Abstract
Aim. To investigate the direct relationship between tumour hypoxia and lactate dehydrogenase (Ldh) levels in serum and tumour in two different pre-clinical murine models. Materials and methods. Experiments were performed in CDF1 or C3H/Km mice implanted with a C3H mammary carcinoma and SCCVII squamous cell carcinoma, respectively. Low oxygen breathing for 1–72 h was used to increase tumour hypoxia. Ldh activity was measured in the serum and tumour cytosole with a colorimetric method. Tumour Ldha mRNA levels were assessed with RT-PCR. Results. The serum Ldh in non-tumour bearing CDF1 mice and C3H/km mice was 10.5±2 U/ml and 12±2 U/ml, respectively. For C3H mammary carcinoma bearing mice, a positive correlation between tumour volume and tumour and serum Ldh was found. Tumour Ldh in SCCVII carcinomas also increased with increasing tumour volume, but no volume dependence of serum Ldh was found. Low oxygen breathing caused a 2–3 fold increase in tumour Ldha mRNA in both tumour models. In C3H mammary carcinoma bearing mice, serum and tumour Ldh significantly increased after 48 and 72 hours of hypoxia, respectively. Low oxygen breathing did not change serum and tumour Ldh in SCCVII carcinoma bearing mice. Reoxygenation for 4 or 24 hours had no additional effect on Ldh activity in any of the models. Discussion. Serum Ldh activity can be a marker for tumour burden in certain types of cancer. The relationship between serum and tumour Ldh and tumour hypoxia has not been confirmed. However, Ldha mRNA may be a potential new marker of tumour hypoxia and should be further investigated.
Alteration of glucose metabolism is a distinct feature of cancer cells. Most of the ATP is normally produced through oxidative phosphorylation. Malignant cells, however, exhibit a high rate of glycolysis Citation[1]. This “glycolytic phenotype” may represent a selective advantage for cancer cells especially in hypoxic conditions.
The molecular mechanism of a high rate of glycolysis has been studied and lactate dehydrogenase (Ldh) was found to play a key role Citation[2]. Lactate dehydrogenase is a tetrameric enzyme with five isoforms (LDH1-LDH5) resulting from a combination of two subunits: LDHA (also known as LDH-1 or LDH-M) and LDHB (also known as LDH-2 or LDH-H). LDH5 (4 LDHA subunits) catalyzes the conversion of pyruvate to lactate, whereas LDH1 (4 LDHB subunits) kinetically favours oxidative phosphorylation. Koukourakis et al. previously showed that LDH5 is the isoenzyme that is predominantly expressed in cancer cells of different malignant tumors Citation[3]. Furthermore, tumour LDH5 expression was found to be associated with activated VEGF/VEGRFR2 pathway Citation[4] and vascular density Citation[5]. Thus, LDH5 can be a marker of aggressive cancer disease. Accordingly, tumour LDH5 expression has a negative prognostic value in patients with colorectal Citation[5] and endometrial carcinoma Citation[4]. Previously, Shim et al. used antisense Ldha in c-myc transformed fibroblasts and showed a significant growth reduction in hypoxic conditions Citation[6]. The observed Ldha dependency for tumour proliferation both in vitro and in vivo conditions has further been proved by Fantin et al. Citation[2]. These findings provide evidence that LDHA is not only a marker of cancer aggressiveness, but also directly involved in cancer promotion and thus a potential therapeutic target.
Tumour hypoxia is a common feature of tumours Citation[7]. The presence of tumour hypoxia is clinically relevant because it promotes cancer disease Citation[8] and induces resistance to radiation therapy Citation[9]. One of the molecular mechanisms of hypoxia induced cancer promotion is transcriptional activation of HIF-1-regulated genes whose protein products are involved in angiogenesis, energy metabolism, cell proliferation and survival Citation[10]. Several genomic approaches have been used to identify hypoxia responsive gene expression profiles. Approximately 1–1.5% of the genome is transcriptionally responsive to hypoxia Citation[11], but there is a significant heterogeneity in hypoxia response between different cell lines. LDHA belongs to genes regulated by HIF-1. A uniform hypoxia induced LDHA expression among six different cellular compartments of tumours has recently been reported Citation[11]. Furthermore, a positive correlation between HIF-1 and LDH5 staining was found in patients operated for non-small cell lung cancer Citation[12] and colorectal cancer Citation[13]. In the above mentioned studies the positive LDH5 staining of tumours was linked to poor prognosis of patients. These findings indirectly suggest tumour hypoxia as an underlying mechanism for cancer aggressiveness.
In the present study we asked whether there is a causal relationship between tumour hypoxia and Ldh. We used two mouse tumour models that were different with regard to hypoxia and necrosis, which allowed us to evaluate the variability of Ldh and hypoxia stress response. First, we measured serum Ldh, tumour Ldh, and tumour Ldha mRNA in mice with different sized tumours. Then, we studied the impact of both acute and prolonged hypoxic stress and reoxygenation.
Materials and methods
Animal and tumour models
The transplanted C3H/Tif mammary carcinoma and SCCVII (C3H/St) squamous cell carcinoma grown in the right rear feet of female CDF1 (C3D2F1: C3H/HeNBomTac female×DBA/2JBomTac male) and C3H/Km mice, respectively, were used. Experiments were carried out in mice with 0–800 mm3 sized tumours. Tumour volume was calculated from the three orthogonal diameters (D values) using the formula: D1×D2×D3×π/6. All experiments were performed under national and European Union approved guidelines for animal welfare.
Serum and tumour tissue samples
A 500 µl-blood sample was obtained from the suborbital sinus of nonanesthetised mice. The samples were centrifuged at 1 500 g for 10 min within 30 min of collection, and the serum stored at −80°C until analysis. Immediately after collection, the mice were killed by cervical dislocation, and the tumours were dissected and fresh frozen. Tumour cytosol extracts were homogenized at 4°C in a phosphate buffer using a rotary homogeniser, followed by centrifugation at 10.000 g for 10 min.
Ldh analysis
Ldh activities were measured in extracted mouse blood serum and tumour cytosol using a colorimetric assay. Briefly, the substrate solution was prepared by dissolving 2.42 g of Tris in 50 ml distilled water, adding 0.975 ml 60% L-lactic acid solution and adjusted to pH 8.2 with 1 M HCl. The colour reagent was prepared by dissolving 100 mg NBT, 100 mg NAD and 10 mg PMS in 20 ml distilled water. Sixteen µl of serum or tumour cytosol were added to 160 µl of substrate and warmed to 30°C for 15 min. Then 32 µl of colour reagent were added and mixed. After 3 min the reaction was terminated with 80 µl of 1 M HCl. Absorbance was recorded at 595 nm against a blank in which water replaced serum or cytosol samples. Ldh activity was then calculated from standard curves with bovine heart Ldh serving as standard. All reagents were purchased from Sigma-Aldrich (Brøndby, Denmark).
Real time, quantitative PCR
Frozen tumour tissues were homogenized and total RNA was extracted using an RNeasy kit (Qiagen, Valencia, CA). Total RNA was treated with DNase I, and reverse transcribed using High capacity cDNA Archive Kit (Applied Biosystems, Foster City, CA). The resulting cDNA was used as a template for PCR. All reactions were done in triplicate. Data analysis was performed using the comparative Ct method. The expression level of Ldh was normalized to the household gene Tfrc, as previously described Citation[14]. Equal amounts of total RNA was used to generate cDNA, and judged by the Ct values, Tfrc was expressed at similar levels in the two tumour models.
Hypoxia and reoxygenation treatments
Mice with 200 mm3 tumours were placed in a gas chamber and exposed to a continuous flow of 10% oxygen (balance nitrogen, flow rate 1.33 l/min) for 1, 3, 6, 24, 48 or 72 hours as previously described Citation[15]. Mice were then returned to ambient conditions and blood samples for serum Ldh analysis taken. Some mice were exposed to hypoxia for 12 hours as described previously and then either blood samples taken immediately or the mice were returned to ambient conditions for 4 or 24 hours before taking blood samples.
Statistical analysis
Statistical analysis was performed using SPSS 10.0 for Windows analytical software. Statistical significance was determined using the two-tailed Student‘s t test and p values less than 0.05 were considered significant. Correlations between ordinals were assessed using regression analysis.
Results
The mean serum Ldh activities in non-tumour bearing CDF1 and C3H/Km mice were 10.5±1.7 U/ml and 12±2 U/ml, respectively. shows the relationship between Ldh activity and tumour volume. For C3H mammary carcinomas, serum Ldh increased to 129±5 U/ml and 234±10 U/ml in mice with 50 mm3 and 700 mm3 sized tumours, respectively. Serum Ldh in C3H/Km mice transplanted with SCCVII carcinomas was higher (ranging from 27 to 39 U/ml) compared to non-tumour bearing mice, but there was no volume dependence. Tumour Ldh in 200 mm3 sized C3H mammary carcinomas was 66±7 U/mg protein and this was 2.6 fold lower than Ldh activity in similarly sized SCCVII carcinomas (171±11 U/mg protein). Tumour Ldh increased with increasing tumour volume reaching 85±4 U/mg protein (p = 0.003) and 247±23 U/mg protein (p = 0.015) in respective 700 mm3 sized C3H mammary carcinomas and SCCVII carcinomas.
Figure 1. Serum (left panel) and tumour (right panel) lactate dehydrogenase activity from either female CDF1 (•) or C3H mice (o) were measured using a colorimetric assay. Measurements were made in mice implanted with differently sized C3H mammary (CDF1 mice) or SCCVII (C3H mice) carcinomas. Individual results are shown, with lines fitted by regression analysis.
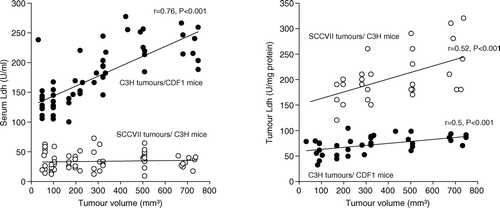
Ldha mRNA levels are presented relative to the level of the household gene, Tfrc Citation[14], normalized to the ratio under ambient conditions, and log2 transformed (). Comparisons of the expression levels of Ldha mRNA in the two tumour models under ambient conditions suggests that the baseline expression levels of Ldha mRNA might be 4-10 fold lower in C3H mammary carcinomas compared to SCCVII carcinomas (data not shown). Low oxygen breathing significantly increased Ldha mRNA levels in both tumour models (). For C3H mammary carcinomas, there was a 1.6 fold increase in Ldha mRNA already at 1 hour of hypoxia (p = 0.03) with a maximal response (2.8 fold) at 48 hours of hypoxia (p = 0.01). However, tumour Ldh significantly decreased from the baseline value of 94±1 U/mg protein to 77±3 U/mg protein at 1 hour of hypoxia (p = 0.005) and thereafter slowly increased to 113±1 U/mg protein at 48 hours (p < 0.001, compared to controls). Serum Ldh showed a similar trend as for tumour Ldh, but a significant change was only seen at 72 hours (172±14 U/ml, controls vs. 231±16 U/ml, p = 0.01). No change in serum Ldh in non tumour bearing CDF1 mice at 72 hours of hypoxia was found (data not shown). For SCCVII carcinomas, the Ldha mRNA level maximally increased at 6 hours of hypoxia (2.5 fold, p = 0.01), and after 24 hours slowly decreased. No change in tumour and serum Ldh in SCCVII carcinoma bearing mice was found in this hypoxia experiment.
Figure 2. Fold difference in serum (▾) and tumour LDH activity (o), and Ldha mRNA expression (•) in 200 mm3 C3H mammary carcinoma (left panel) and SCCVII carcinoma (right panel) bearing mice treated with 10% oxygen for 1–72 hours compared to controls. Data represent mean ±s.e.m. (n = 10 for each group for serum LDH, n = 4–5 for each group for tumour LDH, n = 3–4 for each group for Ldha mRNA measurement; *p < 0.05, **p < 0.001 compared to control).
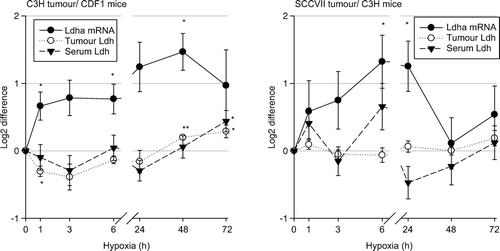
Low oxygen breathing for 12 hours increased Ldha mRNA in both tumour models in the same manner as previously described, but serum and tumour Ldh remained unchanged. Reoxygenation for 4 hours or 24 hours had no additional effect on Ldha mRNA, tumour and serum Ldh in any of the mouse/tumour models ().
Figure 3. Fold difference in serum (black bars) and tumour LDH activity (gray bars), and Ldha mRNA expression (dark gray bars) in 200 mm3 C3H mammary carcinoma (left panel) and SCCVII carcinoma (right panel) bearing mice treated with 10% oxygen for 12 hours and then reoxygenated for 0, 4 or 24 h when compared to controls. Bars represent means ±s.e.m. (n = 7–9 for each group; *p < 0.05, **p < 0.001 compared to control).
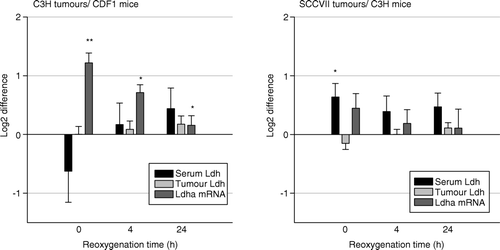
Discussion
Serum Ldh activity and tumour LDH5 expression has been found to be associated with aggressive cancer disease and poor patient outcome Citation[5]. Tumour hypoxia as an underlying mechanism for cancer aggressiveness has recently been proposed Citation[12], Citation[13]. In the present study, we investigated the impact of tumour hypoxia on Ldh in two different mouse tumour models.
Our results show a positive correlation between tumour volume and Ldh activity in both murine tumours. Furthermore, in C3H mammary carcinoma bearing mice we found a positive correlation between tumour and serum Ldh activity, which suggests the secretion of tumour Ldh into the blood (data not shown). This was not the case in SCCVII carcinoma bearing mice, where serum Ldh was independent of tumour volume. The relationship between tumour and serum Ldh has never been fully understood. Koukourakis et al. reported a correlation between the serum Ldh levels and the percentage of cancer cells with positive LDH5 reactivity in non-small-cell lung cancer tissue and colorectal adenocarcinomas Citation[5], Citation[12]. However, serum Ldh failed to reflect tissue expression in 71% of LDH5 positive adenocarcinomas. The lack of association may, however, be explained by different methods of Ldh measurement used; serum Ldh levels are expressed as total enzyme activity while estimates of tumour LDH5 status involve positive immunohistochemical staining. Ldh is typically released from necrotic cells. The tumour models used in this study were different with regard to necrosis. In 200 mm3 sized C3H mammary carcinomas the necrotic fraction ranges from 10–30% Citation[16] and as we have previously shown the number of necrotic cells increases with tumour size Citation[17]. On the other hand, less than 1% of tumour cells in SCCVII carcinoma are necrotic Citation[16]. Thus, tumour necrosis may play a role in the volume dependent increase of serum Ldh in C3H mammary carcinoma bearing mice. The C3H mammary carcinoma and SCCVII carcinoma also differed in respect to the levels of tumour hypoxia. For the C3H mammary carcinoma the radiobiological hypoxic fraction in 200 mm3 tumours has been estimated to be around 15% Citation[18]. It has also been shown that in this tumour model the number of hypoxic cells increases with increasing tumour volume Citation[17], which follows the tumour size dependent changes for serum and tumour Ldh (). For 200 mm3 SCCVII carcinoma the radiobiological hypoxic fraction is less than 1% Citation[19] but results from other groups suggest that at larger sizes it is increased Citation[20], again consisted with the tumour Ldh changes we found.
In the current study, we found that the baseline expression of Ldha mRNA in 200 mm3 SCCVII carcinomas was 4 to 10 fold higher than in similar sized C3H mammary carcinomas, which is consistent with the observed differences in tumour Ldh activity. However, this baseline Ldha mRNA expression is inconsistent with the radiobiological hypoxic fractions in these two tumour types. In a previous study, we exposed both C3H mammary carcinoma and SCCVII carcinoma bearing mice to low oxygen breathing for 1–72 hours Citation[15], and showed that tumour hypoxia (estimated with the Eppendorf electrode) significantly increased after 3 hours in both tumour models and remained elevated during the whole gassing period. Exposing tumour bearing mice to similar conditions in our current study resulted in similar and significant increases in Ldha mRNA expression in both tumour types within 6 hours, and a maximum (2.5 to 2.8 fold difference at 24–48 hours of hypoxia. Tumour hypoxia is known to exist in acute or chronic form. The rapid response (1–6 hours) is consistent with previously reported in vitro findings suggesting Ldha as a marker for acute rather than chronic hypoxia Citation[14], Citation[21]. Hypoxia induced Ldha expression is mediated via HIF-1α. The HIF-1 binding site has been identified and located to the promoter of the Ldha gene Citation[22]. Consistently, tumour LDH5 expression was found to be directly associated with HIF-1α staining in colorectal carcinomas Citation[13].
The general cellular response to hypoxia is the down regulation of high energy processes that involve a decrease of overall rate of protein synthesis, which occurs initially at the level of translation Citation[23]. However, some genes affecting cell survival, angiogenesis and the switch to a metabolic profile of hypoxic cells are selectively expressed and translated during hypoxia. The exact molecular mechanism for selective gene translation is just starting to be understood (for review, see Citation[24]). The presence of positive and negative regulatory elements within the 5′and 3′ untranslated regions of a particular mRNA has been suggested to influence the efficiency of translation. However, a large variability in the control of translation among different tumours is to be expected because of a frequent disruption of up-stream signalling pathways in cancer cells. In our experiment with C3H mammary carcinomas, tumour and serum Ldh did not change in the first 24 hours of hypoxia but significantly increased at 48 and 72 hours. This is consistent with the up-regulation of Ldha (maximum response at 48 hours) and supports the suggestion of Ldha being also selectively translated under hypoxia in this tumour model. The analysis of polysome mRNA from tumours exposed to hypoxia may be needed to provide more information about the level of Ldha mRNA translation. Previously, White et al. performed experiments with ischemia and reperfusion in rabbit myocardium to investigate ischemia-specific changes at the molecular levels Citation[25]. They found a significant increase of Ldh measured in myocardial tissue during ischemia, which is in accordance with our findings. It should be noted, that prolonged hypoxia can increase tumour necrosis Citation[15] and therefore contribute to increased serum Ldh levels. But, the fact that tumour Ldh increased in accordance with serum Ldh in our experiment disproves this suggestion. Our findings seem to indicate that stress-induced Ldh production rather than cell necrosis plays a role in the cellular release of Ldh. The observation that tumour and serum Ldh in SCCVII tumour bearing mice (despite Ldha mRNA induction) did not change during hypoxia, points to tumour specific variability of Ldha translation. Similarly, Blancher et al. previously reported cell line specific variability in the LDHA activity measured in the medium after 16 h of hypoxia Citation[21]. Induction of LDHA activity varied from 1.0 to 1.6 fold in six different breast cancer cell lines. Finally, reoxygenation had no additional effect on Ldh in any of the tumour models used.
In conclusion, using two different mouse tumour models we demonstrated an association between tumour Ldh and tumour burden, which also fits with hypoxic fraction estimates in these tumours. However, secretion of Ldh into the blood appears to be tumour specific suggesting that measurement of serum Ldh has no role as a marker for hypoxia. There was also no relationship between basal Ldha mRNA levels and radiobiological hypoxia in these two tumour models. However, there were significant increases in Ldha mRNA when the animals were exposed to changes in hypoxia. This suggests that although serum and tumour Ldh and Ldha mRNA may not be useful for measuring hypoxia per se, the latter may have the potential to monitor changes in tumour hypoxia. Clearly, further research is needed to confirm this suggestion.
Acknowledgements
The authors would like to thank Ms I. M. Horsman, Ms B. Hermansen, Ms P. Schjerbeck and Ms D. Grand for excellent technical help. This study was supported by a grant from the Danish Cancer Society.
References
- Warburg O. On the origin of cancer cells. Science 1956; 123(3191)309–14
- Fantin VR, St Pierre J, Leder P. Attenuation of LDH-A expression uncovers a link between glycolysis, mitochondrial physiology, and tumor maintenance. Cancer Cell 2006; 9: 425–34
- Koukourakis MI, Giatromanolaki A, Sivridis E. Lactate dehydrogenase isoenzymes 1 and 5: Differential expression by neoplastic and stromal cells in non-small cell lung cancer and other epithelial malignant tumors. Tumour Biol 2003; 24: 199–202
- Giatromanolaki A, Sivridis E, Gatter KC, Turley H, Harris AL, Koukourakis MI. Lactate dehydrogenase 5 (LDH-5) expression in endometrial cancer relates to the activated VEGF/VEGFR2(KDR) pathway and prognosis. Gynecol Oncol 2006; 103: 912–8
- Koukourakis MI, Giatromanolaki A, Sivridis E, Gatter KC, Harris AL. Lactate dehydrogenase 5 expression in operable colorectal cancer: Strong association with survival and activated vascular endothelial growth factor pathway--a report of the Tumour Angiogenesis Research Group. J Clin Oncol 2006; 24: 4301–8
- Shim H, Dolde C, Lewis BC, Wu CS, Dang G, Jungmann RA, et al. c-Myc transactivation of LDH-A: Implications for tumor metabolism and growth. Proc Natl Acad Sci USA 1997; 94: 6658–63
- Vaupel P, Kallinowski F, Okunieff. Blood flow, oxygen and nutrient supply, and metabolic microenvironment of human tumors: A review. Cancer Res 1989; 49: 6449–65
- Hockel M, Schlenger K, Aral B, Mitze M, Schaffer U, Vaupel P. Association between tumor hypoxia and malignant progression in advanced cancer of the uterine cervix. Cancer Res 1996; 56: 4509–15
- Nordsmark M, Overgaard M, Overgaard J. Pretreatment oxygenation predicts radiation response in advanced squamous cell carcinoma of the head and neck. Radiother Oncol 1996; 41: 31–9
- Semenza GL. HIF-1:. Mediator of physiological and pathophysiological responses to hypoxia. J Appl Physiol 2000; 88: 1474–80
- Denko NC, Fontana LA, Hudson KM, Sutphin PD, Raychaudhuri S, Altman R, et al. Investigating hypoxic tumor physiology through gene expression patterns. Oncogene 2003; 22: 5907–14
- Koukourakis MI, Giatromanolaki A, Sivridis E, Bougioukas G, Didilis V, Gatter KC, et al. Lactate dehydrogenase-5 (LDH-5) overexpression in non-small-cell lung cancer tissues is linked to tumour hypoxia, angiogenic factor production and poor prognosis. Br J Cancer 2003; 89: 877–85
- Koukourakis MI, Giatromanolaki A, Simopoulos C, Polychronidis A, Sivridis E. Lactate dehydrogenase 5 (LDH5) relates to up-regulated hypoxia inducible factor pathway and metastasis in colorectal cancer. Clin Exp Metastasis 2005; 22: 25–30
- Sørensen BS, Hao J, Overgaard J, Vorum H, Honore B, Alsner J, et al. Influence of oxygen concentration and pH on expression of hypoxia induced genes. Radiother Oncol 2005; 76: 187–93
- Lukacova S, Overgaard J, Alsner J, Horsman MR. Strain and tumour specific variations in the effect of hypoxia on osteopontin levels in experimental models. Radiother Oncol 2006; 80: 165–71
- Sorensen M, Horsman MR, Cumming P, Munk OL, Keiding S. Effect of intratumoral heterogeneity in oxygenation status on FMISO PET, autoradiography, and electrode Po2 measurements in murine tumors. Int J Radiat Oncol Biol Phys 2005; 62: 854–61
- Lukacova S, Khalil AA, Overgaard J, Alsner J, Horsman MR. Relationship between radiobiological hypoxia in a C3H mouse mammary carcinoma and osteopontin levels in mouse serum. Int J Radiat Biol 2006; 81: 937–44
- Raleigh JA, Chou SC, Arteel GE, Horsman MR. Comparisons among pimonidazole binding, oxygen electrode measurements, and radiation response in C3H mouse tumors. Radiat Res 1999; 151: 580–9
- Horsman MR, Khalil AA, Siemann DW, Grau C, Hill SA, Lynch EM, et al. Relationship between radiobiological hypoxia in tumors and electrode measurements of tumor oxygenation. Int J Radiat Oncol Biol Phys 1994; 29: 439–42
- Murata R, Shibamoto Y, Sasai K, Oya N, Shibata T, Takagi T, et al. Reoxygenation after single irradiation in rodent tumors of different types and sizes. Int J Radiat Oncol Biol Phys 1996; 34: 859–65
- Blancher C, Moore JW, Talks KL, Houlbrook S, Harris AL. Relationship of hypoxia-inducible factor (HIF)-1alpha and HIF-2alpha expression to vascular endothelial growth factor induction and hypoxia survival in human breast cancer cell lines. Cancer Res 2000; 60: 7106–13
- Firth JD, Ebert BL, Ratcliffe PJ. Hypoxic regulation of lactate dehydrogenase A. Interaction between hypoxia-inducible factor 1 and cAMP response elements. J Biol Chem 1995; 270: 21021–7
- Connolly E, Braunstein S, Formenti S, Schneider RJ. Hypoxia inhibits protein synthesis through a 4E-BP1 and elongation factor 2 kinase pathway controlled by mTOR and uncoupled in breast cancer cells. Mol Cell Biol 2006; 26: 3955–65
- van den Beucken T, Koritzinsky M, Wouters BG. Translational control of gene expression during hypoxia. Cancer Biol Ther 2006; 5: 749–55
- White MY, Tchen AS, McCarron HC, Hambly BD, Jeremy RW, Cordwell SJ. Proteomics of ischemia and reperfusion injuries in rabbit myocardium with and without intervention by an oxygen-free radical scavenger. Proteomics 2006; 6: 6221–33