Abstract
Medulloblastoma (MB) is a primitive neuroectodermal tumour constituting a grade IV brain malignancy. Early and correct detection of recurrence or metastasis is desirable for follow-up of patients in this entity. Frequent expression of somatostatin receptors by MB lesions facilitates functional tumour imaging by somatostatin receptor scintigraphy (SRS). To investigate the value of SRS in the follow-up of MB, the results of ten consecutive patients (seven children and three adults) undergoing additional imaging with 111In-pentetreotide were reviewed. Four, 24 and 48 h p.i. planar and whole body images as well as a SPECT study at 4 h p.i. were acquired after intravenous injection of 109±35 MBq 111In-pentetreotide (Octreoscan®). SRS yielded 11 positive and ten negative imaging results, compared to 17 positive and four negative in magnetic resonance imaging (MRI). The lesion-by-lesion analysis with a total of 44 lesions revealed a sensitivity, specificity, positive predictive value (PPV) and negative predictive value (NPV) of 42%, 83%, 94%, 18% for SRS and 89.5%, 50%, 92%, 43% for MRI. Based on a per-patient analysis, considering the patient as to be either tumour-free or tumour-positive by one imaging modality, the following values for sensitivity, specificity, PPV and NPV were obtained: 61%, 100%, 100%, 30% for SRS and 94%, 67%, 94%, 67% for MRI. MRI remains the first step imaging technique in medulloblastoma patients before and after surgery and during the follow-up providing the highest sensitivity. However, to improve specificity and contribute to correct diagnosis in MB 111In-pentetreotide scintigraphy should be considered as a confirmatory second step imaging tool, especially in case of equivocal MRI results. Moreover, a positive SRS scan might serve as a reference before and after somatostatin receptor targeted radiotherapy.
Body
Medulloblastoma (MB) is a primitive neuroectodermal tumour (PNET) considered a grade IV brain malignancy Citation[1]. The diagnosis of MB is based on histopathological examination and its localization on magnetic resonance imaging (MRI). Since surgical resection of MB is not always successful, external radiotherapy (RTx) and chemotherapy (CTx) are part of adjuvant treatment protocols. After combined RTx and CTx, MB patients have an overall 5-year-progression- and event-free survival rate of 85±6% and 83±6%, respectively, but those patients with metastatic disease at the time of diagnosis have a progression-free survival of 67±15% compared to those with localized disease of 90±6% Citation[2]. A careful and appropriate follow-up strategy for early and correct detection of recurrence or metastases is required given the high incidence of metastases (30 – 40%) and tumour recurrence (40 – 60%) Citation[3].
Neuroectodermal tumours show high levels of expression of somatostatin receptors, which allows effective tumour imaging with 111In labelled somatostatin analogues Citation[4], Citation[5]. It has been demonstrated that MB lesions also express somatostatin receptors, mainly the subtypes sst − 1 and sst − 2 Citation[6–8]. The radio labelled somatostatin analogue 111In-pentetreotide (Octreoscan®) has the highest affinity for sst − 2 and can be used for tumour imaging in MB after surgery. To our knowledge, only one study has been published on this modality selectively in medulloblastoma patients Citation[7], apart from case reports. However, this the first study in which the SRS and MRI findings were evaluated during the follow-up period per-lesion basis. In view of potential adjuvant treatment via somatostatin receptor mediated targeted radiotherapy Citation[9], somatostatin receptor scintigraphy (SRS) was performed in addition to MRI. For the purpose of this retrospective study, we analyzed the results of both imaging studies in order to investigate the potential diagnostic role of 111In-pentetreotide scintigraphy in the follow-up of medulloblastoma as compared to MRI.
Material and methods
A total of ten medulloblastoma patients, seven children (mean age 9±3.3 yrs, range 4 – 14 years.; two females and five males) and three male adults with an age of 26, 27 and 28 years at the time of diagnosis were retrospectively included in this study. All patients had undergone SRS with 111In-pentetreotide in addition to MRI during the follow-up period after surgical removal of the primary tumour. No treatments were performed between both examinations, SRS and MRI, with a median time interval in between of 9 days (range 1 – 46). In total, 21 pairs of imaging modalities (SRS and MRI) were available for comparison. All patients received RTx and/or CTx in the course of disease. Signed informed consent was obtained from the patients and/or their parents.
111In-Pentetreotide Scintigraphy
A total of 21 111In-pentetreotide scans were performed in ten patients. All patients were injected with 109±35 MBq (37 – 177 MBq) 111In-pentetreotide (Octreoscan®, Mallinckrodt Diagnostics, Petten, The Netherlands) intravenously. Four, 24 and 48 h p.i. skull planar and whole body images were acquired. A SPECT study of the head was performed at 4 h p.i. using a dual-head gamma camera equipped with medium-energy general purpose collimators settled to the photopeaks of 173 and 247 keV and a window of 20%. Planar images were recorded with a matrix of 128×128 for 15 minutes. Sixty-four projections with an acquisition time of 40 sec/view were acquired in a 64×64 matrix for SPECT study. Reconstructed coronal and transversal images were interpreted without knowledge of the clinical data.
MRI
MRI was performed on a 1.5 Tesla system (Intera or NT, PHILIPS Medical System, Best, The Netherlands) equipped with a standard gradient-system (amplitude 30 mT/s, rise time 0.2 msec). Axial T2-weighted Turbospinecho-, FLAIR- (Fluid Attenuated Inversion Recovery) and T1-weighted Spin-echo-sequences were employed. After intravenous application of 0.2 mmol Gd-DTPA (Magnevist®) / kg bodyweight T1-weighted SE-sequences in axial, sagittal and coronal planes were acquired.
Image Analysis
The MRI images were judged by a neuroradiologist, blinded to the scintigraphic results and clinical data. Contrast medium enhanced lesions in the MRI scan were interpreted as tumourous (recurrence in the primary tumour location or metastatic lesions adjacent to CSF spaces).
In contrast with Müller et al. Citation[7], where conventional imaging modalities were used as gold standard, we used the findings of both imaging modalities, SRS and MRI, during the course of therapy and/or follow-up examinations of each patient, and correlated the findings with each other to define true and false imaging. Definite tumour progression or therapy induced tumour regression was considered as validation of a true tumour lesion. Imaging with SRS or MRI was defined as overall positive if at least one positive lesion was present resulting in the diagnosis of tumour presence by the given imaging modality.
Results
The patient data, SRS and MRI results are presented in . Nine patients died after a mean survival time of 73.1 months (range 9 – 156) after initial diagnosis. Fifty five percent (5/9) of patients had 5 year progression-free survival. Only one patient survived at the time of the final study analysis (15 months + ).
Table I. Patient data, SRS and MRI results.
Altogether, 21 pairs of SRS/MRI examinations in ten patients were available for analysis. In total, 44 lesions were displayed either by SRS or MRI. illustrates two of the three intra-cranial matching SRS and MRI findings of patient number three. The results of the per-lesion analysis are shown in . MRI achieved a high per-lesion sensitivity (89.5%) compared to a low per-lesion sensitivity of SRS (42.1%). On the other hand, SRS exhibited a higher specificity compared to MRI (83.3% versus 50%). The only false positive lesion of SRS is shown in , depicting slightly increased tracer uptake in the proximity of the site of primary surgery and radiation therapy. The false negative intra-cranial findings of SRS were mainly due to small lesion size (≤1 cm). False negative spinal SRS lesions were either too small in size and/or obscured by the physiologic uptake of adjacent organs such as the liver or attenuated by vertebral bone. The per-patient analysis considering the overall statement (patient either tumour-positive or free of tumour) of one imaging modality for one patient at one time point () yielded eleven positive and ten negative imaging results with SRS, compared to 17 positive and four negative results with MRI. The seven false negative SRS scans compared to only one false negative MRI examination. The per-patient sensitivity/specificity of SRS and MRI was 61%/100% and 94%/67%, respectively. In one case, SRS revealed multiple previously unknown metastases outside the central nervous system (CNS), i.e. the bone, lungs and abdomen (), which could then be confirmed by ultrasound and MRI.
Figure 1. Corresponding coronal images of 111In-pentetreotide SPECT (A) and MRI (B,C) in patient three depicting two tumor lesions in a concordant manner. The right temporal lesion (A: arrow; B: arrow) and the fronto-basal lesion (A: arrowhead; C: arrowheads) are easily detected by both imaging modalities. The third tumor lesion of the patient seen by both scans is not depicted here.
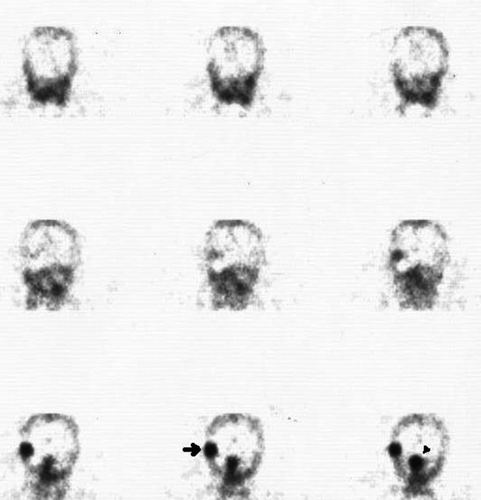
Figure 2. 111In-pentetreotide scintigraphy (A) and MRI (B) of patient number eight demonstrate a potential source of false positive SRS imaging. The sagittal SPECT image (A) shows a slightly increased tracer uptake diffuse in the occipital and sub-occipital area (arrows), while the MRI (B) depicts only postoperative defects and a clip artefact (arrow) after primary tumor resection but no sign of tumor recurrence. This form of unspecific tracer uptake is most likely caused by regenerative processes after surgery and radiation.
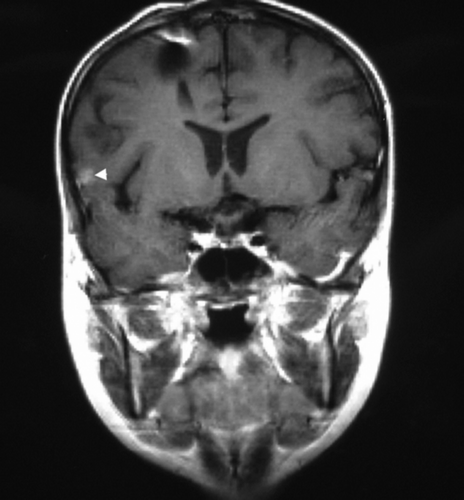
Figure 3. 111In-pentetreotide whole body scintigraphy of patient number nine. The scan shows multiple metastases to the bone, lungs and abdomen.
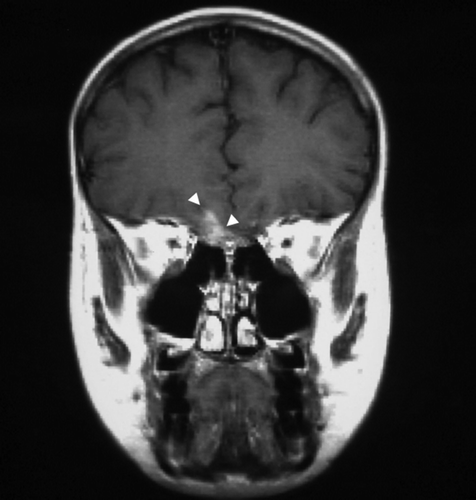
Table II. Per-lesion analysis.
Table III. Per-patient analysis.
Discussion
MB is the most common brain tumour in childhood, and arises mostly in the vermis of the cerebellum with a peak incidence at seven years Citation[1]. It is seen much less common in adults. WHO considers childhood MB as a grade IV brain tumour. The prognosis depends on the status of the MB at the time of diagnosis and the success of the primary surgical intervention. Patients with localized disease at the time of diagnosis and/or a total or near total resection have a more favourable prognosis than those with disseminated disease and/or incomplete tumour resection. As the tumour cannot be totally removed in many cases, RTx and CTx are the next steps in patient management. Combined use of RTx and CTx improves the outcome and progression-free survival of MB patients Citation[2]. Although MB is easily localized by CT, MRI allows a more sensitive detection of the primary tumour and its relationship to adjacent CNS structures Citation[10].
In MB patients, the following nuclear medicine procedures are performed: bone scan Citation[11]; 99mTc-HMPAO or 99mTc-–ECD in neurological complications like mutism Citation[12], Citation[13]; 111In-DTPA for the proof of cerebro-spinal fluid (CSF) flow Citation[14]; 123I-MIBG Citation[15], 201Tl Citation[16], Citation[17], 111In-pentetreotide Citation[7], Citation[18] and FDG-PET Citation[19], Citation[20] for tumour and/or recurrence detection. Among these procedures, the 111In labelled somatostatin analogue pentetreotide shows the most promising results due to high expression of somatostatin receptors, mainly sst2, in MB Citation[8]. Since radio labelled octreotide analogues can be used for diagnosis as well as for radionuclide therapy, the diagnostic demonstration of tracer accumulation may also serve as a basis for alternative treatment assignment. For uptake of 111In-pentetreotide in brain tumours, the blood-brain barrier must be disrupted, since the water-soluble peptide octreotide does not cross the intact barrier Citation[7], Citation[21]. Thus, false negative medulloblastoma scintigraphy could be caused by absent disruption of the blood brain barrier (BBB). Evaluating the BBB with 99mTc-DTPA, after injection intravenously, gives valuable information whether the BBB intact or not Citation[7], Citation[21]. A positive uptake of 99mTc-DTPA reveals disruption of BBB. Therefore, performing a dual isotope scintigraphy with 111In-pentetreotide and 99mTc-DTPA suggests that the uptake in SRS shows the presence of the tumour tissue. Moreover, as all recurrent medulloblastoma lesions in the study of Müller et al. Citation[7] demonstrated uptake of 111In-pentetreotide it was concluded that this functional imaging method is specific for somatostatin receptor positive recurrent MB lesions.
The per-lesion and per-patient sensitivity of MRI is high (89.5% and 94.4%, respectively) and compares to poor corresponding values for SRS (42.1% and 61.1%, respectively). False negative findings of SRS were mainly due to small lesion size (≤1cm) or — in the case of spinal metastatic lesions — caused by absorbing or obscuring adjacent structures such as the vertebral column or organs with high tracer uptake such as the liver. However, specificity of SRS proved to be better than that of MRI, for both the per-lesion (83.3% versus 50%) and per-patient (100% versus 66.7%) specificity. This underlines the specificity of SRS in MB suggested by earlier studies. From our results, it can be concluded that MRI remains the first step-imaging tool in MB. 111In-pentetreotide scintigraphy should be added to the follow-up as a confirmatory imaging tool after positive or equivocal MRI, allowing further differentiation between viable tumour and scar tissue. Pathological scintigraphic tracer uptake significantly substantiates MRI positive findings. This might influence the decision making and patient management. Moreover, as seen in one of our patients (), 111In-pentetreotide scintigraphy permits easy and comprehensive detection of possible distant metastases by whole body scan acquisition without further radiation exposure.
The short overall survival in our patient group (5-year survival <60%) compared to the reported 5-year progression-free survival of 85% Citation[2] reflects the selection of “high risk” patients with initial metastases or positive MRI findings during follow-up in our study. Usually, patients were not examined with SRS in the absence of abnormalities in the follow-up. This results in selection of patients with an overall poorer prognosis which should account for the reduced survival rate in our study group. Nevertheless, it is in those patients with frequent abnormal findings during follow-up or after treatments, where SRS may prove helpful in addition to MRI as opposed to patients free of disease with an uneventful follow-up.
Based on the high expression of sst2 and high affinity binding of the somatostatin analogues in MB, 111In-pentetreotide scintigraphy may also be used pre-operatively in view of gamma-probe guided surgery, which may facilitate complete tumour resection, as seen in other gamma-probe guided removed tumour types Citation[23], Citation[24]. Since MB is a radiosensitive tumour, RTx is very important for successful therapy. Unfortunately, RTx and CTx have side effects such as endocrinological (growth failure, hypothyroidism) and cognitive deficits or ototoxicity. These effects were pronounced in young children being treated with these procedures Citation[25]. The combined use of RTx and CTx implies a negative synergetic effect regarding the risk of secondary cancer development, e.g. acute leukemia Citation[26] and secondary intra-cranial meningioma Citation[27]. Thus, another contribution of 111In-pentetreotide scintigraphy might be the pre-therapeutic evaluation for either intra-thecal or systemic administration of radiolabelled octreotide analogues, since targeted radiotherapy is associated with less side effects than X-RTx. Previously published studies show promising results of radionuclide therapy in brain tumours, such as 125I-seeds Citation[28], radiolabelled monoclonal antibodies Citation[29–31] and somatostatin analogues Citation[7], Citation[9], Citation[32–34], however, more studies will be needed to define the role of these treatment modalities in MB and/or PNET. Recently, Vaidyanathan at al. Citation[34] found that glucose conjugated octreotide analogues like 131I-Gluc-TOC and 131I-Gluc-TOCA showed increased tumour uptake in human medulloblastoma xenografts compared to 131I-TOCA, yielding higher tumour-to-background ratios.
Conclusion
In conclusion, MRI is the first line imaging technique in medulloblastoma patients before and after surgery, as well as during follow-up providing a high sensitivity. 111In-pentetreotide scintigraphy could be added in a second step, especially in case of ambiguous MRI results, in order to improve diagnostic accuracy and optimize patient management after surgery and during follow-up. Apart from that, whole body imaging facilitates the detection of SRS-positive distant metastases. Another purpose of adding this scintigraphic imaging modality is the evaluation for targeted radionuclide therapy as a less toxic alternative for conventional external beam radiotherapy.
Dr. M. Yüksel was supported by a grant from TUBITAK-DFG (Scientific and Technical Research Council of Turkey-Deutsche Forschungs Gemeinschaft), and Dr. Z. Gao was supported by a grant from the DAAD (Deutscher Akademischer Austausch Dienst).
References
- Reddy AT, Packer RJ. Medulloblastoma. Curr Opin Neurol 1999; 12: 681–5
- Packer RJ, Sutton LN, Elterman R, Lange B, Goldwein J, Nicholson, et al. Outcome for children with medulloblastoma treated with radiation and cisplatin, CCNU, and vincristine chemotherapy. J Neurosurg 1994; 81: 690–8
- Dunkel IJ, Finlay JL. High dose chemotherapy with autologues stem cell rescue for patients with medulloblastoma. J Neurooncol 1996; 29: 69–74
- Kwekkeboom DJ, Krenning EP. Somatostatin receptor imaging. Semin Nucl Med 2002; 32: 84–91
- Breeman WA, de Jong M, Kwekkeboom DJ, Valkema R, Bakker WH, Kooij PP, et al. Somatostatin receptor-mediated imaging and therapy: Basic science, current knowledge, limitations and future perspectives. Eur J Nucl Med 2001; 28: 1421–9
- Reubi JC, Lang W, Maurer R. Distribution and biochemical characterization of somatostatin receptors in tumors of the human central nervous system. Cancer Res 1987; 47: 5758–64
- Muller HL, Fruhwald MC, Scheubeck M, Rendl J, Warmuth-Metz M, Sorensen N, et al. A possible role for somatostatin receptor scintigraphy in the diagnosis and follow-up of children with medulloblastoma. J Neurooncol 1998; 38: 27–40
- Fruhwald MC, O'Dorisio MS, Pietsch T, Reubi JC. High expression of somatostatin receptor subtype 2 (sst2) in medulloblastoma: Implications for diagnosis and therapy. Pediatr Res 1999; 45: 697–708
- Beutler D, Avoledo P, Reubi JC, Macke HR, Muller-Brand J, Merlo A, et al. Three-year recurrence-free survival in a patient with recurrent medulloblastoma after resection, high-dose chemotherapy, and intrathecal Yttrium-90-labeled DOTA(0)-D-Phe(1)-Tyr(3)-octreotide radiopeptide brachytherapy. Cancer 2005; 103: 869–73
- Meyers SP, Kemp SS, Tarr RW. MR imaging features of medullobastomas. Am J Roentgenol 1992; 158: 859–65
- Tomita T, Das L, Radkowski MA. Bone metastases of medulloblastoma in childhood; correlation with flow cytometric DNA analysis. J Neurooncol 1990; 8: 113–20
- Ersahin Y, Yararbas U, Duman Y, Mutluer S. Single photon emission tomography following posterior fossa surgery in patients with and without mutism. Childs Nerv Syst 2002; 18: 318–25
- Sagiuchi T, Ishii K, Asano Y, Aoki Y, Kikuchi K, Jinguuji K, et al. Bilateral crossed cerebello-cerebral diaschisis and mutism after surgery for cerebellar medulloblastoma. Ann Nucl Med 2001; 15: 157–60
- Chamberlain MC. Pediatric leptomeningeal metastasis: 111In-DTPA cerebrospinal fluid flow studies. J Child Neurol 1994; 9: 150–4
- Naitoh Y, Sasajima T, Kinouchi H, Mikawa S, Mizoi K. Medulloblastoma with extensive nodularity: Single photon emission CT study with iodine-123 metaiodobenzylguanidine. Am J Neuroradiol 2002; 23: 1564–7
- Rollins NK, Lowry PA, Shapiro KN. Comparison of gadolinium-enhanced MR and thallium-201 single photon emission computed tomography in pediatric brain tumors. Pediatr Neurosurg 1995; 22: 8–14
- Lee JD, Kim DI, Lee JT, Chang JW, Park CY. Indium-111-pentetreotide imaging in intra-axial brain tumors: Comparison with thallium-201 SPECT and MRI. J Nucl Med 1995; 36: 537–41
- Hervas I, Perez-Velasco R, Bello P, Mateo A. In-111 octreotide scintigraphy in adult medulloblastoma. Clin Nucl Med 2003; 28: 343–4
- Holthoff VA, Herholz K, Berthold F, et al. In vivo metabolism of childhood posterior fossa tumors and primitive neuroectodermal tumors before and after treatment. Cancer 1993; 72: 1394–1403
- Mineura K, Sasajima T, Kowada M, Shishido F, Uemura K. Demonstration of cerebral radiation injury with metabolic positron emission tomography images. Pediatr Neurosurg –92 1991; 17: 200–2
- Haldemann AR, Rosler H, Barth A, Waser B, Geiger L, Godoy N, et al. Somatostatin receptor scintigraphy in central nervous system tumors: Role of blood-brain barrier permeability. J Nucl Med 1995; 36: 403–10
- Rollins N, Mendelsohn D, Mulne A, Barton R, Reyes N, et al. Recurrent medulloblastoma: Frequency of tumor enhancement on Gd-DTPA MR imaging. Am J Roentgenol 1990; 155: 153–7
- Adams S, Baum RP. Intraoperative use of gamma-detecting probes to localize neuroendocrine tumors. Q J Nucl Med 2000; 44: 59–67
- Anderson NE. Late complications in childhood central nervous system tumour survivors. Curr Opin Neurol 2003; 16: 677–83
- Martelli H, Ricard M, Larroquet M, Wioland M, Paraf F, Fabre M, et al. Intraoperative localization of neuroblastoma in children with 123I- or 125I-radiolabeled metaiodobenzylguanidine. Surgery 1998; 123: 51–7
- Caglar K, Unal S, Cetinkaya A, Gumruk F, Yetgin S. Acute lymphoblastic leukemia as a second malignant neoplasm in a child with medulloblastoma. Pediatr Hematol Oncol 2003; 20: 535–7
- Strojan P, Popovic M, Jereb B. Secondary intracranial meningiomas after high-dose cranial irradiation: Report of five cases and review of the literature. Int J Radiat Oncol Biol Phys 2000; 48: 65–73
- Rostomily RC, Halligan J, Geyer R, Stelzer K, Lindsley K, Berger MS. Permanent low-activity (125)I seed placement for the treatment of pediatric brain tumors: Preliminary experience. Pediatr Neurosurg 2001; 34: 198–205
- Coakham HB, Kemshead JT. Treatment of neoplastic menegitis by targeted radiation using 131I-radiolabelled monoclonal antibodies. J Neuro-Oncol 1998; 38: 225–32
- Cokgor I, Akabani G, Kuan CT, Friedman HS, Friedman AH, Coleman RE, et al. Phase I trial results of iodine-131-labeled antitenascin monoclonal antibody 81C6 treatment of patients with newly diagnosed malignant gliomas. J Clin Oncol 2000; 18: 3862–72
- Reardon DA, Akabani G, Coleman RE, Friedman AH, Friedman HS, Herndon JE 2nd, et al. Phase II trial of murine (131)I-labeled antitenascin monoclonal antibody 81C6 administered into surgically created resection cavities of patients with newly diagnosed malignant gliomas. J Clin Oncol 2002; 20: 1389–97
- Merlo A, Hausmann O, Wasner M, Steiner P, Otte A, Jermann E, et al. Locoregional regulatory peptide receptor targeting with the diffusible somatostatin analogue 90Y-labeled DOTA0-D-Phe1-Tyr3-octreotide (DOTATOC): A pilot study in human gliomas. Clin Cancer Res 1999; 5: 1025–33
- Schumacher T, Hofer S, Eichhorn K, Wasner M, Zimmerer S, Freitag P, et al. Local injection of the 90Y-labelled peptidic vector DOTATOC to control gliomas of WHO grades II and III: An extended pilot study. Eur J Nucl Med Mol Imaging 2002; 29: 486–93
- Vaidyanathan G, Friedman HS, Affleck DJ, Schottelius M, Wester HJ, Zalutsky MR. Specific and high-level targeting of radiolabelled octreotide analogues to human medulloblastoma xenografts. Clin Cancer Res 2003; 9: 1868–76