RM 8785 linear regression analysis results of each laboratory
A comparison of slopes for the TOR instruments shows that there is a statistically significant difference for the TC slopes at the 1% level, for the EC slopes at the 0.01% level, and for the OC slopes at the 5% level. On the other hand, the same comparison for the TOT instruments revealed no significant difference between the two TOT laboratories for TC, EC, or OC.
The discrepancy between DRI and DRI2 was an unexpected result from this interlaboratory experiment. Further investigation to resolve the cause of this difference is under consideration, the result of which will be reported on at a later time.
RM 8785 Carbon Mass Fraction and EC/TC Ratio
The carbon mass fraction (g g−1) and its standard uncertainty (u) were obtained for each laboratory method by multiplying the slope of each regression line and its u () by 8.55 cm2 (deposit area). Thus, the individual laboratory-method result of RM 8785 carbon mass fraction (i.e., gram of carbon per gram of fine-particulate SRM 1649a deposited on the filter) and expanded uncertainty (U) (CitationTaylor and Kuyatt 1994; CitationISO 1993a) are reported in , corrected for laboratory bias using the slopes from .
TABLE 7 RM 8785 carbon mass fractionFootnote a
The EC/TC ratio in was obtained by regressing EC (μ g cm− 2) on TC (μ g cm− 2), thereby the slope is representative of the EC/TC ratio independent of any uncertainty or bias in the gravimetric measurement. We note from that the EC/TC ratios for DRI and DRI2—0.465 ± 0.019 (U) and 0.514 ± 0.009 (U), respectively—are statistically different based on the t test. This is also evident in of the individual EC/TC ratios (circles) and the box-and-whisker representation of the distribution, i.e., median, first and third quartile ranges (box), and whiskers within 1.5 interquartile ranges above and below the box.
FIG. 5 RM 8785 EC/TC ratios for DRI, DRI2, SLI, and NIST: distribution (circles) and box-and-whisker representation.
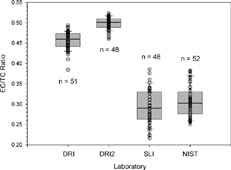
EC/TC ratios for SLI and NIST, 0.282 ± 0.029 (U) and 0.275 ± 0.034 (U), are statistically indistinguishable ().
To minimize the possibility that filter-to-filter effects contributed to the above apparent difference between the DRI instruments, the data from a parallel round-robin experiment was examined. In this experiment, four punches from the same filter were extracted and distributed to the three participating laboratories; DRI received two punches, one for each instrument. This was done for a total of eight filters, which were chosen so as to span the entire gravimetric range. The result of the experiment was that the calculated EC/TC values for DRI were universally (that is, for all eight filters) less than the EC/TC values for the DRI2 (). The probability of this happening by chance is less than 1%, thus making this event also statistically significant based on the paired t test. The SLI and NIST results of round-robin filters showed no pattern ().
FIG. 6 RM 8785 EC/TC ratio for eight round-robin filters: paired comparisons of DRI versus DRI2 (IMPROVE) and SLI versus NIST (STN-NIOSH). Plot character = filter (1 to 8).
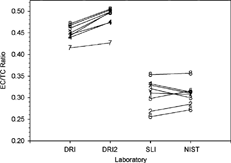
For comparison purposes, the EC/TC ratio was calculated using the mass fractions of EC (g g−1) and TC (g g−1) of columns six and four, respectively (). As one might expect, the regression approach to the EC/TC ratio yields significantly smaller relative standard uncertainties (u), ranging from 1 to 6%, than the calculated approach to the EC/TC ratio using the mass fractions, ranging from 6 to 10%; the latter relative SD was calculated based on propagated standard uncertainties (u). The degree to which the EC/TC values of these two approaches agree, i.e., within < 5% of each other, yields confidence in the gravimetric measurement.
RM 8785 Carbon Value Assignments
A value assignment was determined for each carbon mass fraction for each method. The carbon concentration (μ g cm− 2), adjusted for laboratory bias, was regressed against its corresponding mass of fine particulate SRM 1649a for all measurements obtained from each method. Value assignments and expanded uncertainties (U) for each method are reported as gram of carbon per gram of fine-particulate SRM 1649a deposited on the filter (). The TC reference value assignment for all four laboratory methods combined is 0.229 ± 0.050 g g−1 (U, n = 4); not corrected for blank total carbon.
TABLE 8 NIST RM 8785 value assignments and expanded uncertainty—bias corrected (see )Footnote a
The TC mass fraction of RM 8785 is assigned reference concentration valuesand uncertainties, since values were obtained through an intermethod and interlaboratory comparison involving two TOA methods and three laboratories, one of which was NIST. Reference values are noncertified values that are the best estimate of the true value. Reference values do not meet the NIST criteria for certification since the associated uncertainties may represent only measurement precision, may not include all sources of uncertainty, or may reflect a lack of sufficient statistical agreement among multiple analytical methods.
The EC and OC mass fractions are assigned information concentration values because they differ statistically across methods. Thereby, they are method-defined parameters (). An information value is considered to be a value that will be of use to the SRM user but should not be interpreted as a “true” value, especially in the case of TOA. The EC information concentration value for the IMPROVE method is 0.111 ± 0.035 g g−1 (U, n = 2); the uncertainty for the most part influenced by the significant difference between the two DRI instruments. The EC information concentration value for the STN–NIOSH method is 0.067 ± 0.008 g g−1 (U, n = 2). EC information concentration values are not corrected for blank EC. EC concentrations were only slightly influenced by the optical technique employed. Both transmission and reflectance measurements were obtained simultaneously from the DRI2 instrument (DRI Model 2001®). EC concentrations by reflectance averaged about 2% higher than by transmission for the same filters. The OC information concentration values for both methods are reported in .
CONCLUSIONS
The TC reference value assignment is 0.229 ± 0.050 g g−1 (U = 2u, 95% confidence level, n = 4 laboratory methods), which represents the combined measurements of the IMPROVE (n = 2) and STN–NIOSH (n = 2) methods. This value assignment is representative of three laboratories applying two methods with each laboratory method performed on 16 filters analyzed, 2 filters selected from each octile (mass interval) for each laboratory method analyzed at least in triplicate, plus a single analysis performed by each laboratory method on each of 8 round-robin filters, with one filter selected from each octile.
The EC and OC mass fractions are method dependent and have assigned concentration values that differ across methods for EC by 1.66 ± 0.23 (u, standard uncertainty), IMPROVE:STN–NIOSH ratio; thus it follows algebraically that OC differs across methods by 0.66 ± 0.09 (u) for this ratio. These differences between the two methods are in agreement with TOA measurements of a set of filters having nominally 0.8 mg of fine SRM 1649a deposited identically as RM 8785 but produced prior to the production of RM 8785 (CitationConny et al. 2003). Also, a comparison of the two methods was made using source and ambient PM showing similar results (CitationChow et al. 2001). Therefore, these method-defined parameters can be assigned information values.
RM 8785 Air Particulate Matter on Filter Media represents the first NIST RM developed for the atmospheric sciences monitoring community for intercomparing TC, EC, and OC measurements of atmospheric PM collected on quartz-fiber filter. Specifically, RM 8785 is targeted to thermal–optical instruments that measure EC.
Acknowledgments
The authors wish to thank the following individuals who were instrumental in assisting in the research and development of RM 8785: J. M. Conny, L. A. Currie, D. B. Klinedinst, K. K. Takagi, and M. E. Knab of NIST; E. L. Watson and K. Irwin of SRI; D. Crow, A. Chen, and S. Kohl of DRI; R. B. Kellogg, G. Norris, and J. Weinstein of USEPA; and J. Stone of URG Corp. Funding was in part provided by the US Environmental Protection Agency under Interagency Agreement DW 13939042-01-0 and the National Institute of Standards and Technology, Standards Reference Material Program. Certain commercial equipment, instruments, or materials are identified in this article to specify the experimental procedure adequately. Such identification does not imply recommendation or endorsement by the National Institute of Standards and Technology, nor does it imply that the materials and equipment identified are necessarily the best available for the purpose.
Notes
a UHP, ultrahigh-purity helium (99.999%).
b SLI = 2% O2 in UHP He; NIST = 1% O2 in UHP He.
a Each step proceeds until the FID returns to baseline.
b UHP, ultrahigh-purity helium (99.999%).
a SD of the data. Number in brackets is the relative SD in percent.
* During the interlaboratory study, samples of RM 8785 were identified as “SRM 2784.” SRM 2784 is the SRM number reserved for this material once certification of an analyte is obtained.
a The expanded uncertainty is U = 2u, where u is the standard deviation of the coefficient. The quantity U serves as a basis for computing symmetric 95% confidence limits for the true parameter values.
a IMPROVE = TOR; STN–NIOSH = TOT.
b μg cm− 2 μ g−1 of a fine fraction of SRM 1649a.
c The expanded uncertainty is U = 2u, where u is the standard deviation of the coefficient. The quantity U serves as a basis for computing 95% confidence limits for the true parameter value.
d μ g cm− 2; Res. SD, the residual standard (deviation) uncertainty of the fitted line.
a Based on a total deposit area of 8.55 cm2 and corrected for laboratory calibration bias using the slopes of . Not corrected for blank total carbon.
b Reference value, mass fraction.
c The expanded uncertainty is U = 2u, where u is the standard deviation of the coefficient. The quantity U serves as a basis for computing 95% confidence limits for the true parameter value.
d Information value, mass fraction.
a Carbon mass fractions, based on a total deposit area of 8.55 cm2. Not corrected for blank total carbon.
b Reference value, mass fraction.
c Information value, EC and OC are mass fractions; EC/TC ratio. The reported value of EC/TC in is the average of the corresponding two values in .
d The expanded uncertainty is U = 2u, where u is the standard deviation of the coefficient. The quantity U serves as a basis for computing 95% confidence limits for the true parameter value. The unweighted standard deviation (u) of the mean of these two values was computed, and U = 2u was reported in .
REFERENCES
- Anderson , C. C. 1983 . Collaborative Tests of Two Methods for Determining Free Silica in Airborne Dust , DHHS (NIOSH) Publication No. 83–124 SRI International Report to the U.S. Department of Health and Human Services
- Bagley , S. T. , Stolz , S. L. , Becker , D. M. and Keen , R. E. 1992 . Characterization of Organic Extracts from Standard Reference Materials 1649, Urban Dust Organics, and 1650, Diesel Particulate Matter, Using a Microsuspension Assay—A WHO IPCS CCSM Study . Mutat. Res. , 276 ( 1–2 ) : 81 – 86 . http://www.ncbi.nlm.nih.gov/entrez/query.fcgi?cmd=Retrieve&db=pubmed&dopt=Abstract&list_uids=1370110 http://www4.infotrieve.com/newmedline/detail.asp?NameID=1370110&Session=&searchQuery=Mutat%2E+Res%2E%5BJournal+Name%5D+AND+276%5BVolume%5D+AND+81%5BPage+Number%5D+AND+1992%5BPublication+Date%5D+AND+Bagley%5BAuthor+Name%5D&count=1
- Baron , P. A. and Deye , G. J. 1987 . Generation of Replicate Asbestos Aerosol Samples for Quality Assurance . Appl. Ind. Hyg. , 2 ( 3 ) : 114 – 118 .
- Birch , M. E. and Cary , R. A. 1996 . Elemental Carbon-Based Method for Monitoring Occupational Exposures to Particulate Diesel Exhaust . Aerosol Sci. Technol. , 25 : 221 – 241 .
- Chow , J. C. , Watson , J. G. , Pritchett , L. C. , Pierson , W. R. , Frazier , C. A. and Purcell , R. G. 1993 . The DRI Thermal/Optical Reflectance Carbon Analysis System: Description, Evaluation and Applications in U.S. Air Quality Studies . Atmos. Environ. , 27A ( 8 ) : 1185 – 1201 .
- Chow , J. C. , Watson , J. G. , Crow , D. , Lowenthal , D. H. and Merrifield , T. 2001 . Comparison of IMPROVE and NIOSH Carbon Measurements 2001 . Aerosol Sci. Technol , 34 : 23 – 34 . http://www.csa.com/htbin/linkabst.cgi?issn=0278-6826&vol=34&iss=&firstpage=23
- Conny , J. M. , Klinedinst , D. B. , Wight , S. A. and Paulsen , J. L. 2003 . Optimizing Thermal-Optical Methods for Measuring Atmospheric Elemental (black) Carbon: A Response Surface Study . Aerosol Sci. Technol , 37 : 703 – 723 . http://dx.doi.org/10.1080%2F02786820300920
- Currie , L. A. , Benner , B. A. Jr. , Cachier , H. , Cary , R. , Chow , J. C. , Druffel , E. R. M. , Eglington , T. I. , Gustafsson , O. , Hartmann , P. C. , Hedges , J. I. , Kessler , J. D. , Kirchstetter , T. W. , Klinedinst , D. B. , Klouda , G. A. , Marolf , J. V. , Masiello , C. A. , Novakov , T. , Pearson , A. , Prentice , K. M. , Puxbaum , H. , Quyinn , J. G. , Reddy , C. M. , Schmid , H. , Slater , J. F. , Watson , J. G. and Wise , S. A. 2002 . A Critical Evaluation of Interlaboratory Data on Total, Elemental, and Isotopic Carbon in the Carbonaceous Particle Reference Material, NIST SRM 1649a . J. Res. National Bureau Standard , 107 ( 3 ) : 279 – 298 .
- International Organization for Standardization (ISO) . 1993a . Guide to the Expression of Uncertainty of Measurement , First edition , Geneva , , Switzerland : International Organization for Standardization . ISBN 92-67-10188-9, reprinted 1995
- International Organization for Standardization (ISO). 1993b . International Vocabulary of Basic and General Terms in Metrology (VIM) , 2nd ed. , Geneva , , Switzerland : International Organization for Standardization . ISBN 92-67-01075-1
- Klouda , G. A. , Klinedinst , D. B. , Steel , E. B. , Benner , B. A. Jr. and Parish , H. J. 1996 . Exploring a Method to Produce an Urban Dust Particle Filter Standard . J. Aerosol Sci. , 27 ( Suppl. 1 ) : S351 – S352 . http://www.csa.com/htbin/linkabst.cgi?issn=0021-8502&vol=27&iss=Suppl.+1&firstpage=S351 http://dx.doi.org/10.1016%2F0021-8502%2896%2900248-0
- May , W. , Parris , R. , Fassett , J. , Greenberg , R. , Guenther , F. , Wise , S. , Gills , T. , Colbert , J. , Gettings , R. and MacDonald , B. 2000 . Definitions of Terms and Modes Used at NIST for Value-Assignment of Reference Materials for Chemical Measurements , Gaithersburg , MD : National Institute of Standards and Technology . National Institute of Standards and Technology (NIST) Special Publication 260–136.
- National Institute of Standards and Technology (NIST). 2001 . Certificate of Analysis, SRM 1649a Urban Dust , Gaithersburg , MD : National Institute of Standards and Technology .
- National Institute of Occupational Safety and Health (NIOSH) . 1999 . Elemental Carbon (Diesel Particulate): Method 5040,” Issue 3 (Interim), NIOSH Manual of Analytical Methods. , 4th ed. , DHHS (NIOSH) Publication No. 94–113 Edited by: Cassinelli , M. E. and O'Connor , P. F. Cincinnati , OH : National Institute for Occupational Safety and Health, U.S. Department of Health and Human Services .
- Peterson , M. R. and Richards , M. H. 2002 . “ Thermal-Optical Transmittance Analysis for Organic, Elemental, Carbonate, Total Carbon, and OCX2 in PM2.5 by the EPA/NIOSH method ” . In Proceedings, Symposium on Air Quality Measurement Methods and Technology—2002 , Edited by: Winegar , E. D. and Tropp , R. J. 83-1 – 83-19 . Pittsburgh , PA : Air & Waste Management Association .
- Pineiro-Iglesias , M. , Lopez-Mahia , P. , Muniategui-Lorenzo , S. , Prada-Rodriguez , D. , Querol , X. and Alastuey , A. 2003 . A New Method for the Simultaneous Determination of PAH and Metals in Samples of Atmospheric Particulate Matter . Atmos. Environ , 37 ( 29 ) : 4171 – 4175 . http://dx.doi.org/10.1016%2FS1352-2310%2803%2900523-5
- Taylor , B. N. and Kuyatt , C. E. 1994 . Guidelines for Evaluating and Expressing the Uncertainty of NIST Measurement Results , Gaithersburg , MD : National Institute of Standards and Technology . Natl. Inst. Stand. Technol. Tech. Note 1297.
- Turner , S. , Steel , E. B. , Phelps , J. M. , Windsor , E. S. , Doorn , S. S. and Leigh , S. D. 1992 . “ Development of a Standard Reference Material for Analysis of Asbestos on Filters by TEM ” . In Proceedings of the 50th Annual Meeting of the Electron Microscopy Society of America , Edited by: Bentley , G. W. , Bentley , J. and Small , J. A. 1706 – 1707 . San Francisco : San Francisco Press .
- Waterman , D. , Horsfield , B. , Leistner , F. , Hall , K. and Smith , S. 2000 . Quantification of Polycyclic Aromatic Hydrocarbons in the NIST Standard Reference Material (SRM 1649a) Urban Dust Using Thermal Desorption GC/MS . Anal. Chem. , 72 : 3563 – 3567 . http://www.ncbi.nlm.nih.gov/entrez/query.fcgi?cmd=Retrieve&db=pubmed&dopt=Abstract&list_uids=10952543 http://www4.infotrieve.com/newmedline/detail.asp?NameID=10952543&Session=&searchQuery=Anal%2E+Chem%2E%5BJournal+Name%5D+AND+72%5BVolume%5D+AND+3563%5BPage+Number%5D+AND+2000%5BPublication+Date%5D+AND+Waterman%5BAuthor+Name%5D&count=1 http://dx.doi.org/10.1021%2Fac991372x
- Wise , S. A. , Schantz , M. M. , Hays , M. J. , Koster , B. J. , Sharpless , K. E. , Sander , L. C. , Benner , B. A. Jr. and Schiller , S. B. 1996 . Certification of Polycyclic Aromatic Hydrocarbons in Mussel Tissue and Air Particulate Standard Reference Materials (SRMs) . Polycyclic Aromatic Compounds , 9 : 209 – 216 .
- Wise , S. A. , Sander , L. C. , Schantz , M. M. , Hays , M. J. and Benner , B. A. 1999 . Recertification of Standard Reference Material (SRM) 1649, Urban Dust, for the Determination of Polycyclic Aromatic Hydrocarbons (PAHs) . Polycyclic Aromatic Compounds , 13 ( 4 ) : 419 – 456 .