ABSTRACT
Cavity nesting birds depend on the availability of tree cavities for breeding, but the structure of the surrounding environment may also impact cavity occupancy. Here we investigated the effects of forest structure on occupancy rates of excavated cavities and similar-size nest boxes by cavity nesters in managed southern boreal forests in Finland. We recorded the occupancy over five breeding seasons (2017–2021) and derived forest structure parameters from high-resolution thematic raster maps. We found a high occupancy rate in nest boxes (81%), but lower rates in natural cavities excavated in forest interior, forest edges and retention trees (42-46%). The analyzes focusing on Paridae only revealed that the occupancy rates in nest boxes were decreasing with increasing amounts of Norway spruce and deciduous tree foliage biomass, and tree height, but increasing with the proportion of Scots pine. In forest interior cavities, we found a negative effect of stand age but a positive effect of the proportion of spruce. We conclude that the benefits of nest boxes can be maximized by considering specific forest parameters when deploying them. In excavated cavities the occupancy rates are likely more dependent on the varying internal quality than on the forest structure.
Introduction
Boreal forests represent a biome providing a relative abundance of cavities (Andersson et al. Citation2018; Baroni et al. Citation2020), while still holding one of the lowest cavity densities in the world (Boyle et al. Citation2008; Remm and Lõhmus Citation2011; Andersson et al. Citation2018). Cavities are excavated by primary cavity nesters, mostly woodpeckers (Cockle et al. Citation2011; Ouellet-Lapointe et al. Citation2012; Andersson et al. Citation2018; Pakkala et al. Citation2018a). They choose tree candidates for excavation mainly based on the trunk diameter of a tree (Gutzat and Dormann Citation2018; Basile et al. Citation2020), the stage of its wood decay (Pasinelli Citation2007; Zahner et al. Citation2012; Pakkala et al. Citation2020), and they can prefer certain tree species (Volke et al. Citation2010; Hebda et al. Citation2017; Pakkala et al. Citation2020). In managed boreal forests, the availability of suitable trees has been heavily impacted by broadly applied intensive forest management (Kouki et al. Citation2001; Andersson et al. Citation2018; Määttänen et al. Citation2022). Apart from natural processes like the falling of cavity-bearing trees (Hardenbol et al. Citation2019), frequent logging and clear-cutting are important determinants of cavity availability and distribution in managed forests (Andersson et al. Citation2018). These management practices negatively impact the density of cavity-breeders (Virkkala Citation2004) and also alter the surrounding environment around the existing cavity-bearing trees during the lifetime of the cavities. Under favorable conditions, they can provide breeding opportunities even for decades (Wesołowski Citation2011; Edworthy and Martin Citation2013; Pakkala et al. Citation2018b), depending on the tree decay stage (Edworthy et al. Citation2012) and tree species (Wesołowski Citation2011). The most noticeable change in this environment occurs when cavity-bearing trees, originally located in the forest interior, are left as retention trees in a clear-cut area or at the edge of a forest stand. The cavities in such located trees can be occupied at different rates or by different species compared to forest interior cavities (Carlson Citation1994).
Secondary cavity nesters (SCN) rely on previously excavated cavities (Martin and Eadie Citation1999; Martin et al. Citation2004), and some of them also assess the suitability of the surrounding breeding environment (Goodenough et al. Citation2009). One of the parameters they consider could be the habitat structure (Mänd et al. Citation2005). If the forest structure around a cavity changes significantly due to forest management interventions and no other cavities are available nearby, SCN can be driven to breed in potentially non-optimal environments, including very young forest stands, clear-cut areas, or forest edges (Carlson Citation1994). Furthermore, SCN could prefer forest stands with a relatively higher share of deciduous trees for breeding. In these stands, SCN could find an abundance of Lepidoptera larvae, the preferred prey for some of them during the breeding season (Rytkönen and Orell Citation2001; Mägi et al. Citation2009; Rytkönen et al. Citation2019). Because birds often search for them in deciduous tree canopies (Rytkönen and Krams Citation2003), we assume small SCN could prefer stands with a higher share of these trees in otherwise conifer-dominated boreal forests.
An aggregated distribution of the excavated cavities (Remm et al. Citation2006) and their possible shortage in forests structurally suitable for breeding of SCN can be compensated by deploying nest boxes in forest stands. Unlike woodpecker-excavated holes, these artificial cavities bring several advantages to cavity occupancy research. They are easily accessible for checking their content, they have known locations in the field, their quality can be adjusted in accordance with the research requirements, and, most importantly, all these parameters can be easily controlled for a specific experiment. There are, however, not many studies that have examined how the occupancy of nest boxes compares to that of natural cavities (e.g. McComb and Noble Citation1981; Brawn Citation1988; Lundberg and Alatalo Citation2010; Schwartz et al. Citation2020; Sudyka et al. Citation2022), and we are not aware of such studies in southern boreal forests.
Collecting detailed forest structure data through a field survey can be costly and highly time-consuming, especially for large study areas. For these reasons, high-resolution remotely sensed data on various forest parameters could represent environmental data sets suitable for predicting cavity occupancy. Indeed, satellite-derived data have been successfully used for similar purposes, such as deriving bird-habitat preferences (Fuller et al. Citation2005; Rönkä et al. Citation2008), predicting habitat-related spatial variation in bird abundance (Rhodes et al. Citation2015) or predicting bird occurrence in valuable forest habitats (Virkkala et al. Citation2022). In general, we assume that forest structure data depicting the main forest management interventions (e.g. areas of clear-cutting) and basic structural parameters (e.g. tree canopy coverage and tree species proportion) could represent relevant predictors for tree cavity occupancy (Amininasab et al. Citation2016; Potti et al. Citation2018; Ceia et al. Citation2023).
Here we investigated the effects of the forest structure of southern boreal forests in Finland on the occupancy rates in two cavity types – tree excavated cavities and nest boxes – occupied by SCN. We also performed separate analyzes on combined great tit Parus major and Eurasian blue tit Cyanistes caeruleus cavity occupancy, as these species are widespread breeders in the studied region (Keller et al. Citation2020) and, at the same time, we have limited information on their breeding in natural cavities or large forest areas. We assumed that cavity occupancy is affected by the specific structure of the forest stands surrounding a cavity, as birds generally select specific vegetation structures in their habitats (MacArthur and MacArthur Citation1961; Cody Citation1981). We investigated the effects of forest structure parameters commonly used in forest research (Hui et al. Citation2019), including tree dimensions (e.g. height, diameter or volume), tree species proportions, canopy coverage, foliage biomass, and stand age. Additionally, we distinguished whether a cavity-bearing tree is located in the forest interior, edge, or as a retention tree in a clear-cut. This was done to account for different cavity locations in the forest stands. Our analyzes should elucidate whether these basic forest structural parameters could be considered relevant predictors for the occupancy rates of tree cavities. More specifically, we addressed the following questions: i) What are the occupancy rates in the different cavity types? We predicted that nest boxes would be occupied at higher rates than excavated cavities as observed in various bird species (Brawn Citation1988; Schwartz et al. Citation2020; Baroni et al. Citation2023). ii) Which of the considered forest parameters influence the occupancy rates of these cavity types? We predicted that an increase in all measured forest structure parameters and stand age would positively affect the occupancy rates of cavity types, as higher values of these parameters should be associated with older or more complex forests, which are potentially preferred for breeding. iii) Does the share of deciduous tree species in forest stands increase the occupancy rates? We predicted that an increasing share of deciduous tree species could have a positive effect on occupancy rates in otherwise conifer-dominated forest habitats due to the potentially increased food offer as outlined above. iv) Do the main gradients (as principal components of different traits) in forest structure predict the occupancy rates of cavity types? We tested the possibility that gradients of forest structure derived from the basic forest parameters may serve as suitable predictors of occupancy rates.
Methods
Study area
The study area was located in southern boreal forests in Finland (bounding box 60.48° N, 22.00° E and 60.80° N, 22.45° E), approximately between the city of Turku and the municipalities of Mynämäki, Yläne and Aura, covering ca 335 km2 of forests (). The forests of Southwest Finland, where our study area was located, comprise primarily of Scots pine Pinus sylvestris and Norway spruce Picea abies, deciduous trees are represented mainly by silver and downy birch Betula pendula, B. pubescens and European aspen Populus tremula (Peltola et al. Citation2020). The overwhelming majority of the forests in the study area are intensively managed for wood production. Only a small proportion of the forest area (3%) enjoys protection status (SYKE Citation2021), and these forests are not logged.
Figure 1. Positions of cavity-bearing trees ♦ (n = 215) and nest boxes ● (n = 236) in the study area located in Southwest Finland. The numbers on the symbols denote the aggregated counts of respective cavity types. The background map depicts the main land cover classes which were derived from a modified Corine Land Cover 2018 raster (SYKE Citation2021).
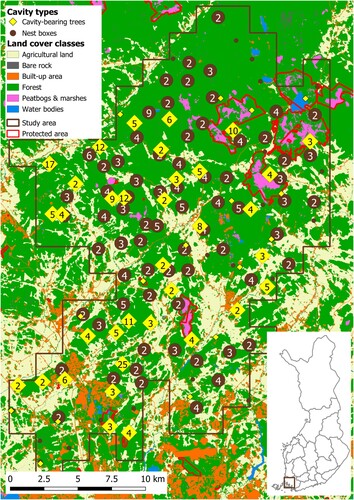
Bird species and cavity types
Tree cavities in the study area are excavated by several primary cavity nesters. A great majority is excavated by great-spotted woodpecker Dendrocopos major (GSW), and a smaller proportion of similar-size cavities by grey-headed woodpecker Picus canus, which we also considered and pooled with GSW cavities. We originally performed a complete census of all GSW-sized excavated cavities in 50 four-hectare grids that were randomly placed in the study area (Baroni et al. Citation2020). In these grids, all potential trees were examined for the existence of cavities (from the ground) in a survey that could take one to four hours depending on the site (Baroni et al. Citation2020). Cavities that were found opportunistically outside the grids during this survey and when moving in this area, e.g. during monitoring of nest boxes (see below) were added to the database and examined in later years. These cavities are a bit different from grid searches, as finding them depends on the routes taken. Especially aspen trees with cavities can be found from a long distance when they are left in clear-cuts in groups or as single retention trees, and at the edges of forest stands. This is not however a problem in the context of this study, as we are not attempting to estimate and extrapolate the number of cavities or calculated their densities in different habitats, but only to examine their occupancy rates in relation to forest structure around them. We used a pole-mounted camera (Wildlife Windows Ltd) to inspect cavities for occupancy from May to June 2018–2021. The use of the camera allowed us to exclude obviously unsuitable cavities for breeding, such as those filled with debris, flooded, or unfinished cavities without a breeding chamber. Each cavity was checked at least once during this period, but some cavities were checked yearly. The timing was chosen so that in addition to resident birds, the cavities could already be occupied also by long-distance migrant species (pied flycatcher Ficedula hypoleuca and common redstart Phoenicurus phoenicurus).
In the accessible GSW cavities (mean ± SD height = 5.5 ± 2.0 m), excavated lower than 13 m, we recognized bird occupancy as the presence of an incubating female, a nest with eggs, and also when we found an incomplete nest, i.e. fresh nesting material was present. Nests of different species are typically easy to identify even in the absence of a parent bird, owing to the species-specific nesting material they use. Cavities containing nests built apparently in previous breeding seasons were considered unoccupied. For subsequent analyzes, we recognized the occupancy per cavity-bearing trees (tree-wise approach), not allowing for the number of occupied cavities in multi-cavity trees, to at least partly take into account the effect of bird territoriality. Cavity-bearing trees with at least one cavity occupied by GSW or pygmy owl Glaucidium passerinum in the focal year were excluded from the data set as unsuitable for the breeding of great tits and blue tits when focusing the analyzes on them.
In the same study area, we placed nest boxes mainly during 2012–2013 and checked their content each year between April and June; here the data from 2017–2020 is used for comparison with the natural cavity study conducted in these years. The placement of nest boxes was done so that there would be no more than one nest box in a square kilometer grid (in the Finnish grid coordinate system) and thus the distance between two nest boxes would be approximately one kilometer or more. Nest boxes were designed to resemble GSW cavities with their dimensions: inner diameter = 15-17 cm, entrance to bottom distance = 30-35 cm, and entrance diameter = 4.5 cm. Before their installation, we poured coarse wooden sawdust on the bottom of each nest box to better reflect a cavity previously occupied by woodpeckers or other species. We attached nest boxes to tree trunks at a height of ca 1.5 m allowing easy checking of their content. We evaluated the occupancy of nest boxes using the same criteria as in excavated cavities.
In total, we collected data on occupancy in 215 cavity-bearing trees (in each year of 2018–2021 we checked 110, 88, 90, and 103 of them, respectively) and 236 nest boxes (in each year of 2017–2020 we checked 192, 210, 134, and 201 of them, respectively). For cavity-bearing trees, we distinguished the following groups based on the location of trees in a forest: retention trees (a tree or group of trees left in a clear-cutting area, n = 42), forest edge (cavity-bearing trees found < 25 m from the stand edge; forest roads were not considered as forming the edges if the forest stands were present on both sides of a road, n = 75), and forest interior (cavity-bearing tree > 25 m from the stand edge, n = 103). Cavity-bearing trees included aspen (n = 158), other deciduous trees (n = 15), Scots pine (n = 26), spruce (n = 6), and undetermined tree species (n = 10). The locations of excavated cavities were more clustered than in nest boxes (), but still more than 2/3 of excavated cavities and nest boxes were located at least 50 m apart, and approximately 90% of excavated cavities were found at least 50 m apart from the nearest nest box.
Forest structure
To describe the forest structure around cavity-bearing trees and nest boxes, we used Multi-source National Forest Inventory (MS-NFI) thematic raster maps of 2017 and 2019 (Mäkisara et al. Citation2019) provided by Natural Resources Institute Finland (Luke). These high-resolution data (16 m × 16 m per pixel) are based on field plot measurement and satellite image data. For our occupancy analyzes, we calculated the means of respective forest parameters for each year in buffers of 25 and 50 m radii around the excavated cavities and nest boxes, to cover both the immediate environment around a cavity and an area approximately corresponding to the breeding territory size of the studied great and blue tits (Krebs Citation1971; Dhondt et al. Citation1982; Sasvari Citation1991). We considered the following forest parameters: mean amount of spruce, pine, and deciduous tree foliage biomass (t.ha−1), mean stand tree height (m), mean stand tree diameter (cm), mean canopy coverage (%), mean growing stock volume of spruce, pine, and deciduous trees (m3.ha−1), mean stand basal area (m2.ha−1), and mean canopy coverage of deciduous trees (%), for which we show mean values and ranges in the buffers of 25 m () and 50 m (Table S1). For years not covered by the MS-NFI product (i.e. 2018, 2020, 2021), we created a vector layer with manually drawn polygons depicting new clear-cut areas and mineral extraction sites, representing key changes in forests, using QGIS 3.20 (QGIS.org Citation2021). We overlapped the forest parameter raster layers with the polygons and set the values of raster layer pixels to zero in clear-cut areas and to NA in extraction sites. The positions of clear-cut and extraction areas were determined from Sentinel-2A high-resolution (10 m per pixel) satellite images (Drusch et al. Citation2012) taken in months June to August in the respective years. We also derived the main gradients of forest structure by means of principal component analysis (PCA, for details see below), as principal components (PCs). Lastly, we obtained the age of forest stands in the buffers from Suomen metsäkeskus (The Finnish Forest Center) spatial data sets, available at https://aineistot.metsaan.fi/avoinmetsatieto/Metsavarakuviot/Karttalehti/
Table 1. Mean ± SE and range (min – max) of values of forest structure parameters in 25 m buffer around the cavity-bearing trees (retention trees n = 42, forest edge n = 75, forest interior n = 103) and nest boxes (n = 236). Pine_fol = mean pine foliage biomass (t.ha−1), spruce_fol = mean spruce foliage biomass (t.ha−1), dec_fol = mean deciduous trees foliage biomass (t.ha−1), height = mean stand tree height (m), diam = mean stand tree diameter (cm), canopy = mean canopy coverage (%), canopy_dec = mean canopy coverage of deciduous trees (%), pine_vol = mean growing stock volume of pine (m3.ha−1), spruce_vol = mean growing stock volume of spruce (m3.ha−1), dec_vol = mean growing stock volume of deciduous trees (m3.ha−1), basal = mean stand basal area (m2.ha−1), age = stand age (years).
Data analyzes
We calculated the probabilities of occupancy for the cavity types (excavated cavities in retention trees, at forest edges, in the forest interior, and nest boxes) in all species and separately for the combination of great and blue tits, and investigated the effect of forest structure on the occupancy of these tit species only, due to their distinctively different habitat requirements among other cavity nesters. Tit species were combined because it is difficult to separate failed nests with certainty, but a great majority of them were great tits (see below).
To obtain the main gradients in forest structure and test their effects on occupancy rates, we performed PCA on all forest structure parameters, using the built-in R function “prcomp”. We further used four PC components, where PC1 can be described as a gradient from sparse forests and clear-cutting areas to grown pine and spruce forests, PC2 as a gradient from deciduous tree dominated to pine-dominated forests, PC3 as a gradient from pine and deciduous tree forests to spruce forests, and PC4 as a gradient from young to old forests (). The gradient interpretation remains the same across the years of the study (Fig. S2, Table S3).
Figure 2. Ordination plots depicting the relationships between the forest parameters along PC1, PC2, PC3 and PC4 gradients of forest structure in 2017. Abbreviations: Pine_fol = mean amount of pine foliage biomass, spruce_fol = mean amount of spruce foliage biomass, dec_fol = mean amount of deciduous tree foliage biomass, height = mean stand tree height, diam = mean stand tree diameter, canopy = mean canopy coverage, canopy_dec = mean canopy coverage of deciduous trees, pine_vol = mean growing stock volume of pine, spruce_vol = mean growing stock volume of spruce, dec_vol = mean growing stock volume of deciduous trees, basal = mean stand basal area, age = mean forest stand age. For ordination plots across all the years see Fig. S2.
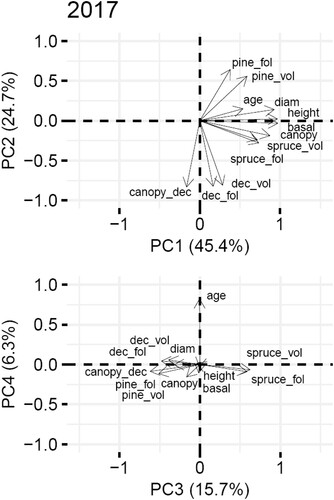
Before building any models, we examined the correlations and variance inflation factors (VIF) of the predictors in the R-package “usdm” (Naimi et al. Citation2014) to minimize multicollinearity between the predictors (VIF < 5, Supplementary Material, Fig. S1, Table S2). Based on this procedure, which indicated high multicollinearity between certain forest structure predictors, we minimized the subset of selected forest structure parameters by excluding canopy coverage, canopy coverage of deciduous trees, basal area, tree diameter, and growing stock volume of tree species, as they were highly correlated (|r| > 0.7) with some of the predictors that were retained in the models, namely tree height and amount of tree foliage biomass. We kept these last mentioned but correlated forest structure variables in separate models because they may be relevant for describing forest birds’ breeding territories, and excluding them would leave their effects on occupancy rates unknown. We built six models to test our hypotheses: The first model shows occupancy rates in both cavity types regardless of the effects of any forest structure variables (Question 1). This model was also fitted for all species combined, unlike the other models that were fitted only for the combination of blue and great tits. The second model shows solely the effect of stand age on occupancy rates in cavity types. The third model reveals the effect of tree height while controlling for stand age. The fourth model shows the effects of the amount of different tree species’ foliage biomass on occupancy rates, controlled for stand age. These models (2, 3 and 4) were built to answer Question 2. The fifth model explores whether the effects of the amount of coniferous tree foliage biomass on occupancy rates of cavity types change with the amount of deciduous tree foliage biomass in the stands, controlled for stand age (Question 3). The last model investigates the effects of the main forest gradients, described by principal components (PCs), on occupancy rates (Question 4). These models were fitted as logistic mixed-effect models with a binomial error structure and a logit link function using the R-package “glmmTMB” (Brooks et al. Citation2017). We defined cavity occupancy (1 = occupied, 0 = unoccupied) as the response variable, cavity type × forest structure or cavity type × PCs interactions were the predictors, age of forest stands was a confounding variable (except for the first and the last model), and an exponential covariance structure was included as a spatial autocorrelation term. The year of cavity checking was not included as a random effect (RE) in any model because the RE models did not perform better than non-RE models (ΔAICc < 2; Table S2a,b). We did not consider stand age in models including PC components since it was already included as a predictor in the PCA analysis (see mentioned above). To investigate possible nonlinear effects of the predictors, we compared models including linear terms to models including polynomial terms using AICc. Only the model including the polynomial term of mean stand tree height outperformed the simpler model (Table S2c, d). We present the output of this model separately (Table S5b, Fig. S3) from a set of six final models that include linear terms only ().
Table 2. The final models relating the occupancy (Occ) to parameters of forest structure around cavity-bearing trees and nest boxes (cavity type). For all species altogether we fitted model 1 only, while for the combination of great and blue tits we fitted all listed models. All models also included exponential covariance structures as spatial autocorrelation terms.
The model residuals were examined using the R-package “DHARMa” (Hartig Citation2022), and we did not find any obvious violations of the distributional assumptions. We assessed model fits by expressing the total variance explained by the models (Nakagawa's R2 for spatial models and Tjur's R2 for non-spatial models) using “performance” package (Lüdecke et al. Citation2021).
Marginal means for factors and linear relationships of the forest parameters were obtained using functions from the R-package “emmeans” (Russell Citation2022), which allowed for a correction for multiple comparison tests by assuming multivariate t-distributions. All analyzes were conducted in R 4.1.2 (R Core Team Citation2022).
Results
We recorded breeding of one primary cavity nester, the great-spotted woodpecker, and eight SCN ().
Table 3. Species breeding in the excavated cavities (cavity-bearing trees, n = 215) and nest boxes (n = 236) in the study area, the number of nests found, and mean species’ occupancy rates in the cavity types. Parus = undetermined, either great or blue tit nest.
A model on occupancy by any species across years showed different occupancy rates among the cavity types (χ2 = 110.5, df = 3, p < 0.0001). Specifically, the occupancy rates were 46% (95% CI: 32.8-59.2%) in retention trees, 43% (33.6-52.8%) in forest edges, 42% (33.7-51.3%) in the forest interior, and nearly twice as high at 81% (77.5-84.3%) in the nest boxes (a). From here on, we only focused on the nests of great and blue tits, since from these species we had sufficient data for examining the effects of forest structure on occupancy (93 and 548 nests in excavated cavities and nest boxes, respectively). Including other SCN (20 and 44 nests in excavated cavities and nest boxes, respectively) with substantially different ecologies and even a non-bird species (Siberian flying squirrel) could only confound the results.
Figure 3. The probability of occupancy of cavity-bearing trees recognized as retention trees (n = 42), forest edge trees (n = 75), and forest interior trees (n = 103) and of nest boxes (n = 236) being occupied by (a) any cavity nesters and (b) by great and blue tits in managed boreal forests of SW Finland in 2017-2021. Error bars depict 95% CIs.
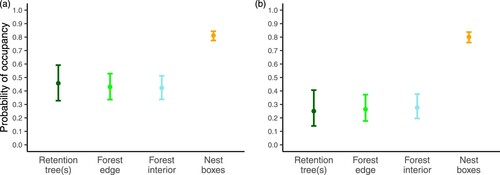
We found an immense difference in great and blue tit occupancy between the cavity types (χ2 = 135.4, df = 3, p < 0.0001, b). The cavities in retention trees, forest edges, and the forest interior were occupied at a low rate of 25% (95% CI: 14.0-40.6%), 26% (17.7-37.2%), and 28% (19.5-37.7%), respectively, while nest boxes were used three times more frequently for breeding, at an occupancy rate of 80% (76.0-83.7%). The age of the forest stand did not have any obvious effect on occupancy rates in the cavity types (), although there was a tendency (p = 0.057) of lower occupancy rates in nest boxes placed in older forests when considering the 50 m buffer (b).
Table 4. Conditional effects of forest structure parameters on the occupancy of cavity-bearing trees (recognizing cavities in retention trees, forest edge, and forest interior) and nest boxes in buffers of (a) 25 m and (b) 50 m around the respective cavity types. Model numbers correspond with the numbering in . For full model outputs see Table S5a. Statistically significant effects (p < 0.05) are in bold.
Most of the statistically significant effects of forest structure on occupancy rates became apparent for nest boxes, and only a few effects appeared in cavity-bearing trees. Increasing tree height was related to a decrease in occupancy of nest boxes in both buffers (). In numbers, the occupancy rates decreased from 91 to 72% and from 93 to 70% with increasing tree height for the 25 and 50 m buffers, respectively (a). The effect of the amount of tree foliage biomass was connected with decreasing occupancy rates with increasing spruce foliage biomass for the 25 m buffer (from 87 to 65%, b) and with increasing deciduous tree foliage biomass for the 50 m buffer (from 86 to 74%, c), both for nest boxes. For foliage biomass, only in pine we found a tendency (p = 0.072) for a positive effect, increasing the occupancy rates for the 50 m buffer in nest boxes (). However, this latter mentioned non-significant effect gained support from an increasing occupancy of nest boxes with the increasing PC2 component (), describing a gradient from deciduous tree dominated to pine-dominated forests, from 66 to 89% and from 67 to 90% for the 25 and 50 m buffers, respectively (, d). An indication of a negative effect of the PC4 component on nest box occupancy for the 25 m buffer (p = 0.066), describing a gradient from young to old stands (), is in line with the forementioned indication of a negative effect of stand age on nest box occupancy rates.
Figure 4. Statistically significant relationships between the probability of occupancy of the nest boxes and cavity-bearing trees and forest structure parameters estimated within 25 and 50 m buffers: (a) tree height, (b) amount of spruce foliage biomass, (c) amount of deciduous tree foliage biomass, (d) forest structure determined by PC2 (a gradient from deciduous tree dominated to pine-dominated stands), (e) forest structure determined by PC3 (a gradient from non-spruce to spruce stands), and (f) forest structure determined by PC4 (a gradient from young to old stands). Histograms show the counts of nest boxes and cavity-bearing trees at specific values of respective forest structure parameters.
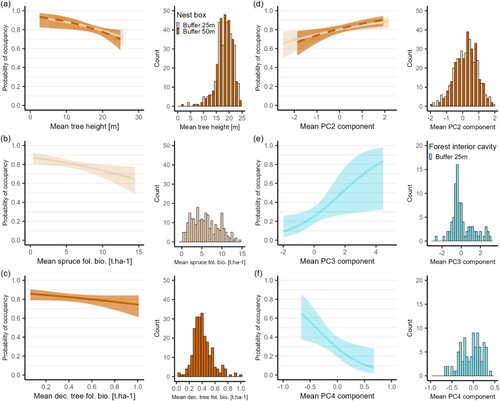
In cavity-bearing trees, we found an increase in forest-interior cavity occupancy with the PC3 component, describing a gradient from non-spruce to spruce stands (), and a decrease in occupancy rates with the PC4 component, describing a gradient from young to old forest stands (), both in the 25 m buffer (a, e,f). In the same cavity type, the increasing amount of pine foliage biomass in the 25 m buffer was associated with a marginally non-significant (p = 0.053) increase in occupancy rates (a).
Significant interaction effects of the amount of deciduous tree foliage biomass were shown only in cavity-bearing trees at forest edges. Contrary to our expectations, these effects were negative, indicating that with an increasing amount of pine foliage biomass the occupancy rates were decreasing more the larger was the amount of deciduous tree foliage biomass in both buffers (statistically significant interaction pine foliage biomass × deciduous trees foliage biomass, b).
The total variance explained by the model for all species was 17.3% and models for great and blue tits explained between 27.5 and 35.1% of the total variability. The models that did not account for spatial autocorrelation explained ∼ 5% less variance (Table S4).
Discussion
We found that cavity nesters, as well as specifically the great and blue tits, occupied cavities excavated by the great spotted woodpecker at much lower rates than nest boxes of comparable dimensions in managed southern boreal forests. Forest structure parameters describing the surrounding environment around the cavity types had some effects on occupancy rates in nest boxes and in excavated cavities that were in the forest interior and forest edges. Specifically, the models showed negative effects of tree height and the amount of spruce and deciduous tree foliage biomass, and a positive effect of the proportion of pine trees in the forest stands on the occupancy rates in nest boxes. In excavated cavities, we found a positive effect of the proportion of spruce trees in the stands, and a negative effect of stand age in the forest interior. Interestingly, on forest edges, we unexpectedly found a negative moderating effect of deciduous tree foliage biomass.
Occupancy of excavated cavities
The absence of a difference in occupancy rates between cavities in retention trees and other types of excavated cavities (forest interior, forest edge) suggests that preserving single or groups of trees in clear-cutting areas could provide similar breeding opportunities for SCN as in unlogged forest stands. Indeed, small SCN were detected in similar densities in clear-cuts with retention trees as in adjacent forest stands when sufficient numbers of trees were preserved (Söderström Citation2009). However, a low number of retention trees can result in less frequent breeding in clear-cuts compared to forest edges (Carlson Citation1994; Söderström Citation2009).
The pattern of low tree cavity occupancy we present here has been shown several times in boreal or higher altitude forests: in the primeval boreal forests in Mongolia (Bai et al. Citation2003), mid-mountain deciduous forests in Catalonia (Camprodon et al. Citation2008), boreal deciduous forests in central-south Sweden (Carlson et al. Citation1998), sub-boreal mixed forests of Canada (Edworthy et al. Citation2018), and in conifer-dominated southern boreal forests in Finland (Pakkala et al., Citation2018b, this study). The explanation for this pattern may lie in the varying internal quality of the cavities (Wiebe et al. Citation2020), assuming birds could preferentially select less degraded cavities (Aitken and Martin Citation2008). Ideally, we should exclude cavities considered by SCN as too poor in quality for breeding from the analyzes, not just cavities obviously unsuitable for breeding (see Methods). Then we could expect to obtain higher occupancy rates, which would, however, better reflect the real supply and demand in the tree cavity system. The low occupancy rates in tree cavities could also result from the fact that only cavities with ideal dimensions and of very good quality may be used for breeding, or SCN may have to compete with primary cavity nesters for superior cavities as they can reuse them (Pakkala et al. Citation2017; Koenig et al. Citation2021).
Apart from an analysis on all cavity nesters, we also analyzed the occupancy of cavities by great and blue tits together, although they can choose slightly different cavities for breeding. When breeding in excavated cavities, the great tit can prefer the larger ones with larger entrances compared to the blue tit (Carlson et al. Citation1998; Remm et al. Citation2006), which frequently breeds also in non-excavated tree holes (Wesołowski and Rowiński Citation2012). Therefore, if the internal quality of a cavity plays a role in their breeding cavity choice, it could be dominantly driven by the great tit's preference for larger GSW cavities. We pooled these two species in the analyzes because it is not always possible to distinguish between them when inspecting natural cavities, but it is important to note that the number of great tits in our data is clearly higher than that of blue tits ().
The excavated cavities had a much more clustered distribution compared to the intentionally spaced nest boxes (). This aggregation could affect the occupancy rates on closely neighboring cavities via intra – or interspecific competition, regardless of the forest structure, as observed in nest boxes installed close to each other and occupied by blue tits (Serrano-Davies et al. Citation2017). Although greater proximity between cavities is a natural phenomenon (Remm et al. Citation2006), we at least tried to cope with this effect on occupancy rate in the most complicated cases of multi-cavity trees, by employing a tree-wise approach. We assume this approach was meaningful since the great tit, accounting for the majority of our occupancy events, practically does not utilize multiple breeding opportunities in the same tree (Dolenec Citation2019).
Many of the suitable cavities can be located in suboptimal breeding environments, such as areas where food sources are scarce or a chance of predator visits is more likely (Sandström Citation1991). If such environments are avoided for breeding, even a high-quality cavity located in them may be occupied less frequently, as indirectly suggested by lower occupancy rates found in nest boxes placed in non-preferred habitats (Mänd et al. Citation2005). Conversely, we can describe the environment as very suitable for breeding, but the available cavities are of poor quality and remain unoccupied. However, the latter scenario is less likely since we inspected the cavities with a camera and excluded those that were very likely unsuitable for breeding.
Occupancy of nest boxes
The decline in the occupancy rate in nest boxes surrounded by forests with high amounts of spruce foliage biomass can be partly related to a generally lower preference for coniferous stands for breeding (Lemel Citation1989; Mägi et al. Citation2009; Mänd et al. Citation2009) or foraging (Suhonen et al. Citation1994; Rytkönen and Krams Citation2003) by great and blue tits in mid – and higher latitudes. However, since birds cannot avoid breeding in coniferous stands dominating in the study area, they might at least not prefer dense coniferous forests. Indeed, the positive relationship in occupancy rates with pine-dominated stands (d) indicates that habitat avoidance probably concerns only much denser spruce but not pine forests (see also for the amount of foliage biomass). We suggest that the understory layer can be poor in vegetation in a cultivated dense forest that has not yet undergone natural thinning or disturbances creating openings. The detection of a significant negative effect of spruce foliage biomass in nest boxes only in the smaller 25 m buffer suggests that SCN may be more sensitive to specific forest structure immediately around the nest than at the edge of their breeding territories. Alternatively, nest boxes may be less frequently occupied in grown spruce forests because in such stands the excavated cavities could be abundant and thus not all cavities (whether nest boxes or tree cavities) can be occupied. The negative effect of tree height on occupancy rates is not surprising when we have a look at the close correlation between this parameter and spruce forest variables, i.e. the amount of foliage biomass and growing stock volume (). Spruce forests were simply also tall forests.
As in the excavated cavities, the occupancy rates in nest boxes could result from their specific attributes. Their relatively little decomposed walls or generally lower humidity inside them (Maziarz et al. Citation2017) could make them of superior quality compared to tree cavities. But despite the high occupancy rate, the role of nest boxes in promoting great and blue tit populations in our study area is not conclusive based only on the presented results. We would need to collect complete information on nesting success or nestling survival rates in both cavity types to fully assess the benefits of nest boxes. They may represent high-quality cavities for building nests, which was reflected in their high occupancy rate. But they might also be occupied frequently because great tits can widely accept nest boxes located in less preferred environments (Mänd et al. Citation2009).
Moderation effect of deciduous foliage
We did not detect the expected positive effect of a higher share of deciduous trees around the cavity types, which could be assumed to provide a higher abundance of Lepidoptera larvae, the preferred food source of the studied great and blue tits (Rytkönen and Orell Citation2001). Instead, we found a negative effect of this parameter in nest boxes, as well as a negative effect of deciduous tree foliage in the interaction with pine foliage biomass in forest edge cavities (). These effects might come from a low preference of great and blue tits for breeding in or near young forest stands formed by birches, or close to retention trees preserved in clear-cut areas (Carlson Citation1994). Also, they could avoid breeding in deciduous trees at forest edges, primarily composed of aspen in our study area, due to a higher risk of nest predation in such habitat (Sandström Citation1991). Finally, deciduous trees may not provide sufficient food quality compared to coniferous forests as noticed in the northern temperate region (Mägi et al. Citation2009).
Other forest and cavity parameters
The indications of significantly lower occupancy rates in nest boxes and forest interior cavities in older forests could be related to the specific structure of the stands. In the study area, the oldest forests are predominantly pine stands growing on shallow rocky soils, and pine stands at the borders of peat bogs (Finnish Forest Center spatial data). Such forests can be structurally different from old-growth forests found elsewhere in southern and middle Finland (Siitonen et al. Citation2000), and thus may not support the breeding of SCN as strongly as has been shown many times in old-growth forests (Virkkala et al. Citation1994; Rosenvald et al. Citation2011; Zawadzka et al. Citation2016; Andersson et al. Citation2018).
The height of a cavity above the ground could rank among the other parameters affecting occupancy rates. It has been shown that greater height is connected with an increased occupancy rate of nest boxes and cavities in blue tits (Serrano-Davies et al. Citation2017), but not in great tits (Dolenec Citation2019). Here the same height of 1.5 m above the ground in nest boxes did not allow to test the effect of this parameter on the occupancy rate. We can only state that the occupied excavated cavities were located higher (mean ± SD: 5.5 ± 2.0 m) than the nest boxes. We also cannot say with certainty if the high occupancy rate of low-mounted nest boxes could be related to a lower chance of nest predation because either higher (Broughton et al. Citation2011), lower (Maziarz et al. Citation2016) or no difference (Sandström Citation1991) in nest predation rates were found with increasing cavity height.
The suitability of tree cavities for breeding might change as the cavities age (Edworthy et al. Citation2018; Pakkala et al. Citation2018b). Unfortunately, since we do not know the age of the majority of the tree cavities we found, we could not test for this effect. Nevertheless, we believe that our annual assessment of each cavity using the pole-mounted inspection camera and the exclusion of those that were apparently unsuitable for breeding (e.g. filled with debris or flooded) helped minimize potential bias arising from variations of cavity attractiveness over time.
Conclusions
Our study shows that placing nest boxes in stands of managed boreal forests has a large potential to provide breeding opportunities for some secondary cavity nesters. Unlike excavated tree cavities, which can vary in their internal quality, cavity nesters could greatly benefit from additional nest boxes (Purcell et al. Citation1997), especially if their attributes are preserved in the long term or they are replaced when too degraded. The indication of a negative effect of forest age on both nest box and forest interior cavity occupancy points out that great and blue tits may not necessarily rely on old-growth forests as breeding habitats, or may have access to numerous tree cavities in such forests, resulting in lower occupancy rates of cavities. To sum up, excavated cavities can be occupied at low rates in managed boreal forests, with a notable effect of only some forest parameters on these rates. Providing suitable nest boxes could increase the occupancy rates of cavity nesters when the offer of suitable tree cavities is limited as in our study area (Baroni et al. Citation2020) or where SCN populations have not reached their capacity levels. When deploying nest boxes, we should consider the basic structure of the surrounding environment, such as tree species proportions, tree height or stand age, in order to maximize the breeding benefits of the nest boxes.
Supplemental Material
Download MS Word (544.2 KB)Acknowledgement
We thank all the colleagues who helped us with checking the nest boxes and excavated cavities: Jorma Nurmi, Kai Kankare, Sven Nordqvist, Jouko Högmander, Laura Hollmen, Timo Lainema, Laura Mulari, Juhani Piekkala, Ville Vasko. We thank @shuafeiwang, the author of a Google Chrome extension called editGPT, v1.0.8, which we used for English grammar checking via ChatGPT (https://chat.openai.com/).
Data availability statement
The data that support the findings of this study are available from the corresponding author, JH, upon reasonable request.
Disclosure statement
No potential conflict of interest was reported by the author(s).
Additional information
Funding
References
- Aitken KEH, Martin K. 2008. Resource selection plasticity and community responses to experimental reduction of a critical resource. Ecology. 89:971–980. doi:10.1890/07-0711.1.
- Amininasab SM, Vedder O, Schut E, de Jong B, Magrath MJ, Korsten P, Komdeur J. 2016. Influence of fine-scale habitat structure on nest-site occupancy, laying date and clutch size in blue tits Cyanistes caeruleus. Acta Oecol. 70:37–44. doi:10.1016/j.actao.2015.11.006.
- Andersson J, Domingo Gómez E, Michon S, Roberge J-M. 2018. Tree cavity densities and characteristics in managed and unmanaged Swedish boreal forest. Scand J For Res. 33:233–244. doi:10.1080/02827581.2017.1360389.
- Bai M-L, Wichmann F, Mühlenberg M. 2003. The abundance of tree holes and their utilization by hole-nesting birds in a primeval boreal forest of Mongolia. Acta Ornithologica. 38:95–102. doi:10.3161/068.038.0205.
- Baroni D, Hanzelka J, Raimondi T, Gamba M, Brommer JE, Laaksonen T. 2023. Passive acoustic survey reveals the abundance of a low-density predator and its dependency on mature forests. Landsc Ecol. doi:10.1007/s10980-023-01667-1.
- Baroni D, Korpimäki E, Selonen V, Laaksonen T. 2020. Tree cavity abundance and beyond: nesting and food storing sites of the pygmy owl in managed boreal forests. For Ecol Manag. 460:117818. doi:10.1016/j.foreco.2019.117818.
- Basile M, Asbeck T, Pacioni C, Mikusiński G, Storch I. 2020. Woodpecker cavity establishment in managed forests: relative rather than absolute tree size matters. Wildl Biol. doi:10.2981/wlb.00564.
- Boyle WA, Ganong CN, Clark DB, Hast MA. 2008. Density, distribution, and attributes of tree cavities in an old-growth tropical rain forest: short communications. Biotropica. 40:241–245. doi:10.1111/j.1744-7429.2007.00357.x.
- Brawn JD. 1988. Selectivity and ecological consequences of cavity nesters using natural vs. artificial nest sites. Auk. 105:789–791. https://academic.oup.com/auk/article/105/4/789/5192846.
- Brooks ME, Kristensen K, van Benthem KJ, Magnusson A, Berg CW, Nielsen A, Skaug HJ, Mächler M, Bolker BM. 2017. glmmTMB balances speed and flexibility Among packages for zero-inflated generalized linear mixed modeling. R J. 9:378–400. doi:10.32614/RJ-2017-066.
- Broughton RK, Hill RA, Bellamy PE, Hinsley SA. 2011. Nest-sites, breeding failure, and causes of non-breeding in a population of British marsh tits Poecile palustris. Bird Study. 58:229–237. doi:10.1080/00063657.2011.582641.
- Camprodon J, Salvanyà J, Soler-Zurita J. 2008. The abundance and suitability of tree cavities and their impact on hole-nesting bird populations in beech forests of NE Iberian Peninsula. Acta Ornithologica. 43:17–31. doi:10.3161/000164508X345293.
- Carlson A. 1994. Cavity breeding birds and clearcuts. Ornis Fenn. 71:120–122.
- Carlson A, Sandström U, Olsson K. 1998. Availability and use of natural tree holes by cavity nesting birds in a Swedish deciduous forest. Ardea. 86:109–119.
- Ceia RS, Lopes PB, Da Silva LP. 2023. Factors determining the occupancy of nest-boxes by great tits (Parus major) in eucalypt plantations. Avian Research. 14:100098. doi:10.1016/j.avrs.2023.100098.
- Cockle KL, Martin K, Wesołowski T. 2011. Woodpeckers, decay, and the future of cavity-nesting vertebrate communities worldwide. Front Ecol Environ. 9:377–382. doi:10.1890/110013.
- Cody ML. 1981. Habitat selection in birds: the roles of vegetation structure, competitors, and productivity. BioScience. 31:107–113. doi:10.2307/1308252.
- Dhondt A, Schillemans J, Laet J. 1982. Blue tit territories at different density levels. Ardea-Wageningen. 70:185–188.
- Dolenec Z. 2019. Nestbox occupancy by the great tit (Parus major L) in young deciduous forest stands. Šumar List (Online). 143:352–352. doi:10.31298/sl.143.7-8.6.
- Drusch M, Del Bello U, Carlier S, Colin O, Fernandez V, Gascon F, Hoersch B, Isola C, Laberinti P, Martimort P, Meygret A. 2012. Sentinel-2: ESA’s optical high-resolution mission for GMES operational services. Remote Sens Environ. 120:25–36. doi:10.1016/j.rse.2011.11.026.
- Edworthy AB, Martin K. 2013. Persistence of tree cavities used by cavity-nesting vertebrates declines in harvested forests: tree cavity persistence in harvested forest. J Wildl Manage. 77:770–776. doi:10.1002/jwmg.526.
- Edworthy AB, Trzcinski MK, Cockle KL, Wiebe KL, Martin K. 2018. Tree cavity occupancy by nesting vertebrates across cavity age. Jour Wild Mgmt. 82:639–648. doi:10.1002/jwmg.21398.
- Edworthy AB, Wiebe KL, Martin K. 2012. Survival analysis of a critical resource for cavity-nesting communities: patterns of tree cavity longevity. Ecol Appl. 22:1733–1742. doi:10.1890/11-1594.1.
- Fuller RM, Devereux BJ, Gillings S, Amable GS. 2005. Indices of bird-habitat preference from field surveys of birds and remote sensing of land cover: a study of south-eastern England with wider implications for conservation and biodiversity assessment. Glob Ecol Biogeogr. 14:223–239. doi:10.1111/j.1466-822X.2005.00145.x.
- Goodenough AE, Elliot SL, Hart AG. 2009. Are nest sites actively chosen? Testing a common assumption for three non-resource limited birds. Acta Oecol. 35:598–602. doi:10.1016/j.actao.2009.05.003.
- Gutzat F, Dormann CF. 2018. Decaying trees improve nesting opportunities for cavity-nesting birds in temperate and boreal forests: a meta-analysis and implications for retention forestry. Ecol Evol. 8:8616–8626. doi:10.1002/ece3.4245.
- Hardenbol AA, Pakkala T, Kouki J. 2019. Persistence of a keystone microhabitat in boreal forests: cavities of Eurasian three-toed woodpeckers (Picoides tridactylus). For Ecol Manag. 450:117530. doi:10.1016/j.foreco.2019.117530.
- Hartig F. 2022. DHARMa: residual diagnostics for hierarchical (multi-level / mixed) regression models. R Package version 0.4.5.
- Hebda G, Wesołowski T, Rowiński P. 2017. Nest sites of a strong excavator, the great spotted woodpecker Dendrocopos major, in a primeval forest. Ardea. 105:61–71. doi:10.5253/arde.v105i1.a8.
- Hui G, Zhang G, Zhao Z, Yang A. 2019. Methods of forest structure research: a review. Curr Forestry Rep. 5:142–154. doi:10.1007/s40725-019-00090-7.
- Keller V, Herrando S, Voríšek P, Franch M, Kipson M, Milanesi P, Martí D, Anton M, Klvanová A, Kalyakin MV, Bauer HG. 2020. European breeding bird atlas 2: Distribution, abundance and change. Barcelona: European Bird Census Council & Lynx Edicions.
- Koenig WD, Hallock EM, Weber DJ, Walters EL. 2021. Nest cavity reuse by the cooperatively breeding acorn woodpecker. Ornithology. 138:ukaa088. doi:10.1093/ornithology/ukaa088.
- Kouki J, Löfman S, Martikainen P, Rouvinen S, Uotila A. 2001. Forest fragmentation in Fennoscandia: linking habitat requirements of wood-associated threatened species to landscape and habitat changes. Scand J For Res. 16:27–37. doi:10.1080/028275801300090564.
- Krebs JR. 1971. Territory and breeding density in the great tit, Parus major L. Ecology. 52:3–22. doi:10.2307/1934734.
- Lemel J. 1989. Habitat distribution in the great tit Parus major in relation to reproductive success, dominance, and biometry. Ornis Scand (Scand J Ornithology). 20:226–233. doi:10.2307/3676917.
- Lüdecke D, Makowski D, Waggoner P, Patil I., Waggoner P, Makowski D 2021. Performance: an R package for assessment, comparison and testing of statistical models. JOSS. 6:3139. doi:10.21105/joss.03139.
- Lundberg A, Alatalo RV. 2010. The pied flycatcher. London: Bloomsbury Publishing.
- Määttänen A-M, Virkkala R, Leikola N, Heikkinen RK. 2022. Increasing loss of mature boreal forests around protected areas with red-listed forest species. Ecol Process. 11:17. doi:10.1186/s13717-022-00361-5.
- MacArthur RH, MacArthur JW. 1961. On bird species diversity. Ecology. 42:594–598. doi:10.2307/1932254.
- Mägi M, Mänd R, Tamm H, Sisask E, Kilgas P, Tilgar V. 2009. Low reproductive success of great tits in the preferred habitat: a role of food availability. Écoscience. 16:145–157. doi:10.2980/16-2-3215.
- Mäkisara K, Katila M, Peräsaari J. 2019. The multi-source national forest inventory of Finland – methods and results 2015. Luonnonvarakeskus.
- Mänd R, Leivits A, Leivits M, Rodenhouse NL. 2009. Provision of nestboxes raises the breeding density of great tits Parus major equally in coniferous and deciduous woodland. Ibis. 151:487–492. doi:10.1111/j.1474-919X.2009.00929.x.
- Mänd R, Tilgar V, Lõhmus A, Leivits A. 2005. Providing nest boxes for hole-nesting birds – does habitat matter? Biodivers Conserv. 14:1823–1840. doi:10.1007/s10531-004-1039-7.
- Martin K, Aitken KEH, Wiebe KL. 2004. Nest sites and nest webs for cavity-nesting communities in interior British Columbia, Canada: nest characteristics and niche partitioning. Condor. 106:5–19. doi:10.1093/condor/106.1.5.
- Martin K, Eadie JM. 1999. Nest webs: a community-wide approach to the management and conservation of cavity-nesting forest birds. For Ecol Manag. 115:243–257. doi:10.1016/S0378-1127(98)00403-4.
- Maziarz M, Broughton RK, Wesołowski T. 2017. Microclimate in tree cavities and nest-boxes: implications for hole-nesting birds. For Ecol Manag. 389:306–313. doi:10.1016/j.foreco.2017.01.001.
- Maziarz M, Wesołowski T, Hebda G, Cholewa M, Broughton RK. 2016. Breeding success of the great tit Parus major in relation to attributes of natural nest cavities in a primeval forest. J Ornithol. 157:343–354. doi:10.1007/s10336-015-1294-2.
- McComb WC, Noble RE. 1981. Nest-Box and natural-cavity use in three Mid-south forest habitats. J Wildl Manage. 45:93–101. doi:10.2307/3807877.
- Naimi B, a s. Hamm N, Groen TA, Skidmore AK, Toxopeus AG. 2014. Where is positional uncertainty a problem for species distribution modelling? Ecography. 37:191–203. doi:10.1111/j.1600-0587.2013.00205.x.
- Ouellet-Lapointe U, Drapeau P, Cadieux P, Imbeau L. 2012. Woodpecker excavations suitability for and occupancy by cavity users in the boreal mixedwood forest of eastern Canada. Écoscience. 19:391–397. doi:10.2980/19-4-3582.
- Pakkala T, Tiainen J, Kouki J. 2017. The importance of nesting cavity and tree reuse in the three-toed woodpecker Picoides tridactylus in dynamic forest landscapes. Ann Zool Fenn. 54:175–191. doi:10.5735/086.054.0116.
- Pakkala T, Tiainen J, Pakkala H, Piha M, Kouki J. 2020. Nest tree characteristics of grey-headed woodpeckers (Picus canus) in boreal forests. Ornis Fenn. 97:89–100.
- Pakkala T, Tiainen J, Piha M, Kouki J. 2018a. How important are nest cavities made by the three-toed woodpecker Picoides tridactylus for cavity-nesting forest bird species? Acta Ornithologica. 53:69–79. doi:10.3161/00016454AO2018.53.1.007.
- Pakkala T, Tiainen J, Piha M, Kouki J. 2018b. Three-toed woodpecker cavities in trees: a keystone structural feature in forests shows decadal persistence but only short-term benefit for secondary cavity-breeders. For Ecol Manag. 413:70–75. doi:10.1016/j.foreco.2018.01.043.
- Pasinelli G. 2007. Nest site selection in middle and great spotted woodpeckers Dendrocopos medius & D. major: implications for forest management and conservation. Biodivers Conserv. 16:1283–1298. doi:10.1007/s10531-007-9162-x.
- Peltola A, Räty M, Sauvula-Seppälä T, Torvelainen J, Uotila E, Vaahtera E, Ylitalo E. 2020. Suomen metsätilastot 2020. Helsinki: Luonnonvarakeskus (Luke).
- Potti J, Camacho C, Canal D, Martinez-Padilla J. 2018. Long-term occupancy of nest boxes as a measure of territory quality for pied flycatchers. J Field Ornithol. 89:337–347. doi:10.1111/jofo.12266.
- Purcell KL, Verner J, Oring LW. 1997. A comparison of the breeding ecology of birds nesting in boxes and tree cavities. Auk. 114:646–656. doi:10.2307/4089284.
- QGIS.org. 2021. QGIS Geographic Information System.
- R Core Team. 2022. R: a language and environment for statistical computing. Vienna, Austria: R Foundation for Statistical Computing. URL https://www.R-project.org/.
- Remm J, Lõhmus A. 2011. Tree cavities in forests – the broad distribution pattern of a keystone structure for biodiversity. For Ecol Manag. 262:579–585. doi:10.1016/j.foreco.2011.04.028.
- Remm J, Lõhmus A, Remm K. 2006. Tree cavities in riverine forests: what determines their occurrence and use by hole-nesting passerines? For Ecol Manag. 221:267–277. doi:10.1016/j.foreco.2005.10.015.
- Rhodes CJ, Henrys P, Siriwardena GM, Whittingham MJ, Norton LR. 2015. The relative value of field survey and remote sensing for biodiversity assessment. Methods Ecol Evol. 6:772–781. doi:10.1111/2041-210X.12385.
- Rönkä M, Tolvanen H, Lehikoinen E, von Numers M, Rautkari M. 2008. Breeding habitat preferences of 15 bird species on south-western Finnish archipelago coast: applicability of digital spatial data archives to habitat assessment. Biol Conserv. 141:402–416. doi:10.1016/j.biocon.2007.10.010.
- Rosenvald R, Lõhmus A, Kraut A, Remm L. 2011. Bird communities in hemiboreal old-growth forests: the roles of food supply, stand structure, and site type. For Ecol Manag. 262:1541–1550. doi:10.1016/j.foreco.2011.07.002.
- Russell LV. 2022. Emmeans: estimated marginal means, aka least-squares means. R Package version 1.7.2.
- Rytkönen S, Krams I. 2003. Does foraging behaviour explain the poor breeding success of great tits Parus major in northern Europe? J Avian Biol. 34:288–297. doi:10.1034/j.1600-048X.2003.03041.x.
- Rytkönen S, Orell M. 2001. Great tits, Parus major, lay too many eggs: experimental evidence in mid-boreal habitats. Oikos. 93:439–450. doi:10.1034/j.1600-0706.2001.930309.x.
- Rytkönen S, Vesterinen EJ, Westerduin C, Leviäkangas T, Vatka E, Mutanen M, Välimäki P, Hukkanen M, Suokas M, Orell M. 2019. From feces to data: a metabarcoding method for analyzing consumed and available prey in a bird-insect food web. Ecol Evol. 9:631–639. doi:10.1002/ece3.4787.
- Sandström U. 1991. Enhanced predation rates on cavity bird nests at deciduous forest edges: an experimental study. Ornis Fenn. 68:93–98.
- Sasvari L. 1991. Territoriality in the great tit Parus major at low and high densities. Bird Behav. 9:88–93. doi:10.3727/015613890791749082.
- Schwartz T, Genouville A, Besnard A. 2020. Increased microclimatic variation in artificial nests does not create ecological traps for a secondary cavity breeder, the European roller. Ecol Evol. 10:13649–13663. doi:10.1002/ece3.6871.
- Serrano-Davies E, Barrientos R, Sanz JJ. 2017. The role of nest-box density and placement on occupation rates and breeding performance: a case study with Eurasian blue tits. Ornis Fenn. 94:21–32. doi:10.13039/501100004837.
- Siitonen J, Martikainen P, Punttila P, Rauh J. 2000. Coarse woody debris and stand characteristics in mature managed and old-growth boreal mesic forests in southern Finland. For Ecol Manag. 128:211–225. doi:10.1016/S0378-1127(99)00148-6.
- Söderström B. 2009. Effects of different levels of green- and dead-tree retention on hemi-boreal forest bird communities in Sweden. For Ecol Manag. 257:215–222. doi:10.1016/j.foreco.2008.08.030.
- Sudyka J, Di Lecce I, Wojas L, Rowiński P, Szulkin M. 2022. Nest-boxes alter the reproductive ecology of urban cavity-nesters in a species-dependent way. J Avian Biol. doi:10.1111/jav.03051.
- Suhonen J, Alatalo RV, Gustafsson L. 1994. Evolution of foraging ecology in Fennoscandian tits (Parus spp). Proc R Soc London Series B: Biol Sci. 258:127–131. doi:10.1098/rspb.1994.0152.
- SYKE. 2021. Finnish environment institute (SYKE), downloadable spatial datasets.
- Virkkala R. 2004. Bird species dynamics in a managed southern boreal forest in Finland. For Ecol Manag. 195:151–163. doi:10.1016/j.foreco.2004.02.037.
- Virkkala R, Leikola N, Kujala H, Kivinen S, Hurskainen P, Kuusela S, Valkama J, Heikkinen RK. 2022. Developing fine-grained nationwide predictions of valuable forests using biodiversity indicator bird species. Ecol Appl. doi:10.1002/eap.2505.
- Virkkala R, Rajasärkkä A, Väisänen RA, Vickholm M, Virolainen E. 1994. Conservation value of nature reserves: do hole-nesting birds prefer protected forests in southern Finland? Ann Zool Fenn. 31:173–186.
- Volke U, Vahter I, Volke V. 2010. Nest site selection of the great spotted woodpecker (Dendrocopos major) in Saaremaa. Hirundo. 23:53–62.
- Wesołowski T. 2011. “Lifespan” of woodpecker-made holes in a primeval temperate forest: a thirty year study. For Ecol Manag. 262:1846–1852. doi:10.1016/j.foreco.2011.08.001.
- Wesołowski T, Rowiński P. 2012. The breeding performance of blue tits Cyanistes caeruleus in relation to the attributes of natural holes in a primeval forest. Bird Study. 59:437–448. doi:10.1080/00063657.2012.722189.
- Wiebe KL, Cockle KL, Trzcinski MK, Edworthy AB, Martin K. 2020. Gaps and runs in nest cavity occupancy: cavity “destroyers” and “cleaners” affect reuse by secondary cavity nesting vertebrates. Front Ecol Evol. 8:205. doi:10.3389/fevo.2020.00205.
- Zahner V, Sikora L, Pasinelli G. 2012. Heart rot as a key factor for cavity tree selection in the black woodpecker. For Ecol Manag. 271:98–103. doi:10.1016/j.foreco.2012.01.041.
- Zawadzka D, Drozdowski S, Zawadzki G, Zawadzki J. 2016. The availability of cavity trees along an age gradient in fresh pine forests. Silva Fenn. 50:article id 1441. doi:10.14214/sf.1441.