Abstract
The aim of this study was to evaluate the uncertainty of patient immobilization within the Elekta body frame (SBF) used for stereotactic body radiotherapy (SBRT) and to suggest margins sufficient to ensure dose coverage to the gross target volume (GTV). The study was based on the evaluation of repeated CT-scans of 30 patients treated by SBRT. The overall uncertainty was divided between uncertainty related to internal movement of the tumor and uncertainty in the patient set-up. Standard deviations of the overall tumor displacement were 2 mm, 3 mm and 4 mm in medial-lateral (m-l), anterior-posterior (a-p), and cranio-caudal (c-c) directions, respectively. In a model based on the data, an ellipsoid planned target volume (PTV) corresponding to the standard deviations in the orthogonal directions and a scaling factor, K defined a 3-dimentional (3-D) probability density. According to the model, a 90% probability of full dose coverage of the GTV was secured using margins of 9 mm (m-l), 9 mm (a-p) and 13 mm (c-c), respectively. The overall uncertainty was dominated by internal tumor movements whereas the set-up uncertainty of the patient in the SBF was less pronounced. It was concluded that the Elekta SBF is useful for immobilisation of patients for SBRT. However, due to internal movement conventional margins of 5 mm in m-l and a-p and 10 mm in the c-c directions may be insufficient for full dose coverage.
Stereotactic body radiotherapy is used to treat primary and secondary tumors of the thorax and abdomen. It is based on principles of stereotactical treatment of brain tumors Citation[1]. The treatment technique differs from traditional radiotherapy by the use of complex field arrangements and by its hypofractionated nature. It is used in the treatment of small volumes with tight margins and SBRT dose plans are characterized by steep dose gradients. High accuracy of the target localisation is therefore of major importance Citation[2]. SBRT involves the use of the SBF for immobilisation defining an external coordinate system in which the tumor can be localised and treated Citation[3–7]. The margins used for treatment are dependent on the internal movement of the tumor and the accuracy of the patient set-up Citation[8].
The aim of this study is to investigate these uncertainties in SBRT based on patient immobilization in the Elekta SBF.
Materials and methods
This study was based on the treatments of 30 patients of whom eight were treated for secondary liver tumors and 22 for primary or secondary lungs tumors. A total of 40 tumors were included in this analysis. Of these, 17 were located in the liver and 23 in the lung. All patients were immobilized by use of the Elekta SBF with a vacuum cushion Citation[1]. Two invasive skin marks (tattoos) on the sternum and one on under each knee and laser based alignment secured a fixed rotation and cranio-caudal position of the patient. The patients were CT scanned between two and four times with at least 1 day between each scan. Intravascular contrast in the form of 100 ml Visipaque with an iodine content of 270 mg/ml was given to all patients with liver tumors. The 69 CT studies performed on a Siemens Somatom as spiral scans with a couch movement of 10 mm/s and a reconstruction slice distance of 4.0 mm. In addition, a topogram was recorded for each CT session. The first CT study served as the basis for the dose plan whereas the subsequent CT studies were used to verify the localisation of the tumor and vertebral spine within the SBF.
It was assumed that the tumor movements between CT studies in the principal directions were independent and normal distributed. Intrafraction movement was not taken into account. All tumors were delineated by the same investigator using the HELAX-TMS dose planning system (version 6.1 B).
For each tumor, a test dose plan was created with three orthogonal fields from the principal directions and an isocentre located centrally within the tumor. The jaws were subsequently set to the tumor outline and these settings together with the known isocentre position were used to calculate the position of the tumor centre of mass within the coordinate system of the SBF. A total of 95 tumor positions were registered.
An internal reference point was defined on each scan by the vertebral spine present in the same cranio-caudal position as the tumor. The displacement of the internal reference point between the dose planning scan and each of the subsequent scans was determined.
Displacement of the internal reference point included displacement in the three principal directions as well as rotation along the longitudinal axis. The cranio-caudal displacement of the internal reference point was determined by the CT topogram. The displacement of the tumor was determined by subtraction of the coordinates of the tumor centre of mass in the dose planning CT study and each of the subsequent scans. Overall tumor displacement and displacement of the internal reference point were defined as difference in the position of the tumor or the internal reference point relative to the external coordinate system of the SBF. Displacement of the tumor due to internal movement (internal tumor displacement) was calculated by subtraction of position coordinates of the tumor and the internal reference system. For calculation of standard deviation Citation[9] of the tumor movement and the displacement of the internal reference point, see Appendix Appendix 1.
A 3-D distribution of displacements of an ellipsoid shape with axis lengths proportional to the corresponding general standard deviations of the total tumor movement was assumed. The ellipsoid represents an iso-probability density surfaces for a 3-D distribution of tumor reposition in the SBF coordinate system. The probability, p for full dose coverage of the tumor depends on the scaling factor, K. The theoretical relation between p and K was found by integration in Appendix Appendix 2.
Results
Results of the analysis are given in and . Standard deviations of overall displacement of the tumor were 2.4 mm, 2.6 mm and 3.6 mm in the l-r, a-p and c-c plane, respectively. The overall displacement in the c-c plane was larger than in the l-r and the a-p planes. For coverage probabilities between 50 and 90%, the related margins are given in . Aiming a coverage probability of 90%, the margins should be 8.6 mm, 9.3 mm and 13.0 mm in the l-r, a-p and c-c direction, respectively. With standard margins of 5 mm (l-r), 5 mm (a-p) and 10 mm (c-c) Citation[4], the target would only be fully covered in 49% of the cases included in the present analysis.
Figure 1. Fitted and empirical probability distribution of overall displacements of 95 tumor positions.
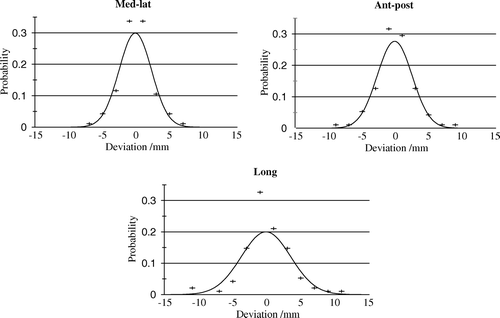
Table I. Standard deviations for overall tumor- internal reference point- and internal tumor displacements measured in millimetres for the medial/lateral (m-l), anterior/posterior (a-p), and cranio-caudal (c-c) directions. Ranges are specified in brackets.
Table II. Probability of dose coverage of the PTV and related margins.
The internal tumor displacement was considerably larger than the displacement of the reference point, meaning that internal movement of the tumor contributed to a greater extend to the overall displacement compared to the displacement caused by set-up error. No significant difference of internal tumor movement was found between lung and liver tumors. The ellipsoid representing an iso-probability density surfaces for a 3-D distribution of tumor reposition in the SBF coordinate system is given in . With K-values of 1 and 3, the probability of having full dose coverage is 6 and 72%, respectively. The theoretical relationship between K and probability of coverage, p is given in . This figure also gives the observed frequency of full dose coverage of tumors in relation to the K-value. For K-values in the interval 0–4, there was a complete agreement between observed and modelled probability. For higher K-values, there was a low observed probability compared to the fitted curve suggesting a number of out-layers. No obvious characteristics of patients representing this part of the curve were observed.
Discussion
Precise immobilization is of outmost importance in SBRT. Due to the small number of high dose fractions and steep dose gradients, not only systematic errors are important. Random errors may be of high importance as well. The Elekta SBF is the most frequently used system for immobilization. It includes an abdominal pressure that reduces the respiration related internal movement of targets in the basal part of the lung and in the liver and an external reference coordinate system used for alignment of the patient in the treatment position. Other groups have performed studies of the reproducibility of patient positioning based on the Elekta SBF. In general, these studies show a good reproducibility of the system allowing the use of small margins of 5 – 10 mm covering the GTV Citation[2], Citation[10–12]. We have found that the standard margin were too small a number of tumors and in the present material we were not able point out groups of tumors that are in risk of being missed due to displacement. No difference in target displacement was found between liver and lung tumors.
Some uncertainty in methodology was related with the present analysis. Variation in delineation of the target and the vertebral spine was included in the estimation of uncertainty in position of the target and the internal reference point. Since delineation of the tumor may be uncertain, this type of study tended to overestimate the internal target displacement compared to the displacement in the reference point. This was especially a problem in liver tumors since imaging of a liver tumor is less precise Citation[13]. Furthermore, breath related internal movement of the tumor introduced an uncertainty in target positioning that could not be determined by the present method based on static CT-scans. Only 4-D CT-scan can depict the effect of respiration on target positioning. So far, this effect has not been taken into account in SBRT.
The present study introduced a model combining an iso-density probability for dose coverage with a scaling factor, K. Based on standard deviations of target positions on consecutive CT-scans it was possible to estimate margins around the GTV sufficient to ensure a specified probability of dose coverage. The model also allowed the judgement of how dose coverage was changed by any change in size of the margin.
The reproducibility of overall target positioning was divided into the reproducibility of internal target positioning related to internal movement and reproducibility related to the positioning of the body in the SBF. We found that the internal target movement was the major contribution to the overall tumor displacement between different scans. The set-up uncertainty including rotation in the SBF of the internal reference point was of minor importance. This showed that the SBF is highly efficient in immobilization of the body or the internal reference point. However, internal movement of the target may be more pronounced that expected and described previously Citation[2], Citation[10–12]. Therefore, introduction of new techniques that may improve the accuracy related to internal movement is highly warranted. Novel respiratory gating technique and on-line imaging guidance may be ways to diminish the uncertainty related with internal movement Citation[10], Citation[11], Citation[14].
It has been shown in a number of studies that SBRT without respiratory gating results in high probability of local control Citation[1], Citation[6], Citation[11], Citation[15]. The high local control rate suggests the use of overkill radiation doses in SBRT. Respiration related target motion may result in blurring of the radiation dose outside the PTV. This may explain the general high local control despite the relatively low frequency of full dose coverage of 49% of tumors as found in the present study. Further escalation of the dose has been proposed and the RTOG group now use a central dose of 20 Gy with 95% isodose encompassing the PTV in order to diminish the risk of local recurrence. However, it is unclear whether the relatively few recurrences are caused by geographical misses or caused by use of low prescribed dose to the target and further escalation of dose may not be needed.
In the present study, estimates of uncertainties of tumor and reference centre were given in a pooled analysis of lung and liver tumors. We found no significant difference in accuracy of positioning between tumors in the two organs, but we are also aware that the power of the study to show such difference is not sufficient. Future sufficiently powered studies may focus on this subject and even differentiate between tumors in different location in an organ Citation[15].
In conclusion, we found that internal movement of the target contributed to the major part of the overall uncertainty in tumor positioning in SBRT. Uncertainty in patient set-up was of less importance. The use of standard margins of 5/10 mm resulted in relatively poor dose coverage of the PTV. The high local control observed in a number of studies on SBRT can be explained by respiratory motion related dose blurring effect of the periphery of the target.
References
- Blomgren H, Lax I, Näslund I, Svanström R. Stereotactic high dose fraction radiation therapy of extracranial tumors using an accelerator. Acta Oncol 1995; 34: 861–70
- Wulf J, Hädinger U, Oppitz U, Thiele W, Flentje M. Impact of target reproducibility on tumor dose in stereotactic radiotherapy of targets in the lung and liver. Radiother Oncol 2003; 66: 141–50
- Herfarth K, Debus J, Lohr F, Bahner M, Fritz P, Höss A, et al. Extracranial stereotactic radiation therapy: Set-up accuracy of patients treated for liver metastases. Int J Radiat Oncol Biol Phys 2000; 46: 329–35
- Hoyer M, Roed H, Hansen A, Ohlhuis L, Petersen J, Nellemann H, et al. Prospective study on stereotactic radiotherapy of limited stage non-small cell lung cancer. Int J Radiat Oncol Biol Phys 2005; 63: S396
- Lax I, Blomgren H, Larson D, Näslund I. Extracranial stereotactic radiosurgery of localized targets. J Radiosurg 1998; 1: 135–48
- Lax I, Blomgren H, Näslund I, Svanström R. Stereotactic radiotherapy of malignancies in the abdomen. Acta Oncol 1994; 33: 677–83
- Wulf J, Hädinger U, Oppitz U, Olshausen B, Flentje M. Stereotactic radiotherapy of extracranial targets: CT-simulation and accuracy of treatment in the stereotactic body frame. Radiother Oncol 2000; 57: 225–36
- Herk M, Remeijer P, Rasch C, Lebesque J. The probability of correct target dosage: Dose-population histograms for deriving treatment margins in radiotherapy. Int J Radiat Oncol Biol Phys 2000; 47: 1121–35
- Harris W, Kratochvil B. An introduction to chemical analysis. Philadelphia:Saunders College Publishing; 1980.
- Eccles C, Brock K, Bissonnette JP, Hawkins M, Dawson L. Reproducibility of liver position using active breathing coordinator for liver cancer radiotherapy. Int J Radiat Oncol Biol Phys 2006; 64: 751–9
- Hara R, Itami J, Kondo T, Aruga T, Abe Y, Ito M, et al. Stereotactic single high dose irradiation of lung tumors under respiratory gating. Radiother Oncol 2002; 63: 159–63
- Hurkmans C, Remeijer P, Lebesque J, Mijnheer B. Set-up verification using portal imaging; review of current clinical practice. Radiother Oncol 2001; 58: 105–20
- Giraud P, Elles S, Helfre S, Rycke YD, Servois V, Carette MF, et al. Conformal radiotherapy for lung cancer: Different delineations of the gross tumor volume (GTV) by radiologists and radiation oncologists. Radiother Oncol 2000; 62: 27–36
- Jin JY, Ajlouni M, Chen Q, Yin FF, Movsas B. A technique of using gated-CT images to determine Internal Target Volume (ITV) for fractionated stereotactic lung radiotherapy. Radiother Oncol 2006; 78: 177–84
- Seppenwoolde Y, Shirato H, Kitamura K, Shimizu S, Herk M, Lebesque J, et al. Precise and real-time measurement of 3D tumor motion in lung due to breathing and heartbeat, measured during radiotherapy. Int J Radiat Oncol Biol Phys 2002; 53: 822–34
Appendix 1
Under the adapted assumptions the general standard deviations for the stochastic variables describing the movement can be derived. For each tumor t, the positions is determined n(t) times. The estimate of the deviation of the x component of the tumor position is calculated aswhere xit is x position of the t'th tumor for the i'th CT study and
is the mean x position of the t'th tumor. Estimate of the deviation in the x direction is obtained by combining the S(x)t of all tumors:
where M is the total number of tumors studied Citation[9]. Similar expressions exist for the y and z directions.
Appendix 2
The theoretical relation between the probability for dose coverage of the tumor, p and the scaling factor, K is derived by numerical integration.Where the volume integral is performed over an ellipsoid given by
For a given value of p and K margins used to form the PTV can be calculated from
Where σx, σy, and σz are the general standard deviations.