Abstract
The aim of this study was to assess the set-up uncertainties and the possible CTV-PTV margin reduction when adopting daily IGRT. Further, to identify any intrafraction time trends in the prostate movements to ensure the margin reduction gained from IGRT. Fifteen prostate cancer patients treated with IMRT using daily IG of three implanted fiducial markers were included. The interfraction uncertainties were assessed by statistically evaluating the daily prostate marker displacement. The intrafraction uncertainties were represented by the difference in prostate marker displacement before and after beam delivery. To evaluate any intrafraction time trends, the data points were divided into two groups with respect to time duration and statistically analysed. This study confirmed that daily IG considerably reduces the set-up uncertainties. Our results implied that if IGRT is performed on a daily basis, both systematic and random set-up errors will be reduced to a minimum, leading to a required set-up margin of only 1.5 mm. Results from measurements of intrafraction motions in time durations ranging from 2 to 27 min, indicated that a margin enlargement of 1 mm was required to account for the intrafraction uncertainties. The results did not suggest any significant time trends in the intrafraction uncertainties.
Image Guided Radiotherapy (IGRT) with the introduction of kilovoltage x-ray tubes and flat panel detectors has offered the ability to visualize internal structures and to more accurately localize the target position. Daily on-line positioning verification reduces not only the systematic, but also the random day to day variations in target position. This is of great importance for prostate treatments where the interfraction target motions can be considerable due to the emptying and filling of flexible organs nearby. Numerous studies have demonstrated a substantial reduction in geometrical uncertainties when employing new technologies to track interfraction target displacements Citation[1–3]. In conventional prostate treatments, the PTV is commonly obtained by adding a symmetric 10 to 15 mm margin to the CTV accounting for geometrical uncertainties. However, the improved target localization using daily image guidance (IG) with fiducial markers implanted in the prostate may permit smaller set-up margins. Many studies have shown that significant margin reductions can be achieved with on-line IG Citation[4–7]. Wu et al. Citation[7] concluded that a minimum of 3 mm margin is necessary to account for geometrical uncertainties with ideal image registration. However, interfraction motion is only one factor that has influence on the margin design. Other factors are the uncertainties associated with target delineation, organ deformations and intrafraction motions.
Advancements made in these IG and beam delivery techniques have enabled further conformity of the dose distribution to increase the prostate prescription dose without exceeding tolerance levels for risk organs. There is a clear dose response relationship that indicates a higher cure rate with higher dose levels, bearing in mind the prostates’ proximity to radiosensitive risk organs, such as the bladder and rectum Citation[8]. However, IGRT combined with intensity modulated radiotherapy (IMRT) tends to prolong beam delivery time and thereby increase the significance of intrafraction prostate motion. It may require more frequent verification of the actual prostate position and re-positioning of the patient during the beam delivery to facilitate margin reduction. Studies Citation[9], Citation[10] have shown that intrafraction prostate motion is related to beam delivery time. Padhani et al. Citation[9] reported significant prostate motion in a period of 7 min and suggested that appropriate margins should be applied to account for intrafraction motions. Another important factor is the rectum filling. Ghilezan et al. Citation[10] reported a 10% probability of prostate (mid-posterior) displacement larger than 3 mm after 9.5 min with empty rectum. The same probability of prostate motion was found already after 2 min with a filled rectum. Schallenkamp et al. Citation[6] concluded no significant intrafraction displacement of prostate position; however, this was assessed for standard four-field non-intensity-modulated beam delivery where shorter beam delivery duration would be expected.
The aim of this study was to:
Quantify the magnitude of inter- and intrafraction prostate marker motions when adopting daily IGRT;
Assess the potential set-up margin reduction obtained by applying daily IGRT;
Identify any intrafraction time trends in the prostate movements during IMRT delivery to ensure the margin reduction gained from daily IGRT.
Materials and methods
The IG positioning system
The IG positioning system used in this study was the commercially available product ExacTrac X-ray 6D (BrainLab AG, Heimstetten, Germany) that comprises of two integrated modules; an infra-red (IR) optical camera system and a stereoscopic kV x-ray imaging system. The two systems are calibrated to establish an internal correlation as well as to define the spatial relationship with the treatment machine's iso-center. A set-up correction based on implanted fiducial markers is assessed in the x-ray system by comparison of x-ray and CT data, and executed in the IR system by real-time tracking of the patient skin markers. A prototype of the integrated system has been investigated thoroughly by Verellen et al. Citation[11].
Patients and beam delivery strategy
This study included 15 prostate cancer patients treated to 74 or 78 Gy with 2.0 Gy per fraction. The CTV was identified as the prostate gland and the entire seminal vesicles, with a PTV margin of 5 mm in all directions. Three cylindrical gold markers (1.2×3 mm), inserted under ultrasound guidance into the prostate (close to the base, mid and apex) served as CTV surrogate. The marker insertion was carried out within one to three hours before the CT scanning. Four to six external skin markers were attached to the patient body surface in the pelvic region prior to scanning. The patients were scanned and treated in the supine position with a standard knee support and feet immobilization to ensure consistent leg position. Helical CT scans were acquired with 3 mm slices reconstructed at 2.5 mm intervals through the pelvis area and with a resolution of 0.9 mm in the transversal plane. Prior to CT scanning and treatment, the patients were instructed to attain an empty bladder and rectum.
The target delineation, treatment planning and treatment were performed either with the BrainLab system; BrainSCAN treatment planning system (TPS) and Novalis 6MV treatment machine or with the Varian system; Eclipse/Helios TPS and Clinac 21EX treatment machine. Iso-center position and CT information were uploaded from the TPS to the ExacTrac X-ray system at the treatment unit. The patients were treated with 5 to 7 coplanar IMRT fields using sliding window technique. With this delivery technique, the time span from patient positioning to the completion of a fraction typically varied from 6 to 20 min.
Prostate localization and treatment
A target “displacement” in this paper refers to the deviation of the prostate marker positions compared to the planned positions. The three implanted markers were outlined in the CT data and their positions relative to the iso-center were defined in the Exac Trac system, taken from the midpoint of the marker contour. During IG set-up, the planned marker positions relative to the iso-center, were projected on the x-ray images. The deviation was calculated in the ExacTrac software by superimposing and fusing the planned marker pattern with the pattern detected in the x-ray images using a least square match. This displacement was given in three coordinates (x, y and z) in three orthogonal directions.
The initial patient set-up was guided by the IR camera system and reflecting skin markers attached to the patient surface. The treatment couch was automatically shifted into position by the ExacTrac system corresponding to a maximum deviation of 2 mm between the actual patient position, represented by the skin markers, and the planned position. For some patients, the marker configurations were disturbed by heavy breathing motions and the initial patient set-up was accepted even though the deviations were oscillating outside the 2 mm limits. To determine the actual prostate position, the initial patient set-up was followed by acquisition of x-ray images and implanted marker fusion along with the corresponding time stamp.
In the next step the patient was repositioned using the known displacement from the initial set-up, described above. To verify this shift correction, an additional set of x-ray images was acquired. A 2 mm action limit was applied; any deviation larger than 2 mm would trigger performance of realignment, i.e. a residual set-up error of 2 mm in each direction was accepted. The displacement along with the time stamp was recorded.
Once the displacement was within the action limits, the patient was treated with the IR real time tracking of the skin markers. In situations when the skin marker positions were out of the tolerance (2 mm), the beam delivery was interrupted followed by a verification of the target marker positions and continuation of the beam delivery.
When the treatment was completed, a final target position was determined in the same manner as described above, with the purpose to evaluate the influence of treatment time on set-up uncertainties. The corresponding time stamp was also recorded.
Each treatment session thereby generated three target positions:
positioning with the reflecting skin markers only;
positioning with IG of target markers prior to treatment;
likewise but immediately after treatment.
Analysis of target displacement
The interfraction uncertainties were assessed by statistically evaluating the daily target marker displacement taken just before beam delivery. The intrafraction uncertainties were represented by the difference in the target marker displacement before and after beam delivery. To analyze if there existed a difference in the displacements, the paired t-test was chosen. A p-value < 0.05 were considered significant.
In this study, the random set-up uncertainty σset-up, was the standard deviation of the weighted random treatment set-up uncertainty for all patients Citation[13]:1 Where σinter,p is the standard deviation of the interfraction random treatment set-up error for patient p in a given direction, np the number of target displacement determined for patient p, N the total number of displacements included in the study and P the total number of patients.
The systematic set-up uncertainty ∑set-up, was the standard deviation of the systematic treatment set-up uncertainty for all patients Citation[13]:2 Where mp is the mean deviation for patient p and np displacement and moverall is the mean systematic error.
We have chosen to use the PTV margin instruction proposed by van Herk et al. Citation[14] which fully separates the effects of systematic and random errors. The required set-up margin (CTV – PTV) was calculated as 2.5∑set-up+0.7σset-up, ensuring a minimum dose of 95% to the CTV for 95% of the patient population. The set-up uncertainties, both systematic and random, which were assessed in this work, will presumably be reduced during IGRT.
To evaluate any time trends or to find any critical treatment durations with respect to target movements, the displacement recorded before and after treatment was correlated in pairs denoted as a Δ displacement and Δ time. These data were then divided in two groups, Δ time shorter and longer than the median Δ time, and statistically analysed using an unpaired t-test. A p-value < 0.05 were considered significant.
Results
The results from 15 prostate patients treated with IMRT were reported. A total of 480 treatment sessions were evaluated and 1 440 prostate locations along with time information analysed. The treatment time from the initial patient set-up to beam off was maximum 30.5 min with a mean value 9.0 min and a median value of 8.3 min.
Inter- and intrafraction analysis
In the target displacements are plotted against time. At time zero, large displacement was present due to patient set-up with skin markers. The next cluster of displacement originates from the set-up prior to treatment with the IG of target markers and the action limit of 2 mm. Finally, the third cluster visualizes the spread in target displacement that occurred during treatment.
Figure 1. Prostate displacement determined with patient set-up using skin markers and IG of target markers.
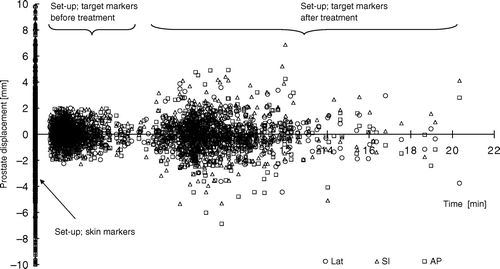
represents the frequency distribution of the target displacement monitored before (a) and after (b) beam delivery. The standard deviation was found to be larger in superior-inferior (SI) and anterior-posterior (AP) directions compared to the lateral (Lat) direction, both before and after treatment. Interfraction uncertainty represented by the displacement measured just before treatment with the acceptance of 2 mm residual error, was small as expected; the standard deviation was lower than 1 mm in all three directions. The intrafraction prostate movements induced a statistically significant increased standard deviation (p < 0.01) but of a moderate magnitude; the increase was 0.5, 0.6 and 0.7 mm in the Lat, SI and AP directions respectively. After treatment, the target displacement was larger than the action limit in 3.1%, 8.5% and in 5.5% of all points in the Lat, SI and AP direction respectively.
Figure 2. Frequency distribution of the prostate displacement with patient set-up using IG of target markers, measured just before treatment (a) and just after treatment (b).
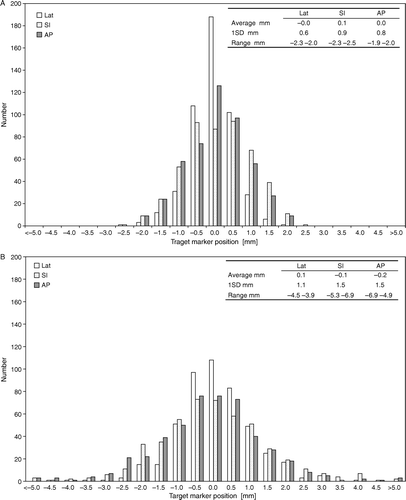
presents the systematic and random set-up uncertainties calculated according to Equation 1 and 2. Here set-up errors from patient alignment with reflecting skin markers are also included. The benefit from daily IG was confirmed, both the systematic and the random uncertainties were reduced. The standard deviation of the systematic set-up uncertainty was 1.5, 3.4 and 2.1 mm in Lat, SI and AP direction respectively with patient set-up using skin markers while considerably smaller with IG; 0.1, 0.3 and 0.5 mm respectively. However, the intrafraction prostate movements increased the set-up uncertainties; the systematic standard deviation increased with 0.4 mm in Lat and SI direction and 0.3 mm in AP direction and the random uncertainties increased with 0.4, 0.5 and 0.6 mm in Lat, SI and AP direction.
Table I. The standard deviation of geometrical uncertainties in target localization presented for three different patient alignments. ∑set-up and σset-up is the standard deviation of systematic and random treatment set-up error for all patients in all directions; lateral, superior-inferior and anterior-posterior.
Margin reduction
In , the calculated set-up margins for each direction, Lat, SI and AP are shown. Again, the results verified that the geometrical uncertainties were reduced with daily IG. The possible margin reduction was considerable when patient alignment was guided by x-ray images and target markers instead of reflecting skin markers; it was reduced with a factor larger than five. If one ignores the intrafraction uncertainties and only consider the target displacement measured at the start of the treatment, a set-up margin of 1.5 mm in all directions would be sufficient. This would be expected when the action limit was 2 mm during IG target set-up. Taking the intrafraction uncertainties into account (average beam delivery time of 9 min) an enlargement of the margin with 1 mm would be needed. However, and this has to be emphasised, this margin only includes the set-up uncertainties.
Table II. Calculated PTV margins required to account for set-up uncertainties. The margins are calculated according to 2.5∑set-up+0.7σset-up with standard deviations presented in .
Intrafraction time trends
presents the difference in prostate position before and after treatment (the intrafraction prostate movement) along with the corresponding time span between the two positions. This is equivalent to Δ target displacement measured for various time durations, denoted Δ time. The largest Δ time was 27.3 min but the major part (92%) was within 10 min. The mean duration was 7.1 min and median 6.6 min.
Figure 3. The difference in prostate displacement before and after treatment (Δ displacement) plotted against the time span (Δ time) between the two positions.
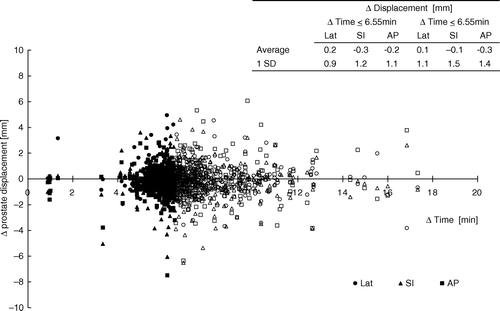
To evaluate any intrafraction time trends, the data points were divided into two groups with respect to Δ time; Δ displacement taken place within 6.6 min (median duration) and those occurring at treatment times longer than 6.6 min. A slight increase was found in the standard deviations of the two groups; 0.9, 1.2 and 1.1 mm in the Lat, SI and AP direction respectively, compared to 1.1, 1.5 and 1.4 mm in the group with treatment durations larger than 6.6 min. However, there was no statistically significant difference between the groups (p > 0.05) suggesting that the increase of 1 mm in margin accounting for intrafraction displacement is required even for shorter time duration than 6.6 min.
Discussion
The results from this study verified a substantial reduction in set-up uncertainties with IGRT and internal target markers, which is consistent with other publications Citation[1–7], Citation[14–17]. Our results implied that if IGRT is performed on daily basis, both systematic and random set-up errors will be reduced to a minimum leading to a required set-up margin of only 1.5 mm. However, this has to be supplemented with margins accounting for other geometrical uncertainties.
An important issue that needs to be considered carefully when IGRT is employed is whether the reduced margins gained from IGRT can be retained if prostate alignment is carried out only at the start of the treatment session.
We have studied intrafraction prostate motions taking place for various time durations ranging from 2 to 27 min based on target marker displacement. From the results we concluded that a margin enlargement of 1 mm is required to account for the intrafraction uncertainties. With the methodology employed in this study there was no indication of any time dependence in the intrafraction uncertainties i.e. no statistically significant increase in target displacement in treatment durations larger than 6.6 min.
Very few studies investigating intrafraction prostate motions, deal with time frames that corresponds to an IMRT delivery that typically has duration from 6 to 20 min. Nederveen et al. Citation[14] assessed intrafraction uncertainties in ten patients with fluoroscopy in time durations of 3 min and with a sampling frequency of 400 ms. They found that for most patients no intrafraction set-up margin is needed if position verification and correction are performed at intervals of 2–3 min.
Our results can be compared to the data presented by Ghilezan et al. Citation[10] which investigated prostate motions in a 1 h MRI session with the sampling interval of 6 s. The probability of displacement was calculated for time durations up to 20 min. They concluded an expected target displacement of < 3 mm within 20 min. In our results 97% of the target displacements were within 3 mm measured during the same time frame. Further, they found a strong correlation between rectum filling and prostate motions. There is a larger probability of prostate motions with a filled rectum than with an empty one. Our results were not correlated to rectum filling. Although, the patients were instructed to attain an empty rectum and bladder.
Another limitation in our methodology was that the target displacement was only monitored before and after treatment; we have no continuous sampling of the prostate motions. Thereby, sharp and quick prostate motions may have been missed.
Our conclusions with respect to margin reduction were based on the assumption that three target markers were an adequate surrogate for the CTV. When characterizing prostate motions or any distortions of the complete target volume, three markers may not be sufficient. However, intrafraction target marker pattern distortion was not detected in any treatment session. An identical marker pattern was recorded before and after treatment indicating no prostate deformation within the volume encompassed by the makers. In the majority of the patients, the initial target marker pattern remained over the course of the treatment. Interfraction target marker distortions were found in only two patients. This occurred in a limited number of the treatment sessions and was caused by a “bad” marker position. The marker was placed in the dorsal border of the prostate gland close to the rectum wall was and strongly subjected to variations in the rectum filling. These patients were further instructed to attain an empty rectum before treatment.
Finally, the suggested margin only accounts for systematic and random set-up uncertainties. The image registration of the prostate location is also attributed to uncertainties that should be considered in the design of set-up margins. Verellen et al. Citation[11] have investigated the positional accuracy of implanted markers in the Exac Trac system; the overall three-dimensional accuracy was represented by a three-dimensional displacement vector of 0.52 mm based on 2 mm CT scan data. In our study, the uncertainties associated with the IR system were neglected since it's only used to accomplish a couch movement corresponding to the displacement calculated in the x-ray system. Prior to each treatment, the displacement was determined in the x-ray system. Further, we have assessed the reproducibility (1SD) in the matching procedure by phantom measurements; 0.06 mm, 0.09 and 0.04 mm in the Lat, SI and AP directions respectively. Another uncertainty to be considered is the accuracy in defining the markers. This may have been influenced by the resolution in the CT data. However, it's our opinion that the image artefacts created by the gold markers had a larger affect than the image resolution of 0.9 mm. In this work the planned marker position relative to iso-center was represented by the midpoint in the marker volume outlined in the CT slices. We estimated the uncertainty in defining the marker midpoint to be less than 0.5 mm. Taking these uncertainties into account, the calculated margin should be 3.0, 3.6 and 3.4 mm in Lat, SI and AP direction respectively.
It is essential to point out that this margin doesn't incorporate uncertainties originated from the target delineation. However, it was not our intention to address this issue as it's not expected to be reduced with IGRT. We add a symmetrical margin of 5 mm to the CT-delineated prostate to create a PTV. Supported by the knowledge that a CT-delineated prostate volume is exaggerated compared to a MRI-delineated volume Citation[18], a margin of 5 mm is considered to be adequate in our institution.
In summary, we have investigated the set-up margin reduction achieved during IMRT of prostate cancer with daily IG using kV x-ray images and three implanted markers. Assuming that these markers enabled an accurate representation of prostate motions, we have found that a CTV-PTV margin of 1.5 mm was sufficient to account for the interfraction set-up uncertainties. However, such a narrow margin was shown to be inadequate to account for intrafraction set-up uncertainties. The target displacement was assessed before and after beam delivery in time durations up to 27 min (median 6.6 min). It was found that in order to account for intrafraction set-up uncertainties, an additional set-up margin of 1 mm was required. Our results implied that the intrafraction uncertainty was time-independent; there was no significant increase in target displacement in treatment durations longer than the median time duration. Finally, incorporating other uncertainties into the margin assessment implicated that a set-up margin of 4 mm in all direction was adequate.
References
- Litzenberg D, Balter J, Lam K, Sandler H, Haken R. Retrospective analysis of prostate cancer patients with implanted gold markers using off-line and adaptive therapy protocols. Int J Radiation Oncology Biol Phys 2005; 63: 123–133
- Chung P, Haycocks T, Brown T, Cambridge Z, Kelly V, Alasti H, et al. On-line aSi portal imaging of implanted fiducial markers for the reduction of interfraction error during conformal radiotherapy of prostate carcinoma. Int J Radiation Oncology Biol Phys 2004; 60: 329–334
- Soete G, Verellen D, Michielsen D, Vinh-Hung V, van de Steene J, van den Berge D, et al. Clinical use of stereoscopic X-ray positioning of patients treated with conformal radiotherapy for prostate cancer. Int J Radiation Oncology Biol Phys 2002; 54: 948–952
- Shimizu S, Shirato H, Kitamura K, Shinohara N, Harabayashi T, Tsukamoto T, et al. Use of an implanted marker and real-time tracking of the marker for the positioning of prostate and bladder cancers. Int J Radiation Oncology Biol Phys 2000; 48: 1591–1597
- De Boer H, van Os M, Jansen P, Heijmen B. Application of the no action level (NAL) protocol to correct for prostate motion based on electronic portal imaging of implanted markers. Int J Radiation Oncology Biol Phys 2005; 61: 969–983
- Schallenkamp J, Herman M, Kruse J, Pisansky T. Prostate position relative to pelvic bony anatomy based on intraprostatic gold markers and electronic portal imaging. Int J Radiation Oncology Biol Phys 2005; 63: 800–811
- Wu Q, Ivaldi G, Liang J, Lockman D, Yan D, Martinez A. Geometric and dosimetric evaluations of an online image guidance strategy for 3D-CRT of prostate cancer. Int J Radiation Oncology Biol Phys 2006; 64: 1596–1609
- Symon Z, Griffith K, McLaughlin W, Sullivan M, Sandler M. Dose escalation for localized prostate cancer: substantial benefit observed with 3D conformal therapy. Int J Radiation Oncology Biol Phys 2003; 57: 384–390
- Padhani A, Khoo V, Suckling J, Husband J, Leach M, Dearnaley D. Evaluating the effect of rectal distension and rectal movement on prostate gland position using cine MRI. Int J Radiation Oncology Biol Phys 1999; 44: 525–533
- Ghilezan M, Jaffray D, Siewerdsen J, van Herk M, Shetty A, Sharpe M, et al. Prostate gland motion assessed with cine-magnetic resonance imaging (cine-MRI). Int J Radiation Oncology Biol Phys 2005; 62: 406–417
- Verellen D, Soete G, Linthout N, van Acker S, De Roover P, Vinh-Hung V, et al. Quality assurance of a system for improved target localization and patient set-up that combines real-time infrared tracking and stereoscopic X-ray imaging. Radiother Oncol 2003; 67: 129–141
- Greener T “Practical determination of systematic and random set-up errors, Σset-up and σset-up using portal imaging” Publication “Geometric Uncertainties in Radiotherapy; defining the Planning Target Volume” The British Institute of Radiology, 2003.
- van Herk M, Remeijer P, Rasch C, Lebesque J. The probability of correct target dosage: dose-population histograms for deriving treatment margins in radiotherapy. Int J Radiation Oncology Biol Phys 2000; 47: 1121–1135
- Nederveen A, van Der Heide U, Dehnad H, van Moorselaar R, Hofman P, Lagendijk J. Measurements and clinical consequences of prostate motion during radiotherapy fraction. Int J Radiation Oncology Biol Phys 2002; 53: 206–214
- Ghilezan M, Yan D, Liang J, Jaffray D, Wong J, Martinez A. Online image guided intensity-modulated radiotherapy for prostate cancer: how much improvement can we expect? A theoretical assessment of clinical benefits and potential dose escalation by improving precision and accuracy of radiation delivery. Int J Radiation Oncology Biol Phys 2004; 60: 1602–1610
- Kitamura K, Shirato H, Seppenwoolde Y, Onimaru R, Oda M, Fujita K, et al. Three-dimensional intrafraction movement of prostate measured during real-time tumour-tracking radiotherapy in supine and prone treatment positions. Int J Radiation Oncology Biol Phys 2002; 53: 1117–1123
- Britton K, Takai Y, Mitsuya M, Nemoto K, Ogawa Y, Yamada S. Evaluation of inter- and intra-fraction organ motion during intensity modulated radiation therapy (IMRT) for localized prostate cancer measured by a newly developed on-board image-guided system. Radiation Medicine 2005; 23: 14–24
- Rasch C, Steenbakkers R, van Herk M. Target definition in Prostate, Head, and Neck. Semin Radiat Oncol 2005; 15: 136–145