Abstract
Regional particle deposition efficiency and deposition patterns were studied experimentally in a human airway replica made from an adult cadaver. The replica includes the oral cavity, pharynx, larynx, trachea, and four generations of bronchi. This study reports deposition results in the tracheobronchial (TB) region. Nine different sizes of monodispersed, polystyrene latex fluorescent particles in the size range of 0.93–30 μm were delivered into the lung cast with the flow rates of 15, 30, and 60 l min− 1. Deposition in the TB region appeared to increase with the increasing flow rate and particle size. Comparison of deposition data obtained from physical casts showed agreement with results obtained from realistic airway replicas that included the larynx. Deposition data obtained from idealized airway models or replicas showed lower deposition efficiency. We also compared experimental data with theoretical models based on a simplified bend and bifurcation model. A deposition equation derived from these models was used in a lung dosimetry model for inhaled particles, and we demonstrated that there was general agreement with theoretical models. However, the agreement was not consistent over the large range of Stokes number. The deposition efficiency was found as a function of the Stokes number, bifurcation angle, and the diameters of parent and daughter tubes. An empirical model was developed for the particle deposition efficiency in the TB region based on the experimental data. This model, combined with the oral deposition model developed previously, can be used to predict the particle deposition for inertial effects with improved accuracy.
INTRODUCTION
The tracheobronchial (TB) airways consist of repeated bifurcating tubes (CitationWeibel 1963). Aerosol deposition in the TB region is a concern in the development of lung diseases, including asthma and bronchogenic cancer. Accurate dosimetric determination is important in assessing realistic risks of inhalation hazards from ambient and occupational exposure. Four major deposition mechanisms include diffusion, gravitational settling, inertial impaction, and interception. Diffusion and gravitational settling are important in small airways, whereas inertial impaction is dominant in the upper TB region. Interception is essential in preventing deposition of fibrous aerosol.
Both experimental and theoretical studies have been used to study regional airway deposition. Made from glass or metal, physical casts of a TB tree from one to several symmetrical bifurcations and having the dimensions of Weibel's idealized airway geometry (CitationWeibel 1963) were used in experimental observations of flow patterns (CitationSchroter and Sudow 1969), as well as aerosol deposition patterns (CitationFerron 1977; CitationMyojo 1987, Citation1990; CitationKim and Iglesias 1989; CitationKim and Garcia 1991; CitationKim and Fisher 1999). These idealized airway models were useful in understanding the flow pattern and deposition mechanisms. Realistic airway replicas made from cadavers have also been used in deposition studies (CitationSchlesinger and Lippmann 1972; CitationSchlesinger et al. 1977; CitationGurman et al. 1984; CitationChan et al. 1978; CitationCohen et al. 1990; CitationCheng et al. 1999; CitationSussman et al. 1991; CitationSmith et al. 2001). Deposition data obtained from realistic airway replicas had large variations and were more difficult to interpret, especially in terms of comparison with theoretical models. However, it is important to understand the differences in deposition patterns between a realistic airway model and those of an idealized airway model.
Several theoretical models have also been developed to predict initial deposition in the tracheobronchial airways. Deposition models for bends have been developed that showed that the Stokes number, bend angle, and curvature ratio are the main parameters in the analytical expression of the deposition (CitationCheng and Wang 1975; CitationYeh et al. 1976; CitationDiu and Yu 1980; CitationCheng et al. 1999). CitationBalásházy et al. (1990) have improved the bend model by considering different flow profiles in the bend and by showing ways to adopt a simple bend to the bifurcation geometry. Based on a stopping distance concept, CitationCai and Yu (1988) showed that inertial deposition in an idealized symmetrical bifurcation is a function of Stokes number, bifurcation angle, and ratio of parent and daughter tube diameters. Deposition equations derived from these models have been used in lung deposition models to predict the deposition pattern in the human respiratory tract.
A number of authors have reported using computer simulation to study particle deposition in the human lung. Hofmann and Koblinger (Citation1990a, Citation1990b, Citation1992) used a Monte Carlo method to model aerosol deposition in the human lung. CitationFerron et al. (1991) simulated air flow with heat and water vapor transported into two-dimensional bifurcations. CitationBalásházy et al. (1991) and CitationEisner (1993) also studied particle deposition in airway bifurcation models. CitationBalásházy (1994) analyzed the particle trajectories in a three-dimensional bronchial airway bifurcation. These investigators used a Navier-Stokes solver but neglected the effects of turbulence. CitationMartonen et al. (1993) studied the fluid dynamics of the human larynx and upper TB airways using a numerical technique. They found that the formation of the laryngeal jet strongly affects airflow patterns in the trachea, the bifurcation zone, and the main bronchi. While their computation included a standard k-ϵ turbulence model, they did not report any results concerning the intensity of the turbulence. CitationAsgharian and Anjilvel (1994) numerically simulated the inertial and gravitational deposition of particles in a square cross section of a bifurcating passage. CitationLi and Ahmadi (1995) performed detailed computer simulations of particle transport and deposition in the first lung bifurcation. They showed that airway turbulence strongly affects the particle deposition rate. In addition, the importance of accurate anisotropic modeling of the directional intensities of turbulence was emphasized for the correct evaluation of the deposition rate. Based on the laminar-to-turbulent airflow analysis of Zhang and Kleinstreuer (2003a, 2003b), they and CitationZhang et al. (2004) simulated and analyzed airflow structures and particle depositions in a representative human upper airway model, i.e., from mouth to the third generation of the TB tree. Their numerical report was in good agreement with data obtained from idealized airway models.
In this article we describe aerosol deposition in a realistic human airway replica as a function of flow rate and particle size. The deposition pattern was compared to several theoretical models and experimental data obtained from idealized airway models and glass bifurcation models. Comparison with theoretical models was important because some of these deposition models have been used to calculate lung deposition patterns of inhaled particles.
MATERIAL AND METHODS
Airway Replica
shows the human airway cast used in the present study, including the oral cavity, pharynx, larynx, trachea, and four generations of bronchi. The oral portion of the cast was molded from a dental impression of an oral cavity of a human volunteer, while the other airway portions of the cast were made from a cadaver (CitationCheng et al. 1997). The process of making the replica, and the dimension of the oral cavity and the particle deposition in this area were also investigated by CitationCheng et al. (1999). The production mold for our cast was made using Dow-E silicone rubber (Dow Corning, Midland, MI, USA). Wax was poured into the production mold; after the wax solidified, the cast was carefully taken out. To minimize the effect of electrostatic deposition, an electrically conductive silicone rubber compound ((KE-4576, one-component RTV) GemTech, Grapevine, TX, USA) was applied to the wax mold used to make the cast. In the deposition experiment, the inside wall of the cast was coated with silicon oil (Dow Corning 550) to minimize particle bounce. This study focused on the results of particle deposition in the TB region of the cast. As shown in , the TB cast was cut into several segments after the experiment to investigate the local particle deposition. In order to compare our results with other studies, the cast was separated into trachea (generation 0) and four generations. The sum of segments 8D, 9D, and 10D was considered as trachea, which has no bifurcation in this region. From the first to the fourth generation, the upper bifurcation was considered in the corresponding generation. For this TB cast, the first generation only has one daughter tube (13F). Another one is right-connected with the second bifurcation (14G), which is considered in the second generation. Only two bifurcations in the fourth generation (32K and 33K) are included in the TB cast. The rest of the terminal bifurcations (20I, 22I, and 23I) belong to the third generation. lists the dimensions of each part of the TB cast, including the angles of each bifurcation. Airflow distribution in each terminal tube of the cast was measured using a hot-wire anemometer probe (Model 8470, TSI Inc., St. Paul, MN, USA). Velocity measurements at terminal segments were made at flow rates of 15, 30, and 60 l min− 1. The overall flow distribution in our model was approximately 62% to the right lung and 38% to the left lung, similar to what has been reported by CitationCohen et al. (1990).
Table 1 Airway dimensions
Aerosol Generation and Experimental Set-Up
Nine different sizes of polystyrene latex fluorescent particles in the size range of 0.93–30 μ m were used in this study (0.93, 1.79, and 2.79 μ m from Polysciences, Warrington, PA, USA; 4.0 μ m from Interfacial Dynamics, Portland, OR, USA; and 6.07, 9.80, 16.0, 23.8, and 30 μ m from Duke Scientific, Palo Alto, CA, USA). Particle sizes were determined using an optical or electron microscope and image analyzer. Particles > 5 μ m were generated as a dry powder from a small-scale powder disperser (Model 3433, TSI Inc.) (CitationChen et al. 1995), and particles < 5 μ m were generated from a Respirgard II® nebulizer (Marquest Medical Products Inc., Englewood, CO, USA). The aerosols passed through a Kr-85 tube to bring the particle charge to Boltzmann's equilibrium, and then entered the TB cast through the oral cavity. Each terminal tube of the cast was connected to a filter (Versapor® 3000, Gelman Sciences, Bedford, CT, USA) and rotameter. The flow rate through each branch was determined prior to the particle deposition study with a velocity transducer (Model 8170, TSI Inc.) at a total flow rate of 15, 30, and 60 l min− 1. The cast was cut into segments, as mentioned previously, after the deposition experiment. Fluorescent material in the particles that deposited in the cast and in the filter was extracted using ethyl acetate. The resultant solution was then filtered using a nylon membrane (0.2 μ m, Dow Corning, Midland, MI, USA) and the fluorescent content of the filtered solution was measured using a fluorescence spectrometer (Hitachi, Danbury, CT, USA). The deposition fraction in each airway segment was calculated from this measurement.
Theoretical Models
Three theoretical models were used to compare our experimental data in this study. These deposition models focused on inertial deposition, which should be the dominant deposition mechanism for the particle sizes used in this study. They were also used in lung deposition models to estimate regional deposition and dose for inhaled particles. Simple bend models have been used to estimate deposition in bifurcation of the TB region as well as in the oral cavity (CitationCheng and Wang 1975; CitationYeh et al. 1976; CitationCheng et al. 1999). A bifurcation can also be considered combining two bends together. Based on a rotational flow profile, the following equation that predicts the particle deposition efficiency (η) of a bend can be used to estimate deposition in an airway bifurcation:
A method was developed by CitationCai and Yu (1988) to predict the particle deposition in an idealized, symmetrical airway bifurcation. This method only considered the impaction and interception mechanisms based on the concept of the stop and interception distances of a particle in the cross section of the daughter tube. For uniform flow, the deposition efficiency can be obtained as follows:
The last theoretical model used in this study was developed by CitationBalásházy et al. (1990). They presented the simultaneous action of inertial impaction and gravitational forces on a particle moving in three-dimensional circular bends. The particle deposition efficiency can be obtained with the following expressions:
RESULTS
Deposition in the Tracheal Region
Particle deposition in the trachea depends on the flow in this region, which has been shown to be turbulent because of laryngeal jet. Our experimental data from the tracheal region were obtained in a realistic physical model containing the oral cavity and larynx. shows the deposition efficiency in the trachea for the different flow rates as a function of Stokes number. Our data were compared with results obtained using realistic airway casts with a mechanical larynx (CitationChan and Lippmann 1980) and without a larynx (CitationSchlesinger et al. 1977). Good agreement was evident for the two experimental data sets using a realistic cast with a larynx. The data from Schlesinger et al. showed lower deposition efficiency than that shown in the current study and the study by CitationChan and Lippmann (1980).
Figure 2 Comparison of tracheal deposition in the replica with reported lung cast deposition data. The fitted curve was based on current data and the data of CitationChan and Lippmann (1980) and CitationSchlesinger et al. (1977).
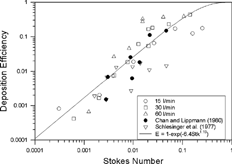
Deposition in the Upper Bronchial Region
shows the comparison of our deposition efficiency in the first generation with the data of CitationChan and Lippmann (1980) and several theoretical models (CitationBalásházy et al. 1990; CitationCai and Yu 1988; CitationCheng et al. 1999). Chan and Lippmann's results basically showed agreement with our results. Since this region of our lung cast is an uneven bifurcation that only has one daughter tube (13 F; ), the comparison with the theoretical model, which normally considered an even bifurcation, is not appropriate. However, because the particles mostly deposit in the carina of a bifurcation, one daughter tube could only slightly affect the deposition efficiency. The particle deposition efficiency of theoretical models from CitationCai and Yu (1988) and CitationBalásházy et al. (1990) are lower than our experimental data in the first generation. The deposition model, based on a bend, developed by CitationCheng et al. (1999) has better agreement with our results in this region. Furthermore, the slopes of all three models were basically the same and were smaller than the experimental data.
Figure 3 Comparison of the deposition efficiency in the first generation of the replica with reported lung cast deposition data and theoretical models.
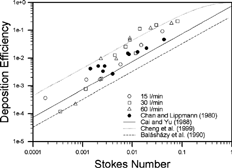
There are two bifurcations in the second generation. The dimensions of these two bifurcations are different, as shown in . gives the particle deposition efficiency in the second generation of the lung cast. Because there is more than one bifurcation after the first generation, and each bifurcation has its own dimension, the multiple lines may apply for the models of CitationCai and Yu (1988) and CitationBalásházy et al. (1990). It also shows the experimental data from CitationChan and Lippmann (1980) and the theoretical predictions in this generation for the comparison. The deposition efficiency of Chan and Lippmann's model in this region was in good agreement with our data. Cai and Yu's model (1988) predicted very well the particle deposition efficiency in the Stokes number range of 0.005–0.3. However, it predicted high deposition for the Stokes number below 0.01. The model of CitationBalásházy et al. (1990) predicted lower deposition efficiency than our experimental data, whereas the model of CitationCheng et al. (1999) gave a slightly higher prediction. The slopes of these three numerical models varied with the slope of the experimental data in the Stokes number below 0.01.
Figure 4 Comparison of the deposition efficiency in the second generation of the replica with reported lung cast deposition data and theoretical models.
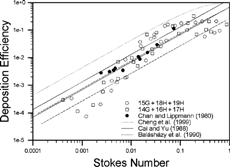
Kim and colleagues also did some experimental studies for the particle deposition in the idealized bifurcations (CitationKim and Iglesias 1989; CitationKim and Fisher 1999). Their studies were based on asymmetrical glass bifurcations with the dimensions approximating the third- to fifth-generation human bronchial airways of CitationWeibel's (1963) model. Our experimental results in the third and fourth generation were also compared with the data from CitationKim and Fisher (1999) and CitationKim and Iglesias (1989). The lung replica used in this study is a four-generation lung cast, while the fourth generation is incomplete. Although there were only two bifurcations in the fourth generation, the particle deposition data in these two bifurcations was assumed to represent each bifurcation in this generation. Figures and show the particle deposition results of our study in the third and fourth generations, respectively, compared with other experimental data and numerical models. Our data showed very good agreement with the results of CitationChan and Lippmann (1980) in both the third and fourth generations. However, both the third and fourth generations' data from CitationKim and Fisher (1999) and CitationKim and Iglesias (1989) were lower than the experimental data obtained by CitationChan and Lippmann (1980) and this study.
NEW EMPIRICAL MODEL
Tracheal Region
Because there is no theoretical model to predict the particle deposition in the tracheal region, the following equation can be used to fit our experimental data and the data obtained by CitationChan and Lippmann (1980):
Bronchial Airways
Particle deposition in each generation (excluding trachea) of the lung cast showed good agreement with literature values, especially the results of CitationChan and Lippmann (1980), in which a realistic lung cast was used. However, the comparison with three deposition models was less satisfactory. Cai and Yu's model (1988) was close to our data but still could not predict the deposition efficiency with the Stokes number below 0.005. Another disadvantage of their model is that the deposition efficiency could be over 100% for a large Stokes number. Thus, Equation (Equation6) was also considered as the basic expression to predict the particle deposition efficiency in each bifurcation of the cast.
The experimental data for each bifurcation was regressed with Equation (Equation6), and the result for the constant values a and b is listed in . Since the value b was relatively constant between 1.2 and 1.42 for every bifurcation of the cast, we considered b as a constant value, which is not changed with the dimension of the bifurcation. The average b value, 1.34 ± 0.08, can be used in Equation (Equation6). The value a, however, was changed with the bifurcation size from 2.7 to 11.6. It is very difficult to find the relationship of value a with the cast size. The only relationship we obtained is the F value from of Cai and Yu's model (1988), which is the function of the bifurcation angle and diameter of the parent and daughter tubes. also lists the F value for each bifurcation, and shows value a as a function of value F. All data were related with a linear curve except two data points: the first generation, and bifurcation 14G of the second generation. A linear equation of a = 5.39F can be obtained if those two points were ignored. Thus, Equation (Equation6) can be simply expressed:
Figure 7 The constant value a in Equation (Equation6) as a function of F value obtained in Equation (Equation3). The solid circles were not regressed.
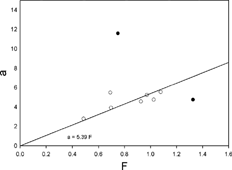
Table 2 Constant values obtained by data regression from Equation (Equation6) for each bifurcation of the lung cast
To check the validity of the empirical model listed in Equation (Equation7), the model was compared with all experimental data for the deposition efficiency in each bifurcation. shows all four generations but only one bifurcation for each generation. Since the empirical model is based on the dimensions of a bifurcation, the bifurcations in the same generation could have different expressions. The lung cast used in this study has a total nine bifurcations within four generations. Thus, nine expressions were obtained in this study. The current model was also applied to the construction of the lung cast used by CitationChan and Lippmann (1980). compares all lung cast data, including Chan and Lippmann's data, with the empirical model developed in this study. All expression lines obtained by generations of our and Chan and Lippmann's lung cast were between the solid lines in and have good agreement with the experimental data.
Figure 8 Comparison of experimental data with developed empirical model in four generations. Only one bifurcation is shown for generations two to four.
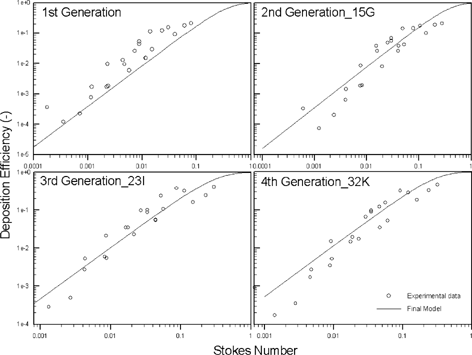
Figure 9 Comparison of all deposition data from the current study and the data from CitationChan and Lippmann (1980) with the developed empirical model.
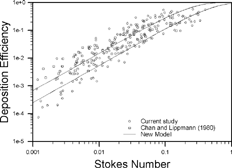
DISCUSSION
Our results show similar deposition efficiency to the reported data in the experimental studies. Those studies were based on the idealized bifurcation models or lung cast models. Our results showed good agreement with the data reported by CitationChan and Lippmann (1980), who also used a lung cast in their study (Figures , , , and ). In the tracheal region, the deposition efficiency with the larynx effect is generally larger than without it. This indicates that the larynx affects the flow pattern of the tracheal region, causing more particles to be deposited here. Because the cast used by CitationChan and Lippmann (1980) has a different configuration compared to the cast we used, there is still a slight difference for the particle deposition in the third generation. However, in the other generations very good agreement was obtained when comparing our data with theirs. In addition to comparing our lung cast data with that of Chan and Lippmann, we also compared our results with the data reported by CitationKim and Fisher's (1999) idealized glass bifurcation models. Because the glass bifurcations only represented the third to fifth generation of CitationWeibel's (1963) lung model, comparison was made only to the third and fourth generations (Figures and ). Results showed that Kim and Fisher's data were slightly lower than the deposition data obtained in this study. This may be due to several reasons, including the fact that the glass models were symmetric and had smooth surfaces, which means they were likely to have lower deposition than the realistic airway replicas with asymmetrical bifurcation and rough surfaces. Also, the idealized glass models do not include a larynx. We already showed that inclusion of the larynx is important in enhancing deposition in the trachea by simulating the turbulent flow conditions. It is likely that the turbulence induced by the laryngeal jet could persist in the first few generations of the tracheobronchial airways, resulting in higher deposition efficiencies. As shown in Figures , , and , we also compared our results to several theoretical deposition models based on the bend model or idealized bifurcation models. Comparing our data with that of the theoretical models indicated that our data were in general agreement with the experimental data, especially that reported by CitationCai and Yu (1988). This comparison indicated that these deposition equations for inertial mechanism were reasonable for the deposition calculation.
However, the agreement was not consistent over the entire range of the Stokes number. This was because the relationship between the deposition efficiency in the airways and the Stokes number for the experimental data and theoretical models was different. In all three theoretical models the deposition efficiency is approximately linearly related to the Stokes number (η ≅ Stk). In experimental measurements obtained in idealized models, as well as in realistic airway replicas, the deposition efficiency is related to the Stokes number as η ≅ Stka. The value of “a” ranged from 1.2 to 1.4 for this study and data reported by CitationChan and Lippmann (1980). For the idealized glass bifurcation model the value of “a” was reported as 1.5 (CitationKim and Fisher 1999). This was to be expected because the theoretical model was based on idealized bend or bifurcation models. Also, the flow profiles used in the theoretical model were either uniform flow or fully developed flow. The secondary flow, which was evident in some studies (CitationSchroter and Sudow 1969), was not considered when developing these idealized models.
The empirical model that we developed based on the experimental data would probably improve the deposition calculation in the lung dosimetric models. The developed particle deposition model of the TB region can be combined with the oral cast model developed by CitationCheng et al. (1999) as a whole airway model and applied to the different areas. The pharmaceutical application is a good example. Many medical inhalers are designed to use the oral inhalation mode to deliver drugs to the TB and/or lower region to treat some respiratory diseases. The present study will be a good model for evaluating the drug delivery efficiency of medical inhalers. The current model can also be used to investigate particle inhalation dosimetry and deposition patterns of air pollutants and occupational hazards.
Acknowledgments
The authors are grateful to G. A. Feather and B. Chen for technical assistance, and V. Fisher and B. Cleveland for reviewing this manuscript. This research was supported by the U.S. National Institute of Occupational Safety and Health, Grant R01 OH03900.
Notes
*Calculated from Equation (16) of CitationCai and Yu (1988).
REFERENCES
- Asgharian , B. and Anjilvel , S. 1994 . A Monte Carlo Calculation of the Deposition Efficiency of Inhaled Particles in Lower Airways . J. Aerosol Sci. , 25 : 711 – 721 .
- Balásházy , I. 1994 . Simulation of Particle Trajectories in Bifurcating Tubes . J. Comput. Phys. , 110 : 11 – 22 . [CROSSREF]
- Balásházy , I. , Hofmann , W. and Martonen , T. B. 1991 . Inspiratory Particle Deposition in Airway Bifurcation Models . J. Aerosol Sci. , 22 : 15 – 30 . [CSA] [CROSSREF]
- Balásházy , I. , Hofmann , W. and Martonen , T. B. 1990 . Inertial Impaction and Gravitational Deposition of Aerosols in Curved Tube and Airway Bifurcations . Aerosol Sci. Technol. , 13 : 308 – 321 .
- Cai , F. S. and Yu , C. P. 1988 . Inertial and Interceptional Deposition of Spherical Particles and Fibers in a Bifurcating Airway . J. Aerosol Sci. , 19 : 679 – 688 . [CSA] [CROSSREF]
- Chan , T. L. and Lippmann , M. 1980 . Experimental Measurements and Empirical Modeling of the Regional Deposition of Inhaled Particles in Humans . Am. Ind. Hyg. Assoc. J. , 41 : 399 – 409 . [PUBMED] [INFOTRIEVE]
- Chan , T. L. , Lippmann , M. , Cohen , V. R. and Schlesinger , R. B. 1978 . Effect of Electrostatic Charges on Particle Deposition in a Hollow Cast of the Human Larynx-tracheobronchial Tree . J. Aerosol Sci. , 9 : 463 – 468 . [CROSSREF]
- Chen , B. T. , Yeh , H. C. and Fan , B. J. 1995 . Evaluation of the TSI Small-scale Powder Disperser . J. Aerosol Sci. , 26 : 1303 – 1313 . [CROSSREF]
- Cheng , K. H. , Cheng , Y. S. , Yeh , H. C. and Swift , D. L. 1997 . Measurements of Airway Dimensions and Calculation of Mass Transfer Characteristics of the Human Oral Passage . J. Biomed. Eng. , 119 : 475 – 482 .
- Cheng , Y. S. and Wang , C. S. 1975 . Inertial Deposition of Particles in a Bend . J. Aerosol Sci. , 6 : 139 – 145 . [CROSSREF]
- Cheng , Y. S. , Zhou , Y. and Chen , B. T. 1999 . Particle Deposition in a Cast of Human Oral Airways . Aerosol Sci. Technol. , 31 : 286 – 300 . [CROSSREF]
- Cohen , B. S. , Susman , R. G. and Lippmann , M. 1990 . Ultrafine Particle Deposition in a Human Tracheobronchial Cast . Aerosol Sci. Technol. , 12 : 1082 – 1091 .
- Diu , C. K. and Yu , C. P. 1980 . Deposition from Charged Aerosol Flows through a Pipe Bend . J. Aerosol Sci. , 11 : 397 – 402 . [CROSSREF]
- Eisner , A. D. 1993 . Universal Bifurcation Model and Its Application to Study Local Particle Transport Phenomena in Upper Airways . J. Aerosol Sci. , 24 : 537 – 549 . [CSA] [CROSSREF]
- Ferron , G. A. 1977 . Deposition of Polydisperse Aerosols in Two Glass Models Representing the Upper Human Airway . J. Aerosol Sci. , 8 : 409 – 427 . [CROSSREF]
- Ferron , G. A. , Hillebrecht , A. , Peter , J. , Priesack , E. , Thoma , M. , Kunzer , I. and Mederer , R. 1991 . Airflow Simulation in Two Dimensional Bifurcations . J. Aerosol Sci. , 22 : S809 – S812 . [CROSSREF]
- Gurman , J. L. , Lippmann , M. and Schlesinger , R. B. 1984 . Particle Deposition in Replicate Casts of Human Upper Tracheobronchial Tree Under Constant and Cyclic Inspiratory Flow: I. Experimental . Aerosol Sci. Technol. , 3 : 245 – 252 . [CSA]
- Hofmann , W. and Koblinger , L. 1990a . Monte Carlo Modeling of Aerosol Deposition in Human Lungs. Part I: Simulation of Particle Transport in a Stochastic Lung Structure . J. Aerosol Sci. , 21 : 661 – 674 . [CSA] [CROSSREF]
- Hofmann , W. and Koblinger , L. 1990b . Monte Carlo Modeling of Aerosol Deposition in Human Lungs. Part II: Deposition Fractions and Their Sensitivity to Parameter Variations . J. Aerosol Sci. , 21 : 675 – 688 . [CSA] [CROSSREF]
- Hofmann , W. and Koblinger , L. 1992 . Monte Carlo Modeling of Aerosol Deposition in Human Lungs. Part III: Comparison with Experimental Data . J. Aerosol Sci. , 23 : 51 – 63 . [CROSSREF]
- Kim , C. S. and Iglesias , A. J. 1989 . Deposition of Inhaled Particles in Bifurcation Airway Models: I. Inspiratory Deposition . J. Aerosol Med. , 2 : 1 – 14 .
- Kim , C. S. and Garcia , L. 1991 . Particle Deposition in Cyclic Bifurcation Tube Flow . Aerosol Sci. Technol. , 14 : 302 – 315 .
- Kim , C. S. and Fisher , D. M. 1999 . Deposition Characteristics of Aerosol Particles in Sequentially Bifurcating Airway Models . Aerosol Sci. Technol. , 31 : 198 – 220 . [CROSSREF]
- Li , A. and Ahmadi , G. 1995 . Computer Simulation of Particle Deposition in the Upper Tracheobronchial Tree . Aerosol Sci. Technol. , 23 : 201 – 223 . [CSA]
- Martonen , T. B. , Zhang , Z. and Lessmann , R. 1993 . Fluid Dynamics of the Human Larynx and Upper Tracheobronchial Airways . Aerosol Sci. Technol. , 19 : 133 – 156 . [CSA]
- Myojo , T. 1987 . Deposition of Fibrous Aerosol in Model Bifurcation Tubes . J. Aerosol Sci. , 18 : 337 – 347 . [CROSSREF]
- Myojo , T. 1990 . The Effect of Length and Diameter on Deposition of Fibrous Aerosol in a Model Lung Bifurcation . J. Aerosol Sci. , 21 : 651 – 659 . [CSA] [CROSSREF]
- Schlesinger , R. B. and Lippmann , M. 1972 . Particle Deposition in Casts of the Human Upper Tracheobronchial Tree . Am. Ind. Hyg. Assoc. J. , 33 : 237 – 251 . [PUBMED] [INFOTRIEVE]
- Schlesinger , R. B. , Bohning , D. E. , Chan , T. L. and Lippmann , M. 1977 . Particle Deposition in a Hollow Cast of Human Tracheobronchial Tree . J. Aerosol Sci. , 8 : 429 – 445 . [CROSSREF]
- Schroter , R. C. and Sudlow , M. F. 1969 . Flow Patterns in Models of the Human Bronchial Airways . Respir. Physiol. , 7 : 341 – 355 . [PUBMED] [INFOTRIEVE] [CROSSREF]
- Smith , S. M. , Cheng , Y. S. and Yeh , H. C. 2001 . Deposition of Ultrafine Particles in Human Tracheobronchial Airways of Adults and Children . Aerosol Sci. Technol. , 35 : 697 – 709 . [CROSSREF]
- Sussman , R. G. , Cohen , B. S. and Lippmann , M. 1991 . Asbestos Fiber Deposition in Human Tracheobronchial Cast. I. Experimental . Inhal. Toxicol. , 3 : 145 – 160 . [CSA]
- Weibel , E. R. 1963 . Morphometry of the Human Lung. , New York : Academic Press .
- Yeh , H. C. , Phalen , R. F. and Raabe , O. G. 1976 . Factors Influencing the Deposition of Inhaled Particles . Environ. Health Perspect. , 15 : 147 – 156 . [PUBMED] [INFOTRIEVE]
- Zhang , Z. and Kleinstreuer , C. 2003a . Modeling of Low Reynolds Number Turbulent Flows in Locally Constricted Conduits: A Comparison Study . AIAA J. , 41 : 831 – 840 .
- Zhang , Z. and Kleinstreuer , C. 2003b . Species Heat and Mass Transfer in a Human Upper Airway Model . Int. J. Heat Mass Transfer , 46 : 4755 – 4768 . [CSA] [CROSSREF]
- Zhang , Z. , Kleinstreuer , C. , Kim , C. S. and Cheng , Y. S. 2004 . Vaporizing Micro-Droplet Inhalation, Transport and Deposition in a Human Upper Airway Model . Aerosol Sci. Technol. , 38 : 36 – 49 .