Abstract
Spatial accuracy in extracranial radiosurgery is affected by organ motion. Motion tracking systems may be able to avoid PTV enlargement while preserving treatment times, however special attention is needed when fiducial markers are used to identify the target can move with respect to organs at risk (OARs). Ten patients treated by means of the Synchrony system were taken into account. Sparing of irradiated volume and of complication probability were estimated by calculating treatment plans with a motion tracking system (Cyberknife Synchrony, Sunnyvale, CA, USA) and a PTV-enlargement strategy for ten patients. Six patients were also evaluated for possible inaccuracy of estimation of dose to OARs due to relative movement between PTV and OAR during respiration. Dose volume histograms (DVH) and Equivalent Uniform Dose (EUD) were calculated for the organs at risk. In the cases for which the target moved closer to the OAR (three cases of six), a small but significant increase was detected in the DVH and EUD of the OAR. In three other cases no significant variation was detected. Mean reduction in PTV volume was 38% for liver cases, 44% for lung cases and 8.5% for pancreas cases. NTCP for liver reduced from 23.1 to 14.5% on average, for lung it reduced from 2.5 to 0.1% on average. Significant uncertainty may arise from the use of a motion-tracking device in determination of dose to organs at risk due to the relative motion between PTV and OAR. However, it is possible to limit this uncertainty. The breathing phase in which the OAR is closer to the PTV should be selected for planning. A full understanding of the dose distribution would only be possible by means of a complete 4D-CT representation.
The introduction into clinical practice of conformal radiotherapy and, later, of Intensity Modulated Radiation Therapy (IMRT) has allowed accurate dose conformation to be achieved, while minimizing dose delivered to surrounding organs at risk (OAR). This achievement has permitted dose escalation programs to be pursued without significant increase in risk of complications, and has also permitted to split the tumoral volume in segments to be irradiated with different dose values, often thanks to biological information given by PET or MR spectroscopy.
IMRT techniques that offer better conformity and steeper dose gradient at target periphery show a significant limitation in their use because of uncertainty in interfraction repeatability of the patient setup and to intrafraction movements, mainly due to respiration. Setup uncertainty can adequately be addressed by means of Image Guided Radiation Therapy techniques (IGRT). Intrafraction organ motion is more difficult to address. For example, use of IMRT in lung and liver lesions exposes to significant risk of geographic miss of the tumoral volume, and makes “dose painting” strategies of tumor sub-segments impossible due to respiratory movements.
Different solutions have been proposed to overcome this difficulty. Among others, two common solutions are enlargement of the Planning Target Volume (PTV) based on CT datasets acquired at end-inhale and end-exhale phases, and breath-holding or respiratory gating techniques. Both these solutions show drawbacks. PTV enlargement may require reduction of the prescribed dose in order to comply with dose constraints within OARs. Breath holding or gating techniques significantly increase treatment times with possible negative effects on the stability of patient setup and possibly affecting treatment efficacy due to low dose rate-related effects.
The problem of organ motion, mainly respiratory, presents therefore a significant impact on all phases of a high-conformity radiation treatment. In particular imaging is a key factor, whether morphological (CT) or functional (PET) in nature. A CT dataset without timing information introduces uncertainty on target and OAR location; a CT-PET dataset, due to long acquisition time, prevents correct identification of sub-volumes that might be treated with different dose prescriptions. Therefore respiratory organ motion makes dose distribution uncertain.
The Cyberknife (Accuray Inc., Sunnyvale, CA) is an image-guided radiation treatment system based on a compact linear accelerator (linac) mounted on a robotic arm Citation[1]. Image guidance and use of implanted fiducial markers allows overall targeting accuracy to be on the order of 1 mm. The Cyberknife is endowed with a motion tracking device, called Synchrony, which enables real-time tracking of target position Citation[2]. Synchrony is based on correlation between the location of internal fiducial markers sampled 20–50 times during treatment and the location (and speed) of external optical markers, tracked in real time. The linac-carrying robot is driven by the calculated law of motion of the set of internal fiducial markers, allowing respiratory tracking to be performed.
The aim of this study is the critical revision of dose distributions obtained by means of Synchrony, in particular in clinical situations where a mobile PTV is accompanied by fixed organs at risk or, in general, by organs at risk that move with respect to the PTV itself.
Material and methods
Ten patients actually treated by means of the Synchrony system have been taken into account (three liver, four lung and three pancreas cases). Prescription and fractionation schemes varied from 25 to 36 Gy at 80% in 3 fractions. For all patients two CT datasets at end inhale and end exhale condition were available. Planning for Synchrony and for the PTV enlargement technique was made on the end-exhale dataset. This choice was based on two considerations: 1) the end-exhale phase is generally more easily reproduced by the patient than end-inhale and 2) it is believed to be more representative of the real situation because it occupies a larger fraction of time than end-inhale within the (normal) respiratory cycle. Treatment margins for Synchrony were chosen equal to 2 mm based on the results of total accuracy tests that resulted in maximum error of 2 mm at the 95% confidence interval.
All plans were recalculated (but not actually delivered) using the old technique consisting in drawing the planning target volume (PTV) on both end-exhale and end-inhale datasets, and considering the join of the two contours as the actual PTV. In cases of large displacement of the two contours (two lung cases showed distance of 18 and 21 mm between centers of mass of tumor contour in end-exhale and end-inhale phases), the actual PTV was estimated as the envelope of the positions occupied in different instants of the respiratory cycle, assuming that a linear law of motion is followed. A treatment plan was then generated using the same prescription and dose constraints as the plan for Synchrony; the underlying CT dataset was end-exhale for the reasons explained above.
Reduction in PTV volume and reduction in risk of complications were estimated for the Synchrony system with respect to the PTV-enlargement technique. Risk of complications was estimated by calculating the Normal Tissue Complication Probability (NTCP) of organs at risk (OAR) for both techniques. NTCP was calculated by means of the Lyman model with correction for fractionation Citation[3]. OARs taken into account were liver, lung, oesophagus, kidney, duodenum and spinal cord. NTCP values calculated by means of the above cited model should be considered as a relative measure of dose sparing and should not be regarded in a strict sense, since they are not validated for hypofractionation. Since for some cases NTCP was already negligible with the PTV-enlargement technique, the Equivalent Uniform Dose (EUD) with correction for fractionation Citation[4] to OARs was also taken into account as an index of dose sparing. EUD was calculated aswhere Vi is the volume of the OAR receiving a dose equal to Di and φ is the volume-effect parameter, specific for the organ/tissue.
After measuring PTV and risk reduction, six of ten patients (two liver, two lung and two pancreas cases) were reconsidered for possible inaccuracies in the estimation of dose to organs at risk. In fact, using the Synchrony system implies that the dose distribution is moved accordingly to the displacement of the centre of mass of the set of fiducial markers. While the set of fiducial markers is generally representative of the position of the target, it may not be representative of the position and movement of the surrounding tissue and, in particular, of organs at risk. Dose volume histograms (DVH) and EUD were calculated from the treatment plan for Synchrony on the end-exhale condition. The dose distribution was then recalculated on the end-inhale dataset shifting the system of coordinates by a quantity corresponding to the displacement of the center of mass of the fiducial markers (in order to simulate the effect of the motion tracking system), and DVH and EUD were recalculated. The actual dose to the OAR was estimated as weighted average of the dose absorbed during inhalation and exhalation, with weights of 1/3 and 2/3, respectively. This calculation has been done after the treatment was performed; calculation for the treatment plan actually delivered was based on the end-exhale dataset. The weighted sum has been performed on a voxel-by-voxel basis; DVHs and radiobiological parameters were recalculated on the new dose distribution.
The end-inhale dataset was previously registered to the end-exhale dataset by limiting the registration to act on the spine region. Normalized mutual information was used as the similarity measure for the rigid transformation.
Finally, a test was performed to decide whether it was convenient to plan on the end-inhale or on the end-exhale dataset in order to reduce the uncertainty on the dose to OARs. Equivalent plans were generated on the two datasets separately, then the obtained dose distribution was transported on the other dataset and the DVH to OARs was recalculated.
Results
Volume of the PTVs as drawn on the double CT technique ranged from 127 to 143 cm3 (liver), from 22 to 360 cm3 (lung) and from 31 to 35 cm3 (pancreas). Mean reduction in PTV volume was 38% for liver cases, 44% for lung cases and 8.5% for pancreas cases. NTCP for liver reduced from 23.1 to 14.5% on average, for lung it reduced from 2.5 to 0.1% on average.
reports the results on the variation of EUD.
Table I. EUD corrected for fractionation for different organs at risk, calculated on a plan relative to the PTV enlargement technique (Join of targets) and with the respiratory tracking system (Synchrony).
reports target volumes and EUD corrected for fractionation for the 10 cases, relative to the organs at risk liver, lung and esophagus for the PTV enlargement and the motion tracking system.
Table II. Target volume and EUD corrected for fractionation for the 10 cases, relative to the PTV enlargement and the motion tracking system.
As for the analysis of possible inaccuracies in the estimation of dose to organs at risk, the cases for which the target moved closer to the OAR during inhalation (three cases of six) showed a small but significant increase in the DVH and EUD of the OAR. In other three cases no significant variation was detected. shows axial and coronal slices on which the dose distribution resulting from planning on full-exhalation (left) and from estimation in full-inhalation (right) is displayed. In this lung case the maximum dose to the spinal cord increased from 0.74 Gy to 1.1 Gy on full inhalation and to 0.82 Gy as weighted average. Despite these dose values are not clinically relevant, the relative increase was 49% and 11%, respectively, which may become a problem in situations where the dose to the organ at risk is closer to the constraint value.
Figure 1. Dose distribution calculated on full-exhalation (left) and estimated on full inhalation (right).
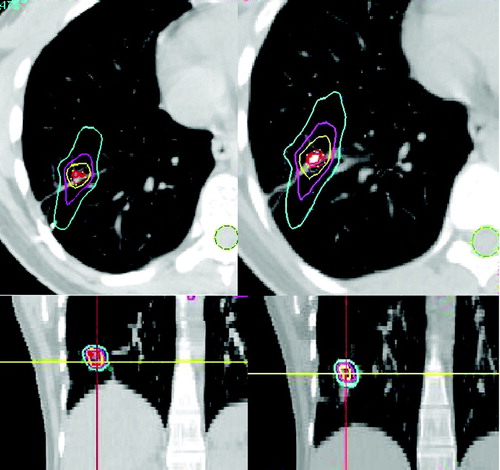
The test to decide whether it was convenient to plan on end-inhale or end-exhale is directly related to the result above. Anyway, even without recalculating NTCP and EUD it was possible to estimate what dataset was best suited for planning. In particular, the closer proximity of OARs to the PTV shall be considered, and the corresponding dataset shall be chosen for planning. In fact this condition assures that the inaccuracy in dose estimation to the OAR shall reveal itself as overestimation, thus representing a safety condition.
Discussion and conclusion
As expected, smaller reduction in PTV volume and smaller uncertainty in the calculation of dose to OARs were estimated for cases that show smaller displacement due to respiration, i.e. pancreas cases.
Use of the end-exhale CT dataset has been suggested for treatment planning because it is the most reproducible phase and because it represents the longer time interval within the respiratory cycle Citation[5], Citation[6]. However, when a motion tracking system is employed, care should be taken in order to assess what dataset (i.e. full inhalation or full exhalation) better represents a safety condition. A general suggestion could be to acquire both ends of the respiratory cycle and to use for planning the dataset that shows the closest proximity of the OAR to the target. This choice may be difficult or impossible if more than one OAR exists, possibly moving with respect to the PTV with different trajectories.
Inhomogeneity correction algorithms may play an important role in the accuracy of dose calculation. In principle, the choice of which phase is optimal for treatment planning may depend not only on locations of OARs and target, but also on electron scatter with different lung fillings. However, the treatment planning software of the Cyberknife system does not use any refined dose inhomogeneity correction such as pencil beam or collapsed cone convolution, but is based on density scaling within the lung region. For this reason this issue was not taken into account in the decision process of which CT phase should be used for planning.
For the above mentioned reasons, the dose distribution obtained by means of Synchrony may not be representative of the actual situation. In particular, when OARs move with respect to the PTV the choice of the CT dataset to use for planning may be insufficient to guarantee accuracy of dose calculation within acceptable limits (e.g., within 5%).
Full timing information may be provided by time-related CT datasets, also referred to as 4DCT. Important studies exist in the literature (see for example Citation[5]) that should help using this information in the treatment planning process. A foreseeable development of current treatment planning systems regards use of this information to solve difficult problems like the presence of more than one OAR that move with respect to each other. It must be noted, however, that even 4DCT gives limited information on breathing motion. Significant uncertainty may arise from inter- and intra-fraction variation of the breathing pattern, as well as variations between imaging and treatment sessions. Patient cooperation appears to be crucial when reproducibility of the breathing pattern has to be achieved.
References
- Adler JR, Murphy MJ, Chang SD, Hancock SL. Image-guided robotic radiosurgery. Neurosurgery 1999; 44: 1299–306
- Schweikard A, Shiomi H, Adler JR. Respiration tracking in radiosurgery. Med Phys 2004; 31: 2738–41
- Lyman JT. Complication probability as assessed from dose volume histograms. Radiat Res 1985; 104: 513–9
- Niemierko A. A. generalized concept of generalized uniform dose (Abstract). Med Phys 1999; 26: 1100
- Zhang T, Orton NP, Tomé WA. On the automated definition of mobile target volumes from 4D-CT images for stereotactic body radiotherapy. Med Phys 2005; 32: 3493–502
- Seppenwoolde Y, Shirato H, Kitamura K, Shimizu S, Herk M, van Lebesque JV, et al. Precise and real-time measurement of 3D tumor motion in lung due to breathing and heartbeat, measured during radiotherapy. Int J Radiat Oncol Biol Phys 2002; 53: 822–34