Abstract
To compare finite-size pencil beam/equivalent path-length (FSPB/EPL) and Monte Carlo (MC) SBRT dose computations for serial tomotherapy and to quantitatively assess dose differences between the dose calculation methods. Based on 72 SBRT plans for pulmonary targets, FSPB/EPL, considering the inhomogeneous lung environment, and MC calculations were performed to establish differences between FSPB/EPL predicted dose and MC derived doses. Compared with MC, FSPB/EPL consistently overestimated minimum doses to the clinical target volume and planning target volumes by an average of 18.1±7.15% (range 4 to 33.4%), and 21.9±10.4% (range 1.2 to 45.5%), respectively. The respective mean target dose differences were 15.5±7.4% (2.8–36.4%) and 19.2±7.6% (3.6–40.1%). Deviations from MC doses were lesion size and location dependent, with smaller lesions completely embedded into lung parenchyma being most susceptible. Larger lesion in contact with mediastinum and chest wall showed lesser differences. In comparison with MC dose calculation, FSPB/EPL overestimates doses delivered to pulmonary SBRT targets. The observed dose differences may have impact on local tumor control rates, and may deserve consideration when using fast, but less accurate dose calculation methods.
Stereotactic body radiation therapy (SBRT) for malignant pulmonary tumors is an effective treatment concept in which high doses of radiation are delivered to a small tumor in a single or few radiation fractions under stereotactic localization techniques. Of significant interest to the SBRT community continues to be the determination of optimal prescription doses. A comprehensive phase 1 dose escalation trial has established safe total and single fraction doses for “SBRT” of primary lung neoplasms Citation[1]. Also, a dose response relationship for the probability of local tumor control has been reported Citation[2]. In order to compare SBRT plans and outcomes from multiple institutions a better understanding of the true delivered radiation dose is needed. Thus, a more accurate dosimetry in the inhomogeneous and low-density environment of the lung is required.
Correction based algorithms used in conventional treatment planning systems assume charge particle equilibrium and do not account appropriately for changes in electron transport when calculating dose in heterogeneous lung tissue. Errors in dose calculation in lung have been shown to exceed 20% for small targets such as the ones typically selected for SBRT treatment Citation[3–9]. Monte Carlo (MC) dose calculation codes are now widely regarded as the most accurate means of radiation transport and dose calculation Citation[10], Citation[11]. However, there is only sparse data in the application of MC calculations for SBRT treatment planning and delivery Citation[4], Citation[7].
This study attempts to accurately characterize SBRT doses given to a large sample of pulmonary targets using a serial tomotherapeutic intensity-modulated delivery method (Nomos Peacock, Nomos, Cranberry Township, PA). Monte Carlo calculated doses were compared to the Finite Sized Pencil Beam (FSPB) /Effective Path Length (EPL) algorithm as implemented in the CORVUS 5.0 planning system.
Materials
SBRT plans of 63 patients with 72 lung lesions (72 individual treatment plans) previously treated by SBRT for primary and secondary lung malignancies generated using a planning system inherent FSBP/EPL dose calculation algorithm were recalculated with Monte Carlo. Doses calculated with Monte Carlo were used as a benchmark to evaluate doses calculated by the FSPB/EPL algorithm.
The original clinically used inverse SBRT plans generated by the Corvus 5.0 optimizer (Nomos, Cranberry Township, PA) were copied, anonymized for study purposes and recalculated by FSPB/EPL. The details of SBRT planning and delivery at UTHSCSA/CTRC are reported in detail in a separate paper in this issue (Fuss et al. Tomotherapeutic SBRT). Relevant to this comparison analysis is predominantly the generation of the PTV which was typically an expansion of the CTV by 5 mm axially and 10 mm cranio-caudally. PTV margins beyond the CTV consist of normal tissues, in the present study mostly low-density lung tissue. However, 61 lesions expressed some degree of overlap between PTV margins and tissue density such as the mediastinum or chest wall. Doses prescribed ranged between 3×12 Gy for lung metastases, and 3×20 Gy for stage I NSCLC. Doses were prescribed so that 95% of the PTV was exposed to the prescribed dose. We aimed for a heterogeneous dose prescription with maximum doses within the CTV of about 150% of PD. Doses were delivered using a 6 MV linear accelerator with an attached binary multi-leaf collimator proprietary to the Nomos serial tomotherapy system.
Dose calculation in Corvus 5.0
Corvus 5.0, like many other commercial inverse treatment planning systems for intensity-modulated radiation therapy, employs a FSPB algorithm approach for fast but approximate dose calculation. Tissue heterogeneity correction was considered during optimization final dose calculation. For dose planning of targets embedded into the low-density lung environment, the inhomogeneity correction used is the Effective Path Length Method (EPL). Continuous annealing was used for the generation of all patient plans in this study. Subsequently, this plan was submitted for Monte Carlo dose calculation, hence planned intensity maps and monitor units are identical for both sets of SBRT plans. It is important to note that this study did not take motion into account in order to isolate the dosimetric differences between the algorithms.
Monte Carlo (PEREGRINE 1.6 b)
Monte Carlo recalculation utilized a commercially available platform, PEREGRINE 1.6b (Nomos, Cranberry Township, PA). Details of the underlying Monte Carlo code and dose model as well as validations of the resulting dosimetry have been published in detail Citation[10], Citation[11].
For MC dose recalculations in the present study, plans generated using FSPP were submitted to Peregrine for calculation of the dose contribution of individual pencil beams and their associated beam intensity.
Prior to conduct of the present study, the Peregrine MC dose model for use with serial tomotherapy and beam delivery via a binary multi-leaf collimator (MIMiC, Nomos, Cranberry Township, PA) was validated. For validations in homogeneous phantoms, calculations agreed to better than 2% or 2 mm to measurements. Calculations agreed to better than 3% in inhomogeneous phantoms containing lung, air and bone material (data not shown), and were thus consistent with prior established performance of the system Citation[11].
Plan analysis
We collected GTV/CTV and PTV volumes. Also the location of targets was assessed, with separation into lesions embedded into lung parenchyma and lesions in contact with soft-tissue structures such as chest wall or mediastinum. Mean, minimum and maximum dose for the targets and critical structures were compared. We report dose differences between MC, and EPL calculated plans as percentage difference in dose from the MC plan, which was considered the benchmark for this comparison.
Statistical analysis was performed using JMP v6 (SAS Institute, Cary, NS). In order to define volumetric cutpoints associated with increased EPL calculated-dose deviance from the MC method, bivariate recursive partitioning (RP) was utilized Citation[12]. To this end, two levels of radiation dose differential were defined: a 10% difference between EPL and MC calculated doses, a dose difference documented to yield clinically detectable outcome differences Citation[13], and 20%, a dose difference that separated favorable and less favorable outcomes in 2 SBRT series Citation[1], Citation[2], Citation[14].
Since, at present, validation data for the MC calculation we utilized has not been subjected to peer-review, we utilized Altman-Bland analysis designed to determine agreement between FSPB/EPL and MC in the absence of a validated standard calculation technique Citation[15]. Systematic bias estimations, equivalent to the mean difference between MC and EPL dose calculations, were derived. The 95% limit of agreement (LOA) is the range within which 95% of all differences between measures may be predicted to occur. The 95% LOA represents the range by which two techniques may be expected to agree 95% of the time, and allows clinicians to determine the degree of interchangeability between two distinct calculation techniques. In the context of the analyzed data, a 5% dose range was defined to represent a clinically acceptable agreement between dose calculation methods.
Results
Target volumes
Average CTV and PTV volumes were 27.2 cm3 (range 0.3 to 142.8 cm3), and 72.3 cm3 (range 5.4 to 256.2 cm3), respectively. The CTV and PTV of eleven lesions were completely embedded into lung tissue. Average CTV and PTV volumes of these lesions was 4.5 cm3 (range 0.3 to 16.4 cm3), and 21.1 cm3 (range 6.1 to 55.6 cm3). Free lesions were statistically significantly smaller than lesions where either the CTV or the corresponding PTV had soft tissue contact (p = 0.013). Lesions in contact with soft tissues were on average 31.5 cm3 (range 0.3 to 142.8 cm3, CTV), with PTV volumes of 80.5 cm3 (range 5.4 to 256.2 cm3).
Target dose exposure
All plans revealed lower minimal, maximal and mean doses for MC compared with FSPB/EPL plans. Differences, or dose loss between FSPB/EPL predicted and MC calculated minimum, maximum and mean CTV dose was 18.1±7.2% (range 4 to 33.4%), 13.4±9% (range 1.1 to 41.64%), and 15.5±7.4% (range 2.8 to 36.4%), respectively. Differences in minimal and mean doses were larger for smaller lesions fully embedded into lung parenchyma compared with other lesions (mean 25.4±5.7% versus 17±7%, p < 0.001; and mean 24.1±67.3% versus 13.9±6.2%, p < 0.001).
Similarly, differences between FSPB/EPL predicted and MC calculated minimum, maximum and mean PTV dose was 21.9±10.4% (range 1.2 to 45.5%), 17.5±13.2% (range 0.7 to 56.3%), and 19.2±7.6% (range 3.6 to 40.1%), respectively. As observed for dose differences to the CTV, differences in minimal and mean doses were larger for smaller lesions fully embedded into lung parenchyma compared with other lesions (mean 30.3±8.4% versus 20.3±10.1%; p = 0.003; and mean 29.9±6.1% versus 17.2±6.2%, p < 0.001).
Volume dependency of dose differences between EPL and MC
Logarithmic regression revealed a statistically significant negative correlation between MC-EPL percent differential and target volume in cm3. documents results from bivariate logarithmic regression analysis. The corresponding adjusted R2 values for minimum, maximum and mean CTV dose versus volumes of CTVs were 0.16, 0.12, and 0.21, respectively. The respective adjusted R2 values for the PTVs were 0.2, 0.15, and 0.24.
Figure 1. Logarithmic regression analysis display of differences in minimum, maximum, and mean clinical target volume (CTV) and planning target volume (PTV) doses between finite size pencil beam/equivalent path length (EPL) and Monte Carlo (MC) dose calculation methods (y-axis), and volumes of targets (x-axis). Bold circles represent lesions embedded into lung, open circles indicate lesions with either direct CTV soft tissue contact or PTV soft tissue overlap.
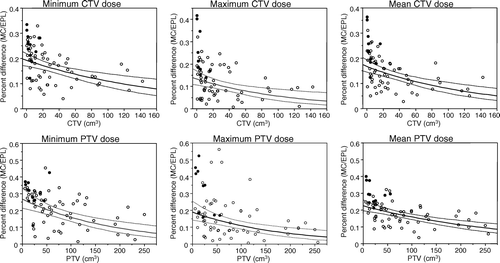
Figure 2. Altman Bland plots of 95% limits of agreement between finite size pencil beam/equivalent path length (EPL) and Monte Carlo (MC) dose calculation methods.
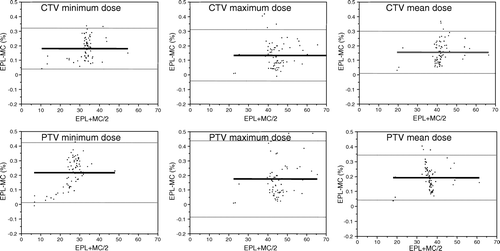
RPA established natural volumetric cutpoints are summarized in ; the specified values represent reasonable estimates of useful volume thresholds, the crossing of which may significantly alter the proportion of plans whose EPL calculated dose differs substantially from the MC by a 10% or 20% dose difference. All cut points were statistically significant at p < 0.05, but the cutpoint established for CTV mean dose and a dose differential of 10% (p = 0.06), and PTV minimum dose for this dose difference level (p = 0.07).
Table I. Recursive portioning analysis derived target volume cut points for dose differences of 10% or more and 20% or more between finite size pencil beam/equivalent path length (FSPB/EPL) and Monte Carlo (MC) based dose predictions.
Agreement between dose calculation methods
According to Altman Bland analysis, FSPB/EPL and MC based dose prediction expressed limits of agreement ranges of between 15% and 26%, 95% of the time (, ). Thus, a 5% dose range agreement between dose calculation techniques was exceeded. Under the hypothesis that MC more closely approximated true delivered dosimetry, this allows to derive that differences between predictions by the dose calculations methods employed may have clinical significance.
Table II. Altman-Bland analysis summary; mean method differential and 95% limits of agreement (LOA) are shown for both total calculated dose and percent of total dose. The clinically accepted dose range 95% LOA of 5% was routinely exceeded.
Discussion
The relevance of this report of Monte Carlo dose recalculations for serial tomotherapy SBRT plans for pulmonary targets is based on preliminary model calculations of dose loss in the periphery of small target volumes when using pencil beam based dose calculations Citation[3], Citation[4], Citation[7], Citation[16]. The clinical importance of this report is given by observations of dose dependent outcomes in SBRT, with dose differences between excellent and marginal outcomes of about 20% Citation[1], Citation[2], Citation[14]. Thus, any difference between the treatment planning system predicted and actually delivered radiation doses may have impact on the probability of local tumor control.
Differences between prescribed and delivered dose may vary between treatment planning systems. It is well understood, that dose predictions or calculations by different dose calculation methods may deviate from measurable doses when the target is embedded into the low-density environment of the lung Citation[3], Citation[4], Citation[16]. The absence of iterative calculations and the limited number of radiation ports used in 3D-conformal based SBRT requires little computation time, allowing the use of sophisticated algorithms like the convolution-superposition method. However, for IMRT planning, the number of field segments or pencil beams can be very large, necessitating the use of faster and somewhat simplified dose calculation algorithms. The very fast pencil beam calculation and EPL heterogeneity correction method used, among other treatment planning systems, by the Corvus inverse planning system, has the advantage that it can be embedded in the optimization routine, allowing the optimizer to consider lung inhomogeneity as it develops the optimal plan. The trade off is the use of a simpler and less accurate tissue inhomogeneity model. The use of very small pencil beams, in the present study 8.5×10 mm, creates additional dose calculation challenges as the fluence and dose profile over each pencil beam differs from larger field dosimetry Citation[5]. Monte Carlo calculations more closely approach the realistically deliverable dosimetry without being a perfect surrogate, still the MC calculated doses distributions were used as a benchmark in this comparison.
As reported in the literature Citation[3], Citation[4], Citation[6–9], Citation[11], Citation[17], FSPB/EPL dose calculations or prediction overestimated the minimum, maximum and mean dose to clinical target volumes when compared with more accurate dose calculations. Thus, a lesser dose than prescribed may be delivered to pulmonary target volumes.
In the present study, comparing FSPB/EPL with MC, distinct dose prediction differences between methods were observed. Compared with MC, FSPB/EPL routinely overestimated the minimum and mean target doses by between 15 and 22%. Smaller lesions embedded into lung parenchyma were most susceptible with differences between FSPB/EPL predicted and MC doses of over 25%. Volumetric cut points provided by RPA analysis indicate that lesions smaller than 17 and 25.6 cm3 (roughly lesions with a diameter of 2.5 to 3 cm and smaller) were more susceptible to 20% or higher dose differences in mean and minimum target dose. The clinical relevance of these findings is based on preliminary validation work that supports the assumption that MC calculations more closely approximate the true delivered dosimetry, thus lower doses than prescribed were likely clinically delivered in the actual patient cohort.
In a subset of cases, realistically delivered absolute doses appear equally low or even lower than doses found to result in local failures as reported by Wulf et al., where total doses of 30 Gy in 3 fractions in resulted in a local control rate of 71% compared with a local control rate of 93% when 36 Gy or 37.5 Gy in 3 fractions was delivered Citation[2]. Also, at the 3×16 Gy and lower dose levels in the NSCLC Indiana dose escalation trial, nine local failures were observed, whereas a single tumor failed treatment at doses of 3×20 Gy or higher Citation[1], Citation[14]. As of today, no inferior outcomes have been observed in the cohort of patients whose plans were recalculated for the present study. In fact, one of the principal aims of the study, an attempted correlation of more realistic dose deposition with local tumor control, was not possible because of the low incidence of local failures. The few local failures were observed in patients with larger tumors, and thus tumors in which the deposited dose was more accurately predicted. Thus, the clinical relevance of the findings in the present study remains unclear at present. In the context of SBRT planning and delivery, a multitude of factors may contribute to tumor control and failure, with the accuracy of the dose calculation algorithm employed being just one component to be considered. Relevant parameters potentially also contributing to favorable outcomes may include tumor histology, simulation imaging and subsequent target volume delineation, dose prescription concept, and means and frequency of image-guidance employed. It is conceivable, that any of the above mentioned parameters may have similar or even higher impact on ultimate outcomes. Thus, despite the fact that delivered SBRT doses in the present series likely differed from the prescribed doses in the multitude of patients, favorable outcomes suggest not to deviate from the present dose prescription practice. At the same time, we suggest caution when using other pencil beam based dose calculation algorithms and more homogeneous SBRT dose prescriptions; the heterogeneous dose prescription strategy pursued in the present patient cohort may have afforded actual dose delivery beyond the threshold for favorable outcomes.
The key conclusion of the present research is a strong suggestion to report not only tumor related parameters such as location and volumes along with dose prescription concepts and dose scheduling but also the means by which dose calculations were performed. Reporting this critical information will allow to more closely and accurately comparing doses and outcomes between institutions and may in the future allow to better approximate dose response relationships for both SBRT tumor control and associated normal tissue complications.
References
- Timmerman R, Papiez L, McGarry R, Likes L, DesRosiers C, Frost S, et al. Extracranial stereotactic radioablation: results of a phase I study in medically inoperable stage I non-small cell lung cancer. Chest 2003; 124: 1946–1955
- Wulf J, Baier K, Mueller G, Flentje M. Dose-response in stereotactic irradiation of lung tumors. Radiother Oncol 2005; 77: 83–87
- Traberg Hansen A, Petersen JB, Hoyer M, Christensen JJ. Comparison of two dose calculation methods applied to extracranial stereotactic radiotherapy treatment planning. Radiother Oncol 2005; 77: 96–98
- Haedinger U, Krieger T, Flentje M, Wulf J. Influence of calculation model on dose distribution in stereotactic radiotherapy for pulmonary targets. Int J Radiat Oncol Biol Phys 2005; 61: 239–249
- Jones AO, Das IJ. Comparison of inhomogeneity correction algorithms in small photon fields. Med Phys 2005; 32: 766–776
- Carrasco P, Jornet N, Duch MA, Weber L, Ginjaume M, Eudaldo T, et al. Comparison of dose calculation algorithms in phantoms with lung equivalent heterogeneities under conditions of lateral electronic disequilibrium. Med Phys 2004; 31: 2899–2911
- Fujisaki T, Kikuchi K, Saitoh H, Tohyama N, Myojoyama A, Osawa A, et al. Effects of density changes in the chest on lung stereotactic radiotherapy. Radiat Med 2004; 22: 233–238
- Krieger T, Sauer OA. Monte Carlo- versus pencil-beam-/collapsed-cone-dose calculation in a heterogeneous multi-layer phantom. Phys Med Biol 2005; 50: 859–868
- Mesbahi A, Allahverdi M, Gheraati H. Monte carlo dose calculations in conventional thorax fields for 60Co photons. Radiat Med 2005; 23: 341–350
- Hartmann Siantar CL, Walling RS, Daly TP, Faddegon B, Albright N, Bergstrom P, et al. Description and dosimetric verification of the PEREGRINE Monte Carlo dose calculation system for photon beams incident on a water phantom. Med Phys 2001; 28: 1322–1337
- Heath E, Seuntjens J, Sheikh-Bagheri D. Dosimetric evaluation of the clinical implementation of the first commercial IMRT Monte Carlo treatment planning system at 6 MV. Med Phys 2004; 31: 2771–2779
- Cook EF, Goldman L. Empiric comparison of multivariate analytic techniques: advantages and disadvantages of recursive partitioning analysis. J Chronic Dis 1984; 37: 721–731
- Dutreix A. When and how can we improve precision in radiotherapy?. Radiother Oncol 1984; 2: 275–292
- McGarry RC, Papiez L, Williams M, Whitford T, Timmerman RD. Stereotactic body radiation therapy of early-stage non-small-cell lung carcinoma: phase I study. Int J Radiat Oncol Biol Phys 2005; 63: 1010–1015
- Bland JM, Altman DG. Statistical methods for assessing agreement between two methods of clinical measurement. Lancet 1986; 1: 307–310
- Jeraj R, Keall PJ, Siebers JV. The effect of dose calculation accuracy on inverse treatment planning. Phys Med Biol 2002; 47: 391–407
- Pawlicki T, Ma CM. Monte Carlo simulation for MLC-based intensity-modulated radiotherapy. Med Dosim 2001; 26: 157–168