Abstract
High-energy external radiotherapy has become one of the most common treatment in localized prostate cancer. We compared the difference of dose distribution, mainly at the 5–30 Gy dose level, in the irradiated pelvic volume among three modalities of radiotherapy for patients with prostate cancer: conventional, conformal and intensity-modulated radiotherapy (IMRT). We selected six patients with prostate cancer treated by conformal radiotherapy at the doses of 46 Gy to PTVN (prostate and seminal vesicles), and 70 Gy to PTV-T (prostate). The conventional technique": an 8-field arrangement was used; the conformal technique 4 fields with a boost through 6 fields. For IMRT, a five-beam arrangement was used. Dose-volume histograms (DVH) were analyzed and compared among the three techniques. The IMRT technique significantly increased the pelvic volume covered by the isodose surfaces below 15 Gy as compared with the conventional and conformal techniques. The mean absolute increase for the pelvic volume included between 5–30 Gy for the IMRT technique, was about 2 900 ml as compared with the conventional technique. However, IMRT significantly reduced the irradiated volume of the rectum in the dose range of 5 to 40 Gy, also significantly reduced the irradiated volume of bladder and femoral heads, and obtained a similar or improved isodose distribution in the PTVs. In addition, the use of IMRT slightly increased the relative dose delivered to the body volume outside the pelvis, as estimated by the use of specific software. A long-term follow-up will be needed to evaluate potential late treatment complications related to the use of IMRT and the low or moderate irradiation dose level obtained in the pelvis and in the whole body.
Prostate cancer is among the most common solid malignancies. External-beam radiation therapy (EBRT) together with radical prostatectomy is the most common treatment used for early prostate cancer Citation[1]. The goal of EBRT is to deliver a uniform absorbed dose to the tumor volume while sparing surrounding normal tissues as much as possible. EBRT has very much changed during the past years: from 2-dimensional (2D) and 3-dimensional (3D) conventional techniques, to 3D conformal techniques, and then to intensity-modulated radiotherapy (IMRT). Conformal radiotherapy and IMRT are more and more widely used.
The conventional (beam arrangement) technique consists of a 4-beam arrangement (known as “box” technique) with 2 antero-posterior opposed beams and 2 lateral opposed beams. The 3D conformal technique involving 4 and then 6-field beam arrangements is often used in prostate cancer at the Institut Gustave-Roussy (IGR). This technique includes 2 phases: (1) a “box” technique, followed by (2) three sets of opposed fields (2 pairs of oblique and 2 lateral beams). The shape of treatment portals is based on the beam's-eye view projection of the target volume.
Recent data show the importance of dose escalation in the treatment of localized prostate cancer. Pollack et al. Citation[2] reported results of a phase III study evaluating dose escalation in patients who were randomized between a total dose of 70 Gy (4-beam arrangement “box” technique) or 78 Gy (including a “box” technique, and a six-field conformal field boost arrangement). The 78 Gy arm showed a statistically significant increased time-free from tumor failure. Lebesque et al. Citation[3] reported a multi-centre randomized phase III trial comparing 68 Gy with 78 Gy in 669 stage T1b – T4N0M0 prostate cancer patients, the failure-free rate at 5 years was significantly better in the 78 Gy-arm compared with the 68 Gy-arm (64% vs. 54%, p = 0.025) Citation[3]. Radiation dose escalation increases toxicity, particularly rectal complications, mainly when delivered with conventional techniques.
Three-dimensional conformal radiotherapy techniques allow an improved overall treatment tolerance for higher doses, as these techniques adapt the high radiation dose volume to the shape of the tumor, reducing the volume of normal tissues exposed to a high-dose level. However, the complication rates, particularly rectal bleeding, may remain substantial. Several analyses Citation[4], Citation[5] suggest that late rectal complications correlate with the volume of rectal wall exposed to a dose higher than 60–70 Gy when given with conventional fractionation. Therefore, the implementation of IMRT, with the potential to further reduce the rectal dose, should be considered to reduce toxicity, as reported by Zelefsky et al. Citation[6]. IMRT may reduce the dose to selected normal tissue structures in an effort to preserve function, while maintaining full dose delivery to tumor targets. Prostate cancer has served as a model for the implementation of IMRT Citation[7], Citation[8]. As indicated by a growing body of experience, IMRT for prostate cancer may represent a major technologic and clinical advance for treatment, by improving dose distribution and reducing the overall treatment toxicity profile.
On the other hand, late complication rates, such as the induction of secondary cancer, have been found to be increased among long-term survivors after cancer treatment Citation[9–13]. Radiotherapy is among the main causes of treatment associated second malignancies. Two recent studies based on the Surveillance, Epidemiology, and End Results (SEER)-Medicare database have suggested a significant increase in development of bladder and colo-rectal cancer, after radiotherapy for prostate cancer Citation[14], Citation[15]. With the use of modern techniques of radiotherapy, such as IMRT to reduce acute toxicity and short-term complications, we need to estimate and evaluate the related late complications in long-term survivors. From this point of view, a theoretical disadvantage of IMRT is the increase of the irradiated volume in the low-dose range because of the use of multiple fields.
The three analyzed techniques (conventional, conformal and IMRT) may differ quite substantially between different centers. In this study, we define the conventional technique as a 3-D conformal technique by shaping the treatment portals based on the beam-eye view (BEV) projection of the target, Regarding IMRT, inversely planned dynamic MLC technique with 20 MV photons has been used in our center. We focused our study on the evaluation of dose distribution by comparing differences in the irradiated whole pelvis with the three external beam radiotherapy modalities as used at the Institut Gustave-Roussy.
Materials and methods
Six patients with localized prostate cancer (with different forms or volumes of prostate and /or seminal vesicles) treated by conformal radiotherapy at the IGR were selected and planned concomitantly by three techniques: a conventional 3-D beam arrangement (with shape of the treatment portals based on the BEV projection of the target using the information of the CT scan), conformal (4 and then 6-field beam arrangements) and IMRT (5 fields).
Computed tomography (CT) scan technique
For each patient, a CT-scan was performed in the treatment position, by exploring 29.7 cm of the pelvic region which is the volume of interest in this study; the central slice was chosen 10 cm under the umbilicus. All slices had a thickness and spacing of 3 mm, and 99 slices were done in total. Antero-posterior and lateral scout films were obtained to ensure that the pubic symphysis was aligned with the central axis.
Volume delineation and dose prescription
The volumes of the target and the organs at risk (OAR), such as rectum, bladder, and femoral heads were delineated by the same physician to maintain consistency in volume definition. The target volumes were defined as the clinical target volume CTV-N for the prostate and the seminal vesicles, and CTV-T for the prostate only. According to current practice at the IGR, for PTVs a margin of 9 mm around the CTVs was added toward the superior, inferior, and anterior directions, while posteriorly, the margin was 6 mm. The PTV volume range was 107–255 cm2 for PTV-N and 65–194 cm2 for PTV-T. A further margin of 9 mm was used to take into account the penumbra of the multi-leaf collimator, and this margin was defined at the center-of-leaves. The same margin in all projections will possibly either overdose or underdose the PTV, as the contribution from other fields in AP and lateral direction is not a similar contribution in superior or inferior direction using coplanar technique. For a high quality of conformal radiotherapy the margin differ some millimeters in the different directions in order to obtain a good quality index. In our study, we simplified this margin and used the same in all directions and for all three techniques to well compare the isodose distributions.
Regarding the contouring technique, the rectum was defined as the portion of the organ corresponding to the level of the prostate and seminal vesicles and extending inferiorly to the anus, and superiorly to 3 CT slices beyond CTVs. The entire bladder and the femoral heads up to the small trochanters were delineated. Bladder and rectum were delineated as solid organs including the thickness of the rectal or bladder wall. The pelvis volume was defined as the part of the body explored by the CT-scan (29.7 cm for each patient).
The studied body segment was automatically outlined by the Somavision system (Varian), and the beam's set up was defined by medical physicists and radiation oncologists within the same system. The same prescription dose was used for the three compared techniques. Dose distribution for the three techniques was calculated by the Varian CadPlan-Helios treatment plan system in order to obtain a nearly equal dose volume covering of PTVs. PTV-N volume percentage to 45 Gy: 98.9% for IMRT, 99.5% for conformal and 99.5% for conventional technique. PTV-T volume percentage to 66.5 Gy: 98.1% for IMRT, 99.1% for conformal and 99.1% for conventional technique. Coplanar photon beams of 20 MV from a Varian 2300CD were chosen for the treatment simulation in all cases.
Beams arrangement of the three techniques and details of radiotherapy techniques are described in . Conventional technique (a) was planned using 4 beams (2 antero-posterior, postero-anterior and 2 lateral fields) for PTV-N, with a boost of 4 beams (same angles) for PTV-T. Conformal technique (a and 1b) was planned firstly using 4 beams as in the conventional technique for PTV-N, with a boost by 6 beams (including 2 lateral fields and 2 sets of oblique opposed fields, angled at 45° from the lateral fields) for PTV-T. The dose was prescribed at the isocenter and the beam weight planned to ensure the best coverage of CTVs. The dynamic MLC IMRT technique (c) was planned using 5 non-opposed beams with the same isocenter. Fields were selected so that all entrance and exit beams were evenly spaced around the patient.
Figure 1. Beams arrangement of three techniques (conventional 4 + 4 beams, conformal 6 + 4 beams, IMRT 5 beams). All beams were asymmetric with multi-leaf collimator (MLC) and had the same isocenter. For all techniques, PTV-N received a total dose of 46 Gy/23 fractions and PTV-T a boost of 24 Gy/12 fractions. Conventional (a): 4 beams (ANT, POST, LAT270, LAT90) for PTV-N (prostate and seminal vesicles), with a boost of 4 beams (same gantries) for PTV-T (prostate). Conformal (a, b): 4 beams (ANT, POST, LAT270, LAT90) for PTV1, with a boost through 6 beams (LAT270, LAT90, OBL45, OBL135, OBL315, OBL225) for PTV2. IMRT (c): 5 beams (F_0, F_70, F_140, F_220, F_290).
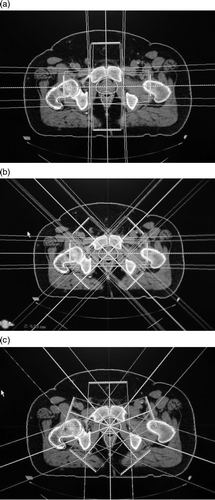
For all techniques, the total dose was 70 Gy, the PTV-N received a total dose of 46 Gy/23 fractions and the PTV-T a boost of 24 Gy/12 fractions with the same angles as for PTV-N, with fractions of 2 Gy given 5 times weekly. All beams were asymmetric through the use of MLC. The same isocenter was used for PTV-N and PTV-T. Since this is a comparative study, the same prescription was applied to all plans that were normalized at the center of PTV-T. For comparison purposes, we used the same dose-volume constraints for all techniques. For the dose optimization of IMRT, constraints to the surrounding critical organs were defined as follows: no more than 25% of the rectum was allowed to receive a dose greater than 62 Gy; no more than 25% of the bladder was allowed to receive a dose greater than 62 Gy; and no more than 5% of both femoral heads were allowed to receive a dose greater than 50 Gy. The dose-volume constraints applied to the OARs are rather “soft” objectives and OARs constraints were given the higher weights than PTV.
The isodose distributions were compared for the three techniques. The DVHs of the three techniques were compared for PTVs, whole pelvis, and OARs: rectum, bladder and femoral heads.
Whole pelvis and low-dose volume
The whole pelvis was defined as the part of the pelvis explored by the CT-scan. The low dose volume was defined as that comprised between the isodoses 5 and 30 Gy.
Whole body radiation dose estimation
Dose estimation to peripheral organs was performed with a software package, Dos_EG, which was developed at the IGR Citation[16], Citation[17]. In the first step of this software, a ‘‘virtual’’ individual phantom is designed for the patient based on the patient's gender, height, anatomical dimensions of the trunk and inclination of the head. The phantom simulates water and lung heterogeneity, but not bony structures.
The second part of the software deals with dose calculation based on entered patient treatment data. The calculation of the absorbed dose is based on an algorithm that includes the primary beam, and takes into account scatter in- and outside of the treatment beams, and of leakage and scattered radiation around the treatment machine derived from direct measurements. Wedges and blocks can be simulated and correction for air cavities of the phantom is made. The absorbed dose was estimated at 188 points in the body (153 points, excepting the pelvis), for each technique of radiation therapy. The absorbed dose received at any site of interest for conventional radiotherapy was compared with the dose at the corresponding site for conformal radiotherapy and IMRT. With the constraints of our software we could not estimate the leakage through the MLC. However, there were many more monitor units (about 30–40%) delivered with the IMRT technique as compared with the 3D-CRT techniques.
Statistics
Friedman test was used to compare DVHs of the three techniques at each dose level; all the tests were calculated in the statistic software SPSS 12.0 for Windows.
Results
Isodose distribution for the low dose volume on the whole pelvis
The DVH analysis (a) for the six patients and according to the radiation technique showed that IMRT significantly increased the pelvic volume covered by various isodose surfaces below 15 Gy as compared with the conformal and conventional techniques (p = 0.007). However, above 15 Gy the order was inverted. For the volume between 5 and 30 Gy, the mean absolute volume increase related to IMRT was 2 900 ml as compared with the conventional radiotherapy. In b, we compared the three techniques while using the irradiated volume by the conformal radiotherapy at each dose level as a reference (100%). The IMRT 5-beam technique significantly increased the pelvic volume covered by various isodose surfaces below 15 Gy as compared with the conformal technique, and the 8-beam conventional technique decreases the pelvic volume as compared with the 10-beam conformal technique at this dose level. For the DVH of the pelvic volume receiving at least 5 Gy, the mean relative volume increase of IMRT, as directly compared with the conventional radiotherapy was about 40%. However, above 15 Gy the order was inverted, especially at the level of 30–35 Gy, being reduced nearly 40% by IMRT, and increased nearly 70% by the conventional compared with the conformal technique. In summary, IMRT significantly reduced the high dose (>30 Gy) irradiation volume in the pelvis.
Figure 2. Pelvic dose volume analysis comparing the three techniques for the six patients. 2a. Pelvic DVH analysis using Friedman test in each dose range with corresponding p-values. 2b. IMRT and conventional techniques compared with the conformal technique used as reference (percentage of pelvic volume irradiated by IMRT versus conformal, and conventional versus conformal at each dose level). Thus, the 100% line represents the conformal technique, and error bars represents the standard deviations for the six patients. IMRT/ Conf% M and Conv/ Conf% M.: mean of percentage of pelvic volume treated by IMRT and conventional technique respectively versus that treated by conformal technique.
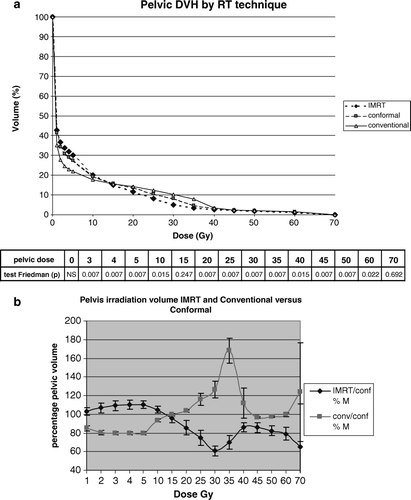
OAR dose-volume
Rectum
The DVH for the rectum of the six patients is shown in . IMRT significantly reduced the volume of irradiated rectum receiving from 5 to 40 Gy as compared with the conformal and conventional techniques. The conformal technique reduced also to some extent the volume of irradiated rectum at the level of 5 to 40 Gy, as compared with the conventional technique. However, the higher dose level (50–70 Gy) volume has not been reduced in the six patients although we found a reduced high dose level volume when it exists a concave shape of the prostate and seminal vesicles surrounding the rectum (data not shown).
Bladder
The DVH for the bladder of the six patients is shown in . IMRT significantly decreased the volume receiving a dose from 5 to 65 Gy compared to the conformal technique and conventional technique.
Femoral head
The DVH for the femurs of the six patients is shown in . IMRT significantly decreased the irradiation volume of femoral heads from the dose of 5 to 35 Gy.
PTV
No significant differences were found in the doses delivered to the 95% of the PTVs volume (D95%). The D95% of PTV-N: 51.2 Gy (SD = 5.7 Gy) for IMRT, 52.3 Gy (SD = 6.3 Gy) for the conformal technique and 51.8 Gy (SD = 6.1 Gy) for the conventional technique. D95% of PTV-T: 67.9 Gy (SD = 0.5 Gy) for IMRT, 68.1 Gy (SD = 0.3 Gy) for the conformal technique and 68.1 Gy (SD = 0.3 Gy) for the conventional technique.
Whole body dose estimation
As shown in , we observed a slight difference in the dose delivered to the 153 dose points in the body outside the irradiation fields, the mean absolute doses received by the conventional, conformal and IMRT techniques were 11.9 cGy, 12.2 cGy and 12.6 cGy, respectively. Comparing with the conventional technique, the mean relative increased dose in the body by the conformal technique and IMRT were 3.2% and 7.1%, respectively. However, our estimation of IMRT did not take into account the leakage irradiation related to the use of MLC.
Table I. Comparison of low radiation doses delivered to the whole body (outside the pelvis) by the three techniques with absolute values (cGy), and increased percentage of dose taking the conventional technique as reference.
Discussion
There are several publications concerning the comparison of dose distribution between IMRT and 3D-conformal radiotherapy for prostate cancer Citation[19–21]. At the Memorial Sloan Kettering Cancer Centre Citation[18], Zelefsky et al. showed that IMRT significantly improved the prescribed dose delivery in the CTV and also reduced the irradiated volumes of rectal and bladder tissues. These data support the notion that the use of IMRT significantly improves the isodose conformation in prostate cancer treatment.
Marchal et al. Citation[19] reported the preliminary results of the French study STIC 2001 aimed to assess the role of IMRT in prostate cancer. They concluded that IMRT may improve the homogeneity of the dose received by the prostate. The dose received by 95% of the PTV-N was 5 Gy higher with IMRT as compared with the conformal technique and the rectal wall received a smaller dose by IMRT. Another French study Citation[20] also showed that the maximal dose to the rectum and the dose received by 25% of the rectal volume were much reduced, especially for concave configurations of the target volume.
In our report, six patients with prostate cancer were studied to compare three irradiation techniques: conventional, conformal and IMRT. The IMRT technique allowed reducing the dose to the organs at risk (), and obtained a similar isodose distribution in the target volume, but leading to an increase of the low-dose pelvic irradiated volume, i.e. between 5 and 30 Gy. We focused the analysis on the low-dose irradiated volume, because of the possibilities of late long-term toxicities, including second malignant neoplasms (SMN). The induction of SMN by radiation exposure is unequivocal since some years after the discovery of x-rays Citation[11], and it has been related to the use of radiotherapy in various tumor sites Citation[12].
Radiotherapy-induced SMN such as lung, breast, bladder and colon cancer etc. have been reported in patients with several types of primary malignancies such as Hodgkin's disease (HD) and testicular cancer for whom the radiation dose is relatively low Citation[23–25]. Regarding SMN after radiotherapy for prostate cancer, Brenner et al. Citation[10] published a study using data from the SEER database. The risk of a second solid tumor of any type was significantly greater after radiotherapy than after surgery, by about 6%. The most dramatic increases were for bladder (77%) and rectum (105%) at 10 years or more after diagnosis. Radiation-induced sarcoma after prostate cancer radiotherapy has occasionally been reported Citation[26].
The exact relationship between SMN and radiation dose has not been fully elucidated Citation[9]. Rubino et al. Citation[27] reported 281 SMN after treatment of breast cancer at the IGR. According to the applied quadratic model, the excess risk of SMN was 0.2% when the involved organ received 1 Gy. The risk of SMN was 6.7-fold higher for doses of ≥25 Gy than in the absence of radiotherapy. This suggests that moderate radiation doses slightly increase the risk of second malignancies. In a cohort of breast-cancer patients Citation[28], the risk of sarcomas was 30.6 higher for doses of more than 44 Gy than for doses of less than 15 Gy. Therefore, we could generally consider that a relatively low dose irradiation (<30 Gy) could induce more second carcinomas mostly appearing 5–10 years after radiotherapy whereas second sarcomas could be induced by a higher irradiation dose.
Our results are consistent with those obtained by Hall et al. Citation[9], who found that the increase of the number of radiation fields produces DVH with a larger volume of normal tissue exposed to lower doses. In addition, the number of monitor units is increased by a factor of 2 to 3, with an increase of the total body exposure, due to leakage radiation. According to Hall et al., IMRT is likely to increase the incidence of SMN as compared with conventional radiotherapy from about 1 to 1.75% for patients surviving 10 years Citation[9]. In our study, we found an increase of 7.1% in the mean dose of different points in the body, outside the pelvis, when we compared IMRT with the conventional technique (). We observed that IMRT increases 30–40% of the MU compared with conventional RT in our study. However, we also observed that IMRT reduced the dose level between 5 and 30 Gy to the rectum and bladder ( and ), and these are the two organs in which most SMN are induced after a pelvic irradiation. Thus, the incidence of these specific SMN would probably not be increased when using IMRT as compared with conformal or conventional techniques. As we showed an increase of the low dose volume irradiation in the pelvis or in the whole body outside the pelvis, we could wonder whether SMN will be increased in the tissues other than rectum and bladder. For this reason, a long-term follow-up will be needed to evaluate this potential iatrogenic effect. However, as in the population of IMRT treated prostate cancer these patients are generally old, the actual increased risk of SMN might be relatively low or undetectable.
Another issue is the neutron contribution to the pelvic and whole body radiation dose. This component is particularly higher with the use of photon energies around 20 MV, as used in the setting of our treatment simulation. The importance of the neutron contribution is also related to the increase of MU related to the use of IMRT. Recently, Kry et al. Citation[21], Citation[22] showed that the risk of secondary malignancy differed substantially between IMRT and conventional radiation therapy for prostate cancer, as well as between different IMRT approaches. They showed that the neutron dose equivalent per MU from beams with energy higher than 15 MV was similar in the conventional and IMRT approaches. Thus, the use of lower photon energies could minimize the out-of-target radiation doses obtained by the IMRT use.
Conclusions
IMRT techniques may give a major advantage in the treatment of prostate cancer by allowing a reduction of the dose to the organs at risk and improving the target volume dose distribution. However, IMRT may also lead to a considerable increase of the low-dose irradiated volume, especially between 5 and 30 Gy in the pelvis, other than rectum and bladder. Certainly a long-term follow-up of IMRT treated prostate cancer patients will be needed for the evaluation of potential late treatment complications, including second malignancies.
Acknowledgements
We thank Anne Beaudré and Charlotte Pichenot for their help in the use of the Treatment planning system; we are grateful to Dr. Carole Rubino for thoughtful help regarding the issue of second malignancies and to Dr. Alberto Bossi for critically reviewing the manuscript.
References
- Mangar SA, Huddart RA, Parker CC, Dearnaley DP, Khoo VS, Horwich A. Technological advances in radiotherapy for the treatment of localised prostate cancer. Eur J Cancer 2005; 41: 908–21
- Pollack A, Zagars GK, Starkschall G, Antolak JA, Lee JJ, Huang E, et al. Prostate cancer radiation dose response: results of the M. D. Anderson phase III randomized trial. Int J Radiat Oncol Biol Phys 2002; 53: 1097–105
- Lebesque JV, van Putten WLJ, Heemsbergen WD. Radiation dose-response in prostate cancer: Results of a multicenter randomized phase III trial comparing 68Gy with 78Gy [Abstract]. Eur J Cancer 2005; Suppl 3: 3 PS3
- Tucker SL, Cheung R, Dong L, Liu HH, Thames HD, Huang EH, et al. Dose-volume response analyses of late rectal bleeding after radiotherapy for prostate cancer. Int J Radiat Oncol Biol Phys 2004; 59: 353–65
- Skwarchuk MW, Jackson A, Zelefsky MJ, Venkatraman ES, Cowen DM, Levegrun S, et al. Late rectal toxicity after conformal radiotherapy of prostate cancer (I): Multivariate analysis and dose-response. Int J Radiat Oncol Biol Phys 2000; 47: 103–13
- Zelefsky MJ, Fuks Z, Hunt M, Yamada Y, Marion C, Ling CC, et al. High-dose intensity modulated radiation therapy for prostate cancer: Early toxicity and biochemical outcome in 772 patients. Int J Radiat Oncol Biol Phys 2002; 53: 1111–6
- Hong TS, Ritter MA, Tome WA, Harari PM. Intensity-modulated radiation therapy: Emerging cancer treatment technology. Br J Cancer 2005; 92: 1819–24
- Bucci MK, Bevan A, Roach M, 3rd. Advances in radiation therapy: Conventional to 3D, to IMRT, to 4D, and beyond. CA cancer J Clin 2005;55:117–34.
- Hall EJ, Wuu CS. Radiation-induced second cancers: The impact of 3D-CRT and IMRT. Int J Radiat Oncol Biol Phys 2003; 56: 83–8
- Brenner DJ, Curtis RE, Hall EJ, Ron E. Second malignancies in prostate carcinoma patients after radiotherapy compared with surgery. Cancer 2000; 88: 398–406
- Sigurdson AJ, Jones IM. Second cancers after radiotherapy: Any evidence for radiation-induced genomic instability?. Oncogene 2003; 22: 7018–27
- Dores GM, Metayer C, Curtis RE, Lynch CF, Clarke EA, Glimelius B, et al. Second malignant neoplasms among long-term survivors of Hodgkin's disease: A population-based evaluation over 25 years. J Clin Oncol 2002; 20: 3484–94
- Travis LB, Andersson M, Gospodarowicz M, van Leeuwen FE, Bergfeldt K, Lynch CF, et al. Treatment-associated leukemia following testicular cancer. J Natl Cancer Inst 2000; 92: 1165–71
- Baxter NN, Tepper JE, Durham SB, Rothenberger DA, Virnig BA. Increased risk of rectal cancer after prostate radiation: A population-based study. Gastroenterology 2005; 128: 819–24
- Moon K, Stukenborg GJ, Keim J, Theodorescu D. Cancer incidence after localized therapy for prostate cancer. Cancer 2006; 107: 991–8
- Diallo I, Lamon A, Shamsaldin A, Grimaud E, de Vathaire F, Chavaudra J. Estimation of the radiation dose delivered to any point outside the target volume per patient treated with external beam radiotherapy. Radiother Oncol 1996; 38: 269–71
- Shamsaldin A, Grimaud E, Hardiman C, Diallo I, de-Vathaire F, Chavaudra J. Dose distribution throughout the body from radiotherapy for Hodgkin's disease in childhood. Radiother Oncol 1998; 49: 85–90
- Zelefsky MJ, Fuks Z, Happersett L, Lee HJ, Ling CC, Burman CM, et al. Clinical experience with intensity modulated radiation therapy (IMRT) in prostate cancer. Radiother Oncol 2000; 55: 241–9
- Marchal C, Lapeyre M, Beckendorf V, Aletti P, Hasle E, Dubois JB, et al. Preliminary results of the assessment of intensity modulated radiotherapy (IMRT) for prostatic and head and neck tumors (STIC 2001). Cancer Radiother 2004; 8(Suppl)(1)S121–7
- Chauvet I, Gaboriaud G, Pontvert D, Zefkili S, Giraud P, Rosenwald JC, et al. Constraints and dosage for prostate cancer patients treated with conformal radiotherapy and intensity modulated radiation therapy. Cancer Radiother 2004; 8: 337–51
- Kry SF, Salehpour M, Followill DS, Stovall M, Kuban DA, White RA, et al. Out-of-field photon and neutron dose equivalents from step-and-shoot intensity-modulated radiation therapy. Int J Radiat Oncol Biol Phys 2005; 62: 1204–16
- Kry SF, Salehpour M, Followill DS, Stovall M, Kuban DA, White RA, et al. The calculated risk of fatal secondary malignancies from intensity-modulated radiation therapy. Int J Radiat Oncol Biol Phys 2005; 62: 1195–203
- Travis LB, Gospodarowicz M, Curtis RE, Clarke EA, Andersson M, Glimelius B, et al. Lung cancer following chemotherapy and radiotherapy for Hodgkin's disease. J Natl Cancer Inst 2002; 94: 182–92
- Travis LB, Hill DA, Dores GM, Gospodarowicz M, van Leeuwen FE, Holowaty E, et al. Breast cancer following radiotherapy and chemotherapy among young women with Hodgkin disease. JAMA 2003; 290: 465–75
- Travis LB, Fossa SD, Schonfeld SJ, McMaster ML, Lynch CF, Storm H, et al. Second cancers among 40,576 testicular cancer patients: Focus on long-term survivors. J Natl Cancer Inst 2005; 97: 1354–65
- Prevost JB, Bossi A, Sciot R, Debiec-Rychter M. Post-irradiation sarcoma after external beam radiation therapy for localized adenocarcinoma of the prostate. Tumori 2004; 90: 618–21
- Rubino C, de Vathaire F, Shamsaldin A, Labbe M, Le MG. Radiation dose, chemotherapy, hormonal treatment and risk of second cancer after breast cancer treatment. Br J Cancer 2003; 89: 840–6
- Rubino C, Shamsaldin A, Le MG, Labbe M, Guinebretiere JM, Chavaudra J, et al. Radiation dose and risk of soft tissue and bone sarcoma after breast cancer treatment. Breast Cancer Res Treat 2005; 89: 277–88