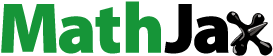
ABSTRACT
Norway spruce (Picea abies) is a widely used Christmas tree species in the Nordic countries. Postharvest needle retention is an important characteristic for Christmas trees and compared to many fir (Abies) species, Norway spruce has poor postharvest needle retention. This trait is one of the most important qualities in choice of natural versus plastic trees. In this study, current year shoots were cut from 30 Norway spruce seedlot sources, including the most widely used Norwegian Christmas tree provenances, and tested to identify genetic variation in postharvest needle retention. Current year shoots were collected from one field in November and December 2018, and from three fields in October, November and December 2019. The current year shoots were displayed indoors under controlled conditions and allowed to dry. Differences in postharvest needle retention were seen between seedlots, harvesting dates and locations. Our study indicates possibilities of selecting for improved postharvest needle retention in Norway spruce seed sources. Furthermore, postharvest needle retention should be considered as one characteristic to add in the ongoing Norway spruce Christmas tree breeding program.
Introduction
Norway spruce [Picea abies (L.) Karst] is among the most popular Christmas tree species in the Nordic countries. As a native species, Norway spruce traditionally used to be the main Christmas tree in northern Europe. However, in the 1980s, more luxurious true fir (Abies) trees with better postharvest needle retention were introduced in Europe and also in Norway where it has steadily increased in popularity (Leivsson Citation1987; Strande Citation2015).
In Norway, the tradition of using Norway spruce has remained strong and it is still the most cultivated Christmas tree together with subalpine fir [Abies lasiocarpa (Hook.) Nutt.] and Nordmann fir [A. nordmanniana (Stev.) Spach.]. Currently, the Norwegian Christmas tree market consists of approximately 40% Norway spruce (Strande Citation2015). There are several advantages in cultivating Norway spruce compared with fir trees. It is fast growing and adapted to the Norwegian climate, meaning it is hardy, tolerate many soil types, get less damaged by wildlife and it is more resistant against pathogens within the genera Phytophthora (Pettersson et al. Citation2019). However, worldwide the use of Norway spruce as a Christmas tree has steadily decreased compared to fir, probably due to needle loss problems (Wilmot et al. Citation2017). Poor postharvest needle retention in Norway spruce prevents extended display periods, which is a major limitation when consumers buy their trees early and want to maintain the “tree freshness” until the beginning of January.
Chastagner et al. (Citation2000, Citation2004) have shown that cut branches displayed dry can predict the postharvest needle retention characteristics of whole Christmas trees. For many fir species, postharvest needle retention experiments have been conducted over many years to select the best material, and it has been shown that progenies from superior trees have improved postharvest needle retention (Nielsen and Chastagner Citation2005a). Skúlason et al. (Citation2018) tested postharvest needle retention of 26 provenances of subalpine fir and corkbark fir [A. lasiocarpa var. arizonica (Merriam) Lemmon] for Christmas tree production and found significant variation between provenances. They concluded that it is important to select the provenances which can increase product value by selecting those with better postharvest needle retention.
Normally, tree species with large distribution areas have a wide variation in phenology and other traits (Langlet Citation1963), hence one can expect a large variation for Norway spruce, also in postharvest needle retention. A wide variety of Norway spruce seed sources are used for Christmas tree production in Norway. As in forestry, it is important to use the best seed sources available. Breeding for the Christmas tree industry is an expressed goal of the Norwegian Forest Seed Center (Edvardsen Citation2010). Large economic gains can potentially be achieved if the best trees adapted for Christmas tree production are used (Nielsen et al. Citation2011). However, so far, postharvest needle retention has not been a part of the regularly selection and breeding program for Christmas trees in Norway (Nyeggen and Skage Citation2001, Citation2002, Citation2005).
Even if growers harvest their trees at the same time every year (by the end of November or early December) the amount of postharvest needle loss varies. Proper cold acclimation of the trees before harvest has been shown in many studies to be important (Mitcham-Butler et al. Citation1988; Thiagarajan et al. Citation2012; MacDonald et al. Citation2014; MacDonald and Lada Citation2018). Fir trees harvested in early autumn (September, October) have poorer postharvest needle retention than trees harvested later in the autumn or in the winter (November, December) (Mitcham-Butler et al. Citation1988; Chastagner and Riley Citation2007; MacDonald and Lada Citation2008; MacDonald et al. Citation2014). However, no studies of postharvest needle retention and time of harvest has been reported for Norway spruce Christmas trees with potential seed sources for the Norwegian market.
In Scandinavia, there is clearly a demand for traditional, domestically produced and low environmental impact Christmas trees. Norway spruce matches these criteria very well, however, poor postharvest needle retention is an issue for the consumers and one of the main reasons why fir is selected over Norway spruce or why costumers choose plastic Christmas trees. Therefore, there is a need to investigate variation in postharvest needle retention for Norwegian seed sources of Norway spruce to identify both superior seed sources and breeding trees. A huge amount of work has been done to explore seed sources with a high potential to deliver high quality Christmas trees (Nyeggen and Skage Citation2001, Citation2002, Citation2005). Due to harsh growing conditions in Norway, production of exotic Christmas trees is limited by climatic boundaries, e.g. production of Nordmann fir occurs mainly in coastal regions of the most southern part of Norway due to low winter temperature inland (Fløistad et al. Citation2015). Growing areas for subalpine fir are also limited by harsh winter climate (Fløistad et al. Citation2017). Identifying Norway spruce material with better postharvest needle retention may increase the domestic production of Christmas trees and thereby decrease the import. According to Strande (Citation2015), approximately 20% of the Christmas trees are imported to Norway.
Based on the increasing interest in Christmas tree production in Norway, the objective of our research was to determine seed source variation of Norway spruce concerning postharvest needle retention. In an initial study in 2018 and a larger study the following year, we tested postharvest needle retention of 30 seedlots of Norway spruce, most of them seedlots used for Christmas tree production.
Material and methods
The 30 Norway spruce seedlots we tested are currently evaluated in a Christmas tree field trial in Southern Norway. The experiments took place in November and December 2018 and October, November and December 2019.
Plant material and site description
In the spring of 2014, a Norway spruce Christmas tree trial was established by the Norwegian Forest Seed Center. Parallel fields were established at eight locations in southern Norway. In the trial, a total of 30 seedlots were represented, most of them in all the parallel fields. Hereof five seedlots came from controlled crossings, 17 from open pollinated clones and eight provenances (free pollination) ().
Table 1. Norway spruce (Picea abies) seed sources and seedlots represented in the fields near Sandefjord, Stavsjø and Brumunddal.
The trial is currently evaluated for growth traits. In our postharvest needle retention experiment, only trees from one field in 2018 and three fields in 2019 were used (). A field in Sandefjord municipality [59°12 = N, 10°13 = E, 100 meters above sea level (m a.s.l.)] was used both in 2018 and 2019, while two fields in Ringsaker municipality, Stavsjø (60°47 = N, 10°46 = E, 215 m a.s.l.) and Brumunddal (60°55 = N, 10°58 = E, 376 m a.s.l.) were only used in 2019. All field trials had been established using 2-year-old plug-plants in a randomized complete block design with five replicates, each with nine trees per replicate and seedlot. The trees were planted in a 3 × 3 replication on a 1.3 × 1.3 m spacing. Seedlings where produced at Skogplanter Midt- Norge AS at Skjerdingstad nursery. The Brumunddal test field contained all 30 seedlots, while Sandefjord and Stavsjø contained 26 and 21 seedlots, respectively ().
Figure 1. Location of Christmas tree test fields included in a postharvest needle retention experiment of Norway spruce (Picea abies) in southeastern Norway. The fields were located in Sandefjord municipality and Ringsaker municipality (field Stavsjø and Brumunddal).
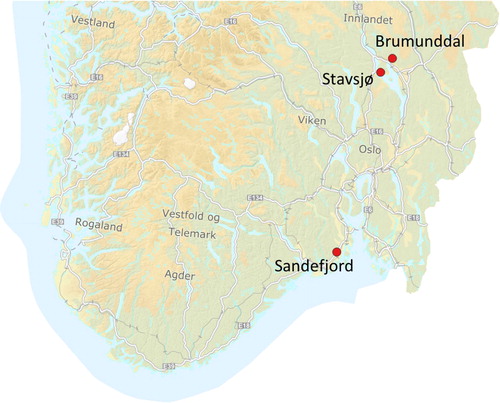
Weather data (daily and monthly mean temperatures) during the periods of the experiments were obtained from the nearest weather station for each field (Agrometeorology Norway Citation2020). Those were Ramnes, Kise and Ilseng meteorological stations for field Sandefjord, Stavsjø and Brumunddal, respectively. The daily mean temperature norms and days with frost for the period 1961–1990 where collected from Eklima web portal (Norwegian Meteorological Institute Citation2020).
Tree management in the field
Different management had been applied in the fields at Sandefjord, Stavsjø and Brumunddal regarding weed control and fertilization. Wildlife (roe deer or moose) damage had occurred on some trees in Sandefjord in 2018. Some trees in Brumunddal 2019 were slightly trimmed by the grower. These treatments influenced only few of the current year shoots selected for our experiment. No chemical pest control had been conducted in either field.
Collection of current year shoots
In both 2018 and 2019 current year shoots were collected on lateral branches from the third uppermost branch whorl. For all fields, one current year shoot from 12 trees per seedlot were harvested. For Sandefjord, one current year shoot was harvested from six randomly selected trees in two blocks of each seedlot and year. The same trees were sampled in 2018 and 2019. For Stavsjø and Brumunddal, one current year shoot was harvested from three randomly selected trees in four blocks of each seedlot. The total number of shoots collected from each individual tree in Sandefjord was five, i.e. one per month in November and December 2018 and one per month in October, November and December. For Stavsjø 2019 and Brumunddal 2019, totally three shoots were collected per tree, i.e. one per month in October, November and December.
Experiment 2018
In 2018 (the first year of the study), current year shoots were harvested 15 November and 6 December from the field near Sandefjord. The current year shoots, including previous year shoot as a “handle”, were cut with secateurs and placed into labeled paper bags, transported back to NIBIO, Ås and stored in a covered space outdoors overnight. They were set up in a display room the following day. The shoots were arranged in the same block structure as in the field.
Experiment 2019
In 2019, current year shoots were harvested from the three fields (Sandefjord, Stavsjø and Brumunddal) during October, November and December. The current year shoots were cut in the same manner as in 2018 and directly placed in labeled multipot trays/containers [i.e. trays with small volumes per plug (1.4 × 1.4 × 3.3 cm = 6.5 cm3)], with capacity for holding the current year shoots by inserting the “handles” in the plugs using the same block structure as in the fields. For each field, all the current year shoots were harvested in one day, transported to the institute, kept outdoors and set up in a climate chamber (different room from 2018) the following day. Current year shoots were harvested 14, 15 and 16 October, 11, 12 and 13 November and 2, 3 and 4 December from Stavsjø, Brumunddal and Sandefjord, respectively.
Display room
Experiment 2018
In 2018, all current year shoots were arranged at room temperature in a display room at NIBIO. The current year shoots were displayed dry on a large table in three wooden wire-covered frames (120 × 80 × 10 cm) with 2 × 2 cm square grids. The shoots were arranged in 13 rows using the field block structure. Three HOBO water temperature Pro V2 data loggers (Onset Company, Bourne, MA, USA), one on each wooden frame, continuously recorded the temperature and relative humidity (RH) in the room throughout the experiment.
Experiment 2019
In 2019, the current year shoots were displayed dry in a climate chamber in the multipot trays/containers where they already had been fixed in the field. The trays with shoots were arranged on one shelf per field.
Measurements
The length of the harvested lateral shoots was measured. Needle loss was rated on the 4th (only 2018), 6th, 8th, 11th, 13th, and 16th (only 2019) day after placing the shoots in the display room (starting and ending in the exact same order as the shoots were harvested in the field). Needle loss was rated by brushing top- and both side-shoots of the branch with thumb and forefinger together to remove any loose or shedding needles. The accumulated needle loss was registered on each measurement date using the same needle loss rating scale as Nielsen and Chastagner (Citation2005b). The scale goes from 0–7, where 0 = no needle loss, 1 = < 1%, 2 = 1–5%, 3 = 6–15%, 4 = 16–33%, 5 = 34–66%, 6 = 67–90% and 7 = 91–100% needle loss. For each field, harvest date and evaluation day, the average needle loss was calculated for the given seedlot.
Statistics
The following model was applied:where
is the observed response variable for tree j within seedlot i and block l at time k.
is an intercept,
is the fixed effect of seedlot i,
is a random effect of tree j within seedlot i and block l,
is the fixed effect of time k,
is the interaction between seedlot i and time k,
is a random effect of block l, β is a coefficient measuring the linear influence of the covariate
(the shoot length corresponding to
), and
is the usual error terms. The random variables
are assumed to be independent, normally distributed with expected value zero and variances
respectively. Unknown parameters to be estimated are
.
Experiment 2018
The model above was fitted for the response variables needle loss for day 6, 8 and 11. For these three response variables the residual plots did not indicate any serious deviations from the usual assumptions.
Experiment 2019
The same model was fitted for the response variables needle loss for day 8, 11 and 13. For these three response variables the residual plots did not indicate any serious deviations from the usual assumptions. Where significant differences were detected, Tukey's multiple comparison method with 5% significance level was used for grouping of the seedlots and the harvesting times.
Data analysis were carried out using the program Minitab® 18 to determine significant main or interaction effects at a significance level of α = 0.05. Analysis of variance (ANOVA) was carried out using the GLM procedure for providing p-values for all effects in the models. Components of variance and needle loss values for the seedlots were estimated using the Mixed Effects Model procedure.
Results
Climatic conditions
The mean fall temperatures during September to December for both 2018 and 2019 were warmer than the norm for the period 1961–1990 (). The number of days with daily mean temperatures below zero (Tm < 0°C) in 2018 and 2019 were much fewer than the norm period 1961–1990 (). The first long frost period occurred in the end of November 2018 and beginning of November 2019, i.e. after the first harvest in both years (). In 2019, the daily mean temperatures for Stavsjø and Brumunddal were similar to each other (). The daily mean temperatures were slightly warmer for Sandefjord, the most southern site ().
Figure 2. Daily mean temperatures in September to December during 2018 (Sandefjord) and 2019 (Sandefjord, Stavsjø and Brumunddal). In 2018, current year shoots were harvested 15 November and 6 December from Sandefjord (short arrows). In 2019, current year shoots were harvested 14, 15 and 16 October, 11, 12 and 13 November and 2, 3 and 4 December from Stavsjø, Brumunddal and Sandefjord, respectively (long arrows). Data obtained from Agrometeorology Norway (Citation2020).
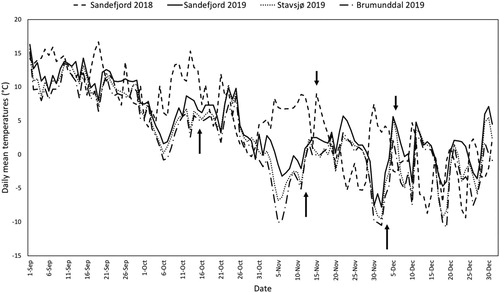
Table 2. Climatic conditions at the test fields.
There was no snow when collecting in October 2019, but a complete snow cover during November and December in Stavsjø and Brumunddal, and only a light snow cover in Sandefjord on these occations. At Stavsjø in December, all trees had received freezing rain that formed a thick ice cover on the branches which were further weighed down by snow. In the climate chamber, the ice melted but these current year shoots had a higher amount of needle loss at the first rating and the needles that fell off were discolored (yellow) from the middle to the top. This effect subsided as most of the yellowed needles fell off during the first two measurements (day 6 and 8).
Display room
In 2018, the display room held 23 ± 2°C and 35% RH. However, there was unfortunately a temperature increase in the display room from the first recording period of 15–28 November (21.8°C, 37.8% RH) to the second recording period of 6–19 December (23.9°C, 32.5% RH). This is the reason why we shifted display room in 2019.
For the larger study in 2019, the climate chamber held a steady temperature of 19.5 ± 1 °C and 60% RH throughout the experiment.
Length of current year shoots
In 2018, lateral shoot lengths were 6.5–27 cm with an average of 15.4 cm for Sandefjord. In 2019, the shoot lengths were 6–40, 4–39, 7–37 cm long with an average of 19.1, 18.8, 19.7 cm for Sandefjord, Stavsjø and Brumunddal, respectively.
Needle loss
Experiment 2018
At day 4 almost no needles were shed and at day 13 nearly all had fallen off for the majority of the seedlots, hence the variation was small between seedlots at those recording days. The Tukey's pairwise comparisons test displayed the biggest differences between seedlots after 8 days ().
Table 3. Results from analysis of variance and estimated components of variance for Sandefjord 2018 and Sandefjord, Stavsjø and Brumunddal 2019.
There was a significant difference in needle loss concerning time of harvest, where the harvest from December 6 had a higher amount of average needle loss (3.49), than the harvest from November 15 (3.04) (, ). Average needle loss ratings for November and December ranged from 2.49–4.74 (). Seedlots 6, 25, 4, 10, 21 and 15 had significantly better postharvest needle retention than seedlots 23 and 3, 2.49–2.62 versus 4.36 and 4.74, respectively (p < 0.0001).
Figure 3. Observed average needle loss values for 21 Norway spruce (Picea abies) seedlots tested in November and December 2018 from a field near Sandefjord. The seedlots were sorted by the average test results obtained in the larger study in 2019 across all three test periods (October, November, December) and all three fields (Sandefjord, Stavsjø and Brumunddal) (see ). Needle loss was recorded using a scale from 0–7, where 0 = no needle loss, 1 = < 1%, 2 = 1–5%, 3 = 6–15%, 4 = 16–33%, 5 = 34–66%, 6 = 67–90% and 7 = 91–100% needle loss (Nielsen and Chastagner Citation2005b).
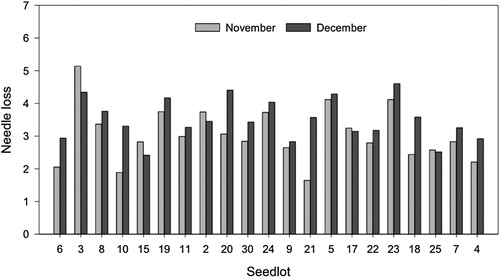
Table 4. Mean needle loss values per seedlot after eight days in a display room for samples (current year shoots) collected in a field near Sandefjord 15 November and 6 December 2018. Needle loss was measured using the same needle loss rating scale as Nielsen and Chastagner (Citation2005b). See for needle loss scale.
There was a significant influence of shoot length on needle loss, where the longer shoots performed better (). There was also a significant interaction effects for seedlots x time on needle loss ().
Experiment 2019
At day 6 almost no needles had been shed, and at day 16 almost all needles had been shed for the majority of the seedlots, hence the variation between seedlots was small at these times. This was the case for all three harvest dates. For the recording on day 11, there was a significant difference between seedlots in mean needle loss values for the average of October, November and December () for all three fields. Also, there was a significant interaction effect for seedlots x time on needle loss for all three fields (). The variation within seedlots () was larger than between seedlots (
) for all three fields ().
Sandefjord
There was a significant difference in needle loss for time of harvest, where October had the highest amount of average needle loss, 4.86, followed by December, 3.20, and November, 2.72 (, ).
Figure 4. Average needle loss values for three fields (Sandefjord, Stavsjø and Brumunddal) and three different harvest dates (October, November and December 2019). Current year shoots of Norway spruce (Picea abies) were harvested 14, 15 and 16 October, 11, 12, 13 November and 2, 3, 4 December from Stavsjø, Brumunddal and Sandefjord, respectively. See for needle loss scale. Different letters indicate significant differences within fields (Sandefjord = abc, Stavsjø = ABC and Brumunddal = abc).
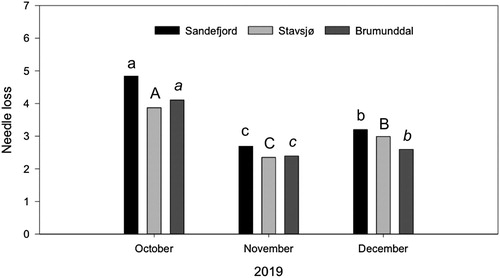
For the Tukey's pairwise comparisons test, which displayed the biggest differences between seedlots after 11 days, the needle loss ratings ranged from 2.47 to 4.78 (). Seedlot 6 had significantly better postharvest needle retention than seedlots 22, 12, 16, 23 and 4, 2.47 versus 3.97–4.78, respectively (p < 0.0001) (, ).
Figure 5. (a, b, c and d) Observed average needle loss values for 21 Norway spruce (Picea abies) seedlots tested in October, November and December 2019 at three different fields, Sandefjord, Stavsjø and Brumunddal. The seedlots are sorted by their average needle loss value across all three test periods and fields with highest needle loss on the right. See for needle loss scale.
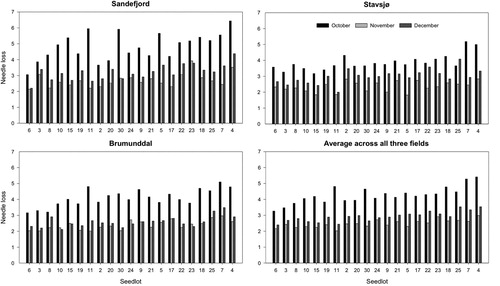
Table 5. Mean needle loss values per seedlot after 11 days in a display room for samples (current year shoots) collected at three fields (Sandefjord, Stavsjø and Brumunddal) in October, November and December 2019. Needle loss was measured using the same needle loss rating scale as Nielsen and Chastagner (Citation2005b). See for needle loss scale.
There was a significant influence of shoot length on needle loss, where the longer shoots performed better ().
Stavsjø
There was a significant difference in needle loss for time of harvest, where October had the highest amount of average needle loss, 3.87, followed by December, 2.99, and November, 2.35 (, ).
The Tukey's pairwise comparisons test displayed the biggest differences between seedlots after 11 and 13 days, displayed dry. The ranking of seedlots did not differ between day 11 and 13 and data from day 11 were therefore used to be able to compare data between fields. The needle loss ratings ranged from 2.48–3.72 (). Seedlot 15, had significantly better postharvest needle retention than seedlot 4, 2.48 versus 3.72 (p = 0.0115) ().
There was no significant influence of shoot length on needle loss ().
Brumunddal
There was a significant difference in needle loss for time of harvest, where October had the highest amount of average needle loss, 4.10, followed by December, 2.59, and November, 2.40 (, ).
The Tukey's pairwise comparisons test displayed the biggest differences between seedlots after 11 days when needle loss ratings ranged from 2.49–3.85 (). Seedlot 13 had significantly better postharvest needle retention than seedlot 7, 2.49 versus 3.85. Seedlot 7 had significantly poorer postharvest needle retention than 13, 6 and 3, 3.85 versus 2.49–2.51 (p = 0.0003) ().
There was a significant influence of shoot length on needle loss, where the longer shoots performed better ().
Sandefjord, Stavsjø and Brumunddal
In 2019, the mean values for needle loss of all seedlots for October were 4.86, 3.87 and 4.10 for Sandefjord, Stavsjø and Brumunddal, respectively (). In November and December there was less variation in needle loss with mean values for November 2.72, 2.35 and 2.40, and for December, 3.20, 2.99 and 2.59, for Sandefjord, Stavsjø and Brumunddal, respectively.
We made an in depth analysis of three seedlots that remained approximately the same in rank throughout the experiment. In , needles loss from day 6–16 is presented for the three seedlots and all fields. Seedlot 6 had a low level of needle loss throughout the experiment (good), seedlot 30 remained in the middle (medium) while seedlot 7 had a high level of needle loss in 2019 and average needle loss in 2018 (poor) (). Other seedlots varied between years, e.g. seedlot 25 and 4 did poorly at all fields in 2019 but well in 2018.
Figure 6. Overview for three selected seedlots of Norway spruce (Picea abies), where seedlot 6 had good postharvest needle retention, seedlot 30 was average, and seedlot 7 had poor postharvest needle retention in both 2018 and 2019. A, B, C = October 2019, D, E, F = November 2019 and G, H, I = December 2019. Furthermore, A, D, G = seedlot 6, B, E, H = seedlot 30 and C, F, I = seedlot 7. See for needle loss scale. The figure is based on the observed mean needle loss values.
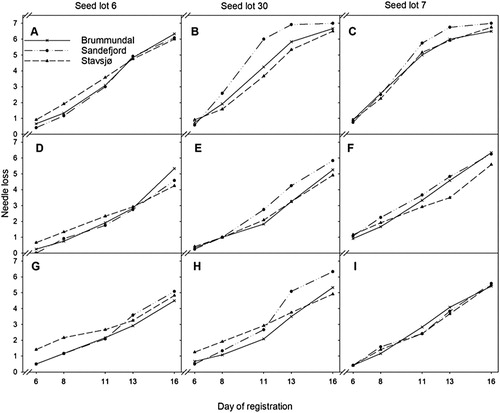
Discussion
Postharvest needle retention is an important characteristic for Christmas trees and, therefore, poor postharvest needle retention in Norway spruce may prevent early harvest and long transport distances. In our experiments, there were variations between fields and provenances like it is known from Nordmann fir (Nielsen and Chastagner Citation2005a, Citation2005b). Also, interaction between field and provenances commonly occurred as reported on subalpine fir by Skúlason et al. (Citation2018).
We found several seedlots consistently performing well in all three experimental fields. Samples from seedlot 6 had stable and good quality. The crossings from seed orchard Stange performed among the five best seedlots in all three fields. Seedlot 6 and 3 ranked 1st and 4th in all fields and at all sampling times in 2019, respectively, both controlled crossings with clone 5466 as the pollen source. Seedlot 1 (Clone 1641) did well in Brumunddal (5th place), but we had no data from Sandefjord and Stavsjø since they were not present in those fields. Seedlot 13 (Clone 5466), did best of all seedlots in Brumunddal (1st place) but average in Sandefjord. Others seedlots remained stable in their rating like seedlot 30 and 7 with medium and poor postharvest needle retention, respectively.
Some seedlots varied in their ranking between years. There was also a larger span of variation between seedlots in 2018 compared to 2019. The more homogeneous needle retention across seedlots in 2019 may partly be due to the more stable conditions in the display room than in 2018.
Instead of modeling and analyze the response variables for needle loss on different days separately it may be possible to build a model for the response variables for needle loss where day is modeled as a covariate. Then we may have a model with expected needle loss being a continuous function, linear or non-linear, of day. Including time and seedlot as in the model above, these expectation functions may depend on seedlot and time.
The majority of seedlots performed best at the field near Brumunddal. This is the northernmost field, with highest elevation and the lowest temperatures. In the field at Sandefjord, which is the most southern field, with the lowest elevation and highest temperatures, the majority of seedlots performed the poorest among the three fields included in the study. However, in addition to temperature and cold acclimation, there may be other explanations for the variation between the fields. Management, such as fertilizing and weeding and field characteristics, such as organic matter and soil mineral content has been reported to influence needle retention (MacDonald and Lada Citation2018). Fertilization and cultivation routines may explain the site variation found in our experiment, but probably not the within site variation. Stavsjø and Brumunddal are two closely located fields, and the mean needle loss values for the seedlots did not differ much for October (3.87, 4.11, respectively). The corresponding value for Sandefjord was 4.83, which is a much larger needle fall considering the scale used (Nielsen and Chastagner Citation2005b). The average needle abscission in November and December combined for Stavsjø and Brumunddal was 2.67 and 2.49, respectively, whereas needle abscission was 2.95 for Sandefjord. The higher increase in needle retention from October to November and December at the Sandefjord field, compared to the two other fields, remains unclear.
Considering the improvements in methodology from the first year, 2018, to the larger study in 2019, i.e. the change from fluctuating temperatures and humidity in the display room, to the climate chamber with controlled conditions, it is not realistic to compare the year to year variation in needle retention. Temperature and humidity seriously influence needle loss via respiration, where higher temperatures and dryer conditions accelerate respiration (Mitcham-Butler et al. Citation1988; MacDonald et al. Citation2012). Blankenship and Hinesley (Citation1990) showed that respiration in Fraser fir [A. fraseri (Pursh) Poir.] increased exponentially with increasing temperature. When spruce species dries to a certain moisture content, they typically experience very heavy needle loss (Hinesley and Chastagner Citation2004). When considering the overall needle retention at day 8 for November and December combined in Sandefjord 2018, the needle loss values were already larger, ranging from 2.49–4.74, than at day 11 in 2019, ranging from 2.17–3.94. We can, however, not exclude that mild temperature events in Sandefjord in November and December 2018 may explain a lower needle retention in that year compared to 2019 (MacDonald et al. Citation2014).
In a study from another Christmas tree field, also located in southeastern Norway, we compared needle retention of Norway spruce with Serbian spruce [Picea omorika (Panč.) Purk.] (both unknown provenances) using the same procedure as for the 2019 study, i.e. harvesting in October, November and December (unpublished data). When harvesting in November the mean needle loss after 11 days was 2.25 and 0.47 for Norway spruce and Serbian spruce, respectively. For this location, the average needles loss, across all harvesting dates, for day 16 in Norway spruce was 4.87, approximately the same as for Serbian spruce at day 32 (5.28). For Serbian spruce, the needle retention improved from October (6.08) to November (4.83) and December (4.92) when evaluated at day 32. This improvement from October to November and December is similar as for Norway spruce in our 2019 study. However, these evaluations on Serbian spruce were only done for one field. The difference in needle retention between the two species should be studied in greater detail.
In our larger study in 2019, we found that the overall needle retention improved from October (4.27) to November (2.48) and December (2.93), and this occurred in all the fields. This corresponds to findings by Chastagner and Riley (Citation2007) where fir trees harvested in early autumn (September and October) had lower needle retention than trees harvested in late autumn or winter (November and December). Skúlason et al. (Citation2018) showed that harvesting subalpine fir and corkbark fir in November was better than October with respect to postharvest needle retention. MacDonald et al. (Citation2014) identified November and December as an ideal time to harvest balsam fir [Abies balsamea (L.) Mill.] from a needle retention perspective, and Mitcham-Butler et al. (Citation1988) showed that Fraser fir collected in late November had improved needle retention compared to October.
Unlike October, in November and December, trees at all three locations had been exposed to several cold spells with temperatures below zero, hence they had developed a level of hardiness like described by Weiser (Citation1970), which is critical for improved needle retention after harvest (Hinesley and Chastagner Citation2004). For all three fields, November had the best needle retention but there was minor difference in needle retention between November and December. The ice cover on the shoots at Stavsjø in December was probably part of the reason for higher needle loss values there, due to damaging during harvest of the shoots. The significant interaction we found between seedlot and time of harvest, was probably due to rank changes of the seedlots. Based on the large variations between seedlots and the significant interaction, it is not possible to provide a clear recommendation on seed sources with high needle retention potential under all conditions. Further testing is needed.
Frost hardiness in conifers develops with increasing periods with low temperatures (Sakai and Larcher Citation1987), however mild spells in the hardening phase may interrupt the hardening (Granhus et al. Citation2009), and this may also be the case for needle retention. We therefore wonder if the loss in needle retention from November to December in 2019, especially at the field near Sandefjord, was partly due to an increase in temperatures before harvest. An extended study is needed to fully explore the relationship between cold acclimation and postharvest needle retention in Norway spruce.
The variation between current year lateral shoot lengths was large in our study. There was significantly less needle loss for longer shoots from Brumunddal and Sandefjord but that was not the case for Stavsjø (). It probably takes longer time for larger shoots to loose enough water to reach a critical moisture content and damage thresholds where needles start to shed. We suggest that future studies should be more careful concerning selection of similar sized current year shoots instead of focusing on harvesting from the third uppermost branch whorl (as in this study). Nielsen and Chastagner (Citation2005b) harvested tungs from the 3rd to 6th uppermost branch whorl of the main stem, a method that would have allowed us to compare shoots with similar length. We have not considered any non-linear relationship between shoot length and needle loss, nor any interactions between shoot length and the factors seedlot and time.
Since there is high demand for Christmas trees with excellent postharvest needle retention, our findings of some seed sources that performed well and stable concerning postharvest needle retention on samples from all three fields, indicate promising results for further breeding. It provides a potential for combining our results with other traits observed for Christmas tree values in the ongoing evaluation program by the Norwegian Forest Seed Center. Further work on Norway spruce postharvest needle retention is supported by the experience from several fir species that have gained improved needle retention due to targeted breeding for this characteristic (Nielsen and Chastagner Citation2005a, Citation2005b; Skúlason et al. Citation2018). Furthermore, growing of somatic embryogenesis plants provides a very interesting potential for production of individuals with the most decried characteristics (Egertsdotter Citation2019; Chen et al. Citation2020). Improving the postharvest needle retention of Norway spruce Christmas trees is also very important due to the fact that expansion of fir production may become limited due to susceptibility to several serious disease-causing organisms like Neonectria neomacrospora and Phytophthora spp. as well as lack of available land with suitable climatic conditions (Talgø et al. Citation2006, Citation2007; EPPO Citation2017; Nielsen et al. Citation2017; Skúlason et al. Citation2017). Norway spruce on the other hand is well adapted to the Norwegian climatic conditions and suffers from fewer lethal diseases and pests.
In addition to individual traits on the Christmas trees, growers should be aware of the potential influence of the environmental conditions prior to harvest and how this influence on postharvest needle retention.
Acknowledgements
The authors are grateful to Arjan Besemer at The Norwegian Forest Seed Center for valuable assistance regarding field selection, marking and harvesting. We are also grateful to the Christmas tree growers Bjørn Hageberg, Knut Helset, Yrjan Elias Fevang and Arnfinn Espenes, who cooperated in this effort. We also like to thank Eirik Nordhagen, Magnhild Sekse Erdal, Halvard Hole and Håvard Eikemo for practical and technical assistance.
Disclosure statement
No potential conflict of interest was reported by the authors.
References
- Agrometeorology Norway. 2020. [accessed 2020 Jan]. http://lmt.nibio.no.
- Blankenship SM, Hinesley LE. 1990. Tolerance of controlled atmosphere storage of cut Fraser fir and its respiration rate in air. HortScience. 25(8):941–943. doi: 10.21273/HORTSCI.25.8.941
- Chastagner GA, Nielsen UB, Riley K. 2000. The use of detached branches to identify sources of Nordmann fir with superior needle retention characteristics. In: Christensen CJ, editor. Improvements in Christmas tree and greenery quality. Skov & Landskab Rep. 7. Hørsholm: Danish Centre for Forest, Landscape and Planning; p. 26–32.
- Chastagner GA, Nielsen UB, Riley KL. 2004. Comparison of the postharvest quality of noble fir boughs from the United States and Danish provenances grown in Denmark. HortScience. 39(3):580–583. doi: 10.21273/HORTSCI.39.3.580
- Chastagner G, Riley K. 2007. Solving the needle loss nuisance – Christmas tree research examines the effect of harvest date on true fir needle retention. Gt Lakes Christmas Tree J. 2:36–39.
- Chen ZQ, Hai HNT, Helmersson A, Liziniewicz M, Hallingbäck HR, Fries A, Berlin M, Wu HX. 2020. Advantage of clonal deployment in Norway spruce (Picea abies (L.) H. Karst). Ann For Sci. 77(1):14. doi: 10.1007/s13595-020-0920-1
- Edvardsen ØM. 2010. Strategi for skogplanteforedling 2010–2040. The Norwegian Forest Seed Center (Skogfrøverket). p. 1–28. [accessed 2020 Jan]. http://skogplanteforedling.no/Dokumenter/Skogfroverket_strategi.pdf.
- Egertsdotter U. 2019. Plant physiological and genetical aspects of the somatic embryogenesis process in conifers. Scand J For Res. 34(5):360–369. doi: 10.1080/02827581.2018.1441433
- EPPO. 2017. Neonectria neomacrospora an emerging disease of fir trees in Northern Europe: addition to the EPPO alert list. EPPO reporting service-pest & diseases, 2017/120. [accessed 2020 Jan]. https://gd.eppo.int/reporting/article-6088.
- Fløistad IS, Nyeggen H, Skage J-O. 2015. Testing species of genus Abies for Christmas tree production in Norway. Scand J For Res. 30(8):653–663. doi: 10.1080/02827581.2015.1062131
- Fløistad IS, Nyeggen H, Skage J-O. 2017. Field trials with Abies lasiocarpa progenies from plus trees and seed orchard clones for Christmas tree production in Norway. Scand J For Res. 32(5):376–383. doi: 10.1080/02827581.2017.1297481
- Granhus A, Fløistad IS, Søgaard G. 2009. Bud burst timing in Picea abies seedlings as affected by temperature during dormancy induction and mild spells during chilling. Tree Physiol. 29(4):497–503. doi: 10.1093/treephys/tpn039
- Hinesley E, Chastagner G. 2004. Christmas trees. In: Gross KC, Wang CY, Saltveit M, editors. The commercial storage of fruits, vegetables, and florist and nursery stocks. USDA; p. 650–658 . Agriculture Handbook Number, 66.
- Langlet O. 1963. Patterns and terms of intra-specific ecological variability. Nature. 200(4904):347–348. doi: 10.1038/200347a0
- Leivsson TG. 1987. Growing Christmas trees and decoration greenery in Norway. Some non-native tree species favorable for this purpose. In Norwegian with English summary. Rapport fra Norsk institutt for skogforskning. Skogforsk. 7(87):1–7.
- MacDonald MT, Lada RR. 2008. Cold acclimation can benefit only the clones with poor needle retention duration (NAD) in balsam fir. HortScience. 43:1273.
- MacDonald MT, Lada RR. 2018. Seasonal changes in soil and tissue nutrition in balsam fir and influence on postharvest needle abscission. Scand J For Res. 33(5):426–436. doi: 10.1080/02827581.2018.1427788
- MacDonald MT, Lada RR, Dorais M, Pepin S. 2012. Influence of humidity and temperature on postharvest needle abscission in balsam fir in the presence and absence of exogenous ethylene. HortScience. 47(9):1328–1332. doi: 10.21273/HORTSCI.47.9.1328
- MacDonald MT, Lada RR, Veitch RS, Thiagarajan A, Adams AD. 2014. Postharvest needle abscission resistance of balsam fir (Abies balsamea) is modified by harvest date. Can J For Res. 44(11):1394–1401. doi: 10.1139/cjfr-2014-0199
- Mitcham-Butler EJ, Eric Hinesley L, Pharr DM. 1988. Effects of harvest date, storage temperature, and moisture status on postharvest needle retention of Fraser fir. J Environ Hortic. 6(1):1–4. doi: 10.24266/0738-2898-6.1.1
- Nielsen U, Hansen JK, Kromann HK. 2011. Impact of site and provenance on economic return in Nordmann fir Christmas tree production. Scand J For Res. 26(2):74–89. doi: 10.1080/02827581.2010.526955
- Nielsen UB, Chastagner GA. 2005a. Genetic variation in postharvest needle retention among Nordmann fir families and grafted clones. Scand J For Res. 20(4):304–312. doi: 10.1080/02827580510008365
- Nielsen UB, Chastagner GA. 2005b. Variation in postharvest quality among Nordmann fir provenances. HortScience. 40(3):553–557. doi: 10.21273/HORTSCI.40.3.553
- Nielsen UB, Xu J, Nielsen KN, Talgø V, Hansen OK, Thomsen IM. 2017. Species variation in susceptibility to the fungus Neonectria neomacrospora in the genus Abies. Scand J For Res. 32(5):421–431. doi: 10.1080/02827581.2017.1287300
- Norwegian Meteorological Institute. 2020. eKlima. [accessed 2020 Jan]. http://eklima.met.no/.
- Nyeggen H, Skage J-O. 2001. Juletrekvalitetar etter kontrollerte krysningar med gran frå Huse og Møystad frøplantasjar. Rapport fra Skogforskningen. 6(1):1–15.
- Nyeggen H, Skage J-O. 2002. Juletrekvalitetar etter open pollinering i granskog på Austlandet. Rapport fra Skogforskningen. 3(2):1–12.
- Nyeggen H, Skage J-O. 2005. Juletrekvalitetar etter kontrollerte krysningar med gran frå Drogseth og Romedal frøplantasjar. Rapport fra Skogforskningen. 2(5):1–12.
- Pettersson M, Frampton J, Rönnberg J, Brurberg MB, Talgø V. 2019. Presence of Phytophthora species in Swedish Christmas tree plantations. Eur J Plant Pathol. 153(4):1221–1236. doi: 10.1007/s10658-018-01638-2
- Sakai A, Larcher W, editors. 1987. Frost survival of plants. Responses and adaptation to freezing stress. Ecological studies. Berlin: Springer-Verlag.
- Skúlason B, Hansen OK, Nielsen UB. 2018. Provenance variation in Christmas tree characteristics in subalpine fir (Abies lasiocarpa) planted in Denmark and Iceland. Scand J For Res. 33(3):222–232. doi: 10.1080/02827581.2017.1348538
- Skúlason B, Hansen OK, Thomsen IM, Talgø V, Nielsen UB. 2017. Damage by Neonectria neomacrospora and Adelges piceae in provenance trials of subalpine fir (Abies lasiocarpa) in Denmark. For Pathol. 47(3):e12326. doi: 10.1111/efp.12326
- Strande J-A. 2015. Producing Christmas trees in “the land of the Midnight sun”. In: Talgø V, Fløistad IS, editors. The 12th International Christmas Tree Research and Extension Conference; 2015 Sept 6–11; Honne. NIBIO Book. Vol. 1(1).p. 16.
- Talgø V, Herrero M, Toppe B, Klemsdal S, Stensvand A. 2006. First report of root rot and stem canker caused by Phytophthora cambivora on noble fir (Abies procera) for bough production in Norway. Plant Dis. 90(5):682–682. doi: 10.1094/PD-90-0682B
- Talgø V, Herrero ML, Toppe B, Klemsdal SS, Stensvand A. 2007. Phytophthora root rot and stem canker found on Nordmann and subalpine fir in Norwegian Christmas tree plantations. Plant Health Prog. 8(1):29. doi: 10.1094/PHP-2007-0119-01-RS
- Thiagarajan A, Lada R, Pepin S, Forney C, Desjardins Y, Dorais M. 2012. Characterization of phytohormonal and postharvest senescence responses of balsam fir (Abies balsamea (L.) Mill.) exposed to short-term low temperature. Trees. 26(5):1545–1553. doi: 10.1007/s00468-012-0728-1
- Weiser CJ. 1970. Cold resistance and injury in woody plants: knowledge of hardy plant adaptations to freezing stress may help us to reduce winter damage. Science. 169(3952):1269–1278. doi: 10.1126/science.169.3952.1269
- Wilmot N, Morel P, Huche-Thelier L, Houis V, Joussemet M-A. 2017. Characterization of postharvest quality of two French Christmas trees: Nordmann fir (Abies nordmanniana) and Norway spruce (Picea abies). In: Talgø V, Fløistad IS, editors. Proceedings from the 12th International Christmas Tree Research and Extension Conference; 2015 Sept 6–11; Honne. NIBIO Book. Vol. 3(6). p. 84–85.