Abstract
Respiratory gating for radiotherapy beam delivery is a widely available technique, manufactured and sold by most of the major radiotherapy machine vendors. Respiratory gated beam delivery is intended to limit the irradiation of tumours moving with respiration to selected parts of the respiratory cycle, and thereby enable dose escalation and/or reduction of dose to organs at risk.
Without adequate use of respiratory correlated image guidance on a regular basis, respiratory beam gating may however have a detrimental effect on target coverage. Image guidance of tumour respiratory motion is therefore of utmost importance for the safe introduction of respiratory gating.
In this short overview, suitable image guidance strategies for respiratory gated radiotherapy are reviewed for two cancer sites; breast cancer and lung tumours.
The ultimate aim of successful radiotherapy is to obliterate the target in question while leaving healthy surrounding tissues undamaged. Because radiotherapy is a non-specific treatment unavoidably leading to irradiation of surrounding healthy tissues, great emphasis is continuously put onto the development of methods to shape a three-dimensionally tight dose distribution around the target.
An important limiting factor to the success of tightly conforming dose distributions is the ability to aim the radiation beam precisely at the target in question with minimal positional error. Positional uncertainty is inherent in radiotherapy, and the prevalent method to account for this is to irradiate a volume larger than the target, an approach standardized in ICRU reports 50 and 62 Citation[1]. Intra-fraction organ motion in the thorax or abdomen can be of a large magnitude due to breathing, and was until recently an unresolved problem of positional error. All organs in the thorax and abdominal regions are affected by breathing, although in different ways and by various degrees. Primarily the lung itself, the heart, liver, chest wall, breast and the diaphragm move with respiration, but also structures such as the kidneys and the prostate can be significantly affected by respiration. A tumour will move along with the organs depending on the tumour location and its fixation to surrounding structures. For most organs there will be motion in all three spatial directions, and for some the inspiration and expiration motion patterns differ from each other, a phenomenon termed hysteresis. The magnitude of the motion is highly dependent on location and is also highly patient individual. Lung tumours have been reported to exhibit motion up to >3 cm in the cranio-caudal direction during normal respiration, while others move only a few millimetres or not at all Citation[32], Citation[5], Citation[13]. In several studies, the median lung tumour motion extent over the population of patients appear to be around 5 − 10 mm in the cranio-caudal direction Citation[13]. The breast only moves around 2–4 mm in the antero-posterior direction during normal respiration, while the heart moves up to several centimetres in the cranio-caudal direction (with the diaphragm) Citation[20], Citation[21], Citation[23], Citation[39].
Breathing motion contributes not only to the positional error during treatment delivery, but also to positional errors in treatment planning, which are of a systematic nature. For free breathing imaging, the breathing motion contributes to the systematic error, because the target may be off-set from its mean position at the time of imaging.
During the 1990s, techniques of respiratory beam gating were proposed to solve this problem Citation[25], Citation[21], Citation[35], Citation[22]. With these techniques, the radiation beam is only turned on during a pre-specified period of the respiration. The organ motion within the radiation field is thus limited to the motion taking place during the beam-on time specified by the beam-on window. Respiratory gated radiotherapy generally requires a motion sensor, a method of determining a suitable beam-on period, a controller to activate/deactivate the beam, acceptable beam characteristics during beam-on time, and a means of verification.
Respiratory gating techniques can and should be used consistently in connection with simulation, CT scanning, planning and treatment in order to obtain consistency Citation[13]. Treatment planning of gated treatment requires knowledge of the patient anatomy and dynamics within the window chosen for beam-on. Some of this knowledge can be obtained with fluoroscopy, but for computerized treatment planning, a CT-scan reflecting the relevant patient anatomy is required. Such a CT-scan may be obtained by running the CT-scanner with the same “beam-on” window as will be used during treatment, also referred to as prospective gated CT-scanning. With this technique, the gating system sends trigger signals to the scanner to acquire each CT-slice in synchronisation with the respiratory signal. Even better in many cases, a 4DCT scan may be acquired for which the complete scan will encompass several sets of slices, each set corresponding to different phases of the breathing cycle, and each set containing an adequate number of slices for target imaging and dose planning Citation[38], Citation[14].
Beam gating is offered by several manufacturers of treatment machines, and the systems offered commercially for respiratory monitoring and beam gating all make use of a surrogate for the respiratory tumour motion, such as external optical markers, pressure belts or spirometers. A gating window can be defined by placing thresholds on the respiratory signal from the surrogate monitoring system, which can be used to automatically (or manually) trigger the treatment beam to be turned on and off (see ). Respiratory correlated image guidance is of utmost importance in the safe implementation of respiratory beam gating due to the large intra- and inter-fraction variations in respiratory pattern affecting tumour motion, as has been shown previously Citation[11], Citation[16], Citation[34]. In Citation[34], the standard deviation of systematic variations in mean tumour position (motion baseline) was found to be 3.9 mm, and in Citation[16] large variations in motion patterns were found intra-fractionally including variations in both motion extent and trajectory pattern.
Figure 1. A respiratory signal obtained from the RPM™ system (Varian Medical Systems, Inc) showing the vertical motion of an optical marker placed on a patient's chest wall versus time. Two horizontal lines overlayed on the breathing curve indicate a chosen gating window in the expiration phase of breathing, and below a step curve indicates beam on and off times.
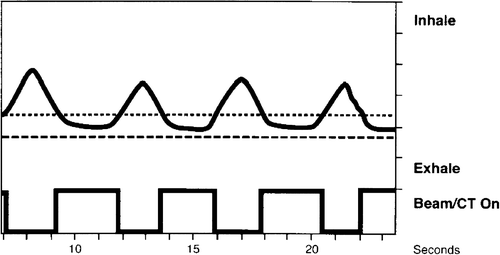
Basically, the image guidance is needed to ensure that the treatment scenario as closely as possible mimics the scenario seen in the image for treatment planning, in the case of respiratory gating the phase-specific CT-image (gated or picked from a 4DCT scan).
A number of the commercially available systems on the market for respiration monitoring and/or beam gating (and tumour tracking) offer an image guidance solution as a complement to the surrogate respiration monitoring Citation[9], Citation[31], Citation[27]. However, these commercially available gating systems also allow use of the gated beam delivery without the necessary amount of image guidance to ensure that this can be done safely. Furthermore, for several of the available systems, the image guidance (hardware and software) offered is inadequate although it provides a means of performing imaging in synchronization with the respiration.
In the following, we will go through the need for image guidance for the safe implementation of respiratory beam gating in two different cancer sites; breast cancer, and lung cancer.
Respiratory gating for breast cancer radiotherapy
Tangential field breast cancer radiotherapy is mainly employed adjuvantly to breast-conserving surgery in otherwise healthy women to improve local control and survival. In these cases, a primary concern is unwanted pulmonary and cardiac irradiation, which implies a risk of late toxicity. Cardiac mortality is reported to be higher in patients receiving radiotherapy for left-sided breast cancer than in patients receiving radiotherapy for right-sided breast cancer by ∼1% Citation[26]. In Citation[30], the relative risk of heart disease is reported up to above 2% with effective heart radiation doses of 2–4 Gy, and increased cardiac morbidity as well as mortality has been reported in Citation[8], especially in combination with smoking.
During inspiratory chest wall expansion, the heart is pulled inferiorly, increasing the distance to the breast and lymph nodes, and for this reason, breathing adapted radiotherapy has been investigated for breast cancer. It has been shown that by treating only in the inspiration phase of free breathing or breathing with enhanced inspiration, the doses to both heart and lungs can be reduced significantly Citation[20], Citation[18], Citation[28]. Administering radiation only during deep inspiration has shown reductions of heart doses up to 80–90% (of volume receiving more than 50% of prescription dose), corresponding to a decrease in cardiac mortality risk down to 1‰ (calculated by a normal tissue complication probability model Citation[19]).
Because breast movement with respiration is small (2–4 mm, Citation[20]), the question of respiratory target immobilization is of less importance for this site. Since the breast is furthermore an external structure, an external optical marker positioned near the breast and used for respiration monitoring will mimic the motion of the breast closely, and thus the correlation of respiration phase and target position is simple.
The need for image guidance is in this case rather uncomplicated. Standard image guided setup of the patient using kV or MV images will ensure the correct positioning of the bony anatomy, which is not affected by respiration. Additionally, images should be acquired to ensure the correct positioning of the breast within the gating thresholds. This can be done using MV portal images in the beam's eye view acquired within the gating window. When this verification is performed immediately before treatment, the correct positioning of the breast throughout the beam-on time can be inferred from the monitoring of the external marker position as a surrogate for the breast position. Some portal imaging devices are capable of performing imaging in cine-mode during beam on, as illustrated in . Such a facility can be used to verify the actual breast position during beam-on in an off-line review setting, as an additional safety measure. In our clinic, we use kV-kV orthogonal imaging for bony anatomy setup of the breast cancer gating patients in a no-action-level protocol on the first three days of treatment. Additionally, we perform MV imaging in the gating window on the first treatment day, and cine portal imaging on a weekly basis as a verification tool. Over 43 patients, we have measured average residual motion of 1.5mm left-right, 2.3mm anterior-posterior and 2.3mm superior-inferior (reported in Citation[4]), which is within expected magnitudes for an enhanced inspiration breathing protocol Citation[19].
Figure 2. Illustration of the acquisition of portal MV images in the beam's eye view for one of the tangential fields throughout a gated breast cancer treatment. At least one image is acquired in each gating window.
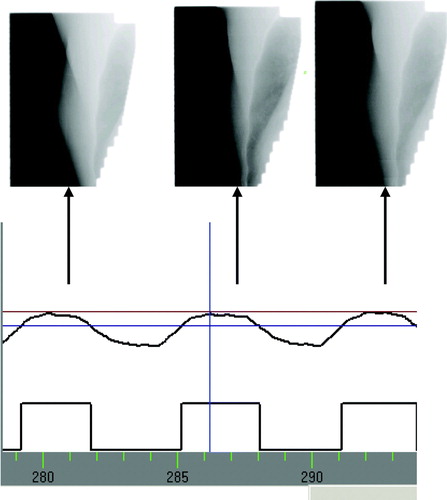
The use of kV image guidance is less suited for breast cancer gating verification, as the breast is not easily recognized in kV images. However, on-board kV may be used to image the position of the diaphragm rather than the breast, in radiographic images or fluoroscopic images before or during treatment (for gantry mounted systems however in angles different than the treatment beam angle), or in 4D CBCT images before treatment. The heart moves with the diaphragm, and imaging of the diaphragm position can therefore be used as a good surrogate for the heart position. The heart is the most important organ at risk in breast irradiation, and it may therefore be of some relevance to verify the heart position in addition to the breast position for the breast cancer gating treatment.
Respiratory gating for lung tumour radiotherapy
Radiotherapy is widely used in the treatment of locally advanced lung cancer. Curative radiotherapy requires high radiation doses, but the amount of normal lung in the high-dose region is generally the dose-limiting factor. For NSCLC stage I and II, radiotherapy can provide local disease control with a control rate of 80–90% and a 3-year survival of around 50% Citation[2], Citation[29]. For locally advanced NSCLC (stage III), the 2-year survival is 10–30%, and the toxicity is the dose-limiting factor Citation[3]. A general agreement does not exist in the literature as to the cut-off magnitude for radiation dose to healthy lung, but our local guideline states that no more than 30% of the volume of the ipsilateral lung should receive more than 20 Gy, and that the contralateral lung is completely spared.
Lung tumours have been shown to move substantially – up to more than 3 cm – even during shallow breathing. Thus, reduction of tumour motion, and thereby internal treatment margins, is of importance to maximize the dose. Respiratory gated treatment provides a possibility of motion (and thereby margin) reduction. Furthermore, the lung volume (and hence the lung tissue density) varies during respiration, and following anatomical changes and/or electron transport changes may favour certain breathing phases.
In the case of lung cancer, the use of external surrogates for respiration monitoring, such as those used in the commercially available gating systems, does not give a direct monitoring of the actual tumour position and motion. With a (good) choice of surrogate, there will be a correlation between the surrogate and the actual tumour motion, however the correlation will not be ideal, and it will not be stable over time Citation[16], Citation[7]. The use of beam gating assumes knowledge of the tumour position, although the systems act on surrogates, and it is therefore mandatory to employ image guidance to establish the correlation between the surrogate and the tumour motions. The variations in the correlation between surrogate and tumour can be divided into four different types; mean position shifts (translation), amplitude changes (scaling), phase shift changes (latency), and changes in correspondence (breakdown of correlation model, including breathing frequency changes). All of these variations can take place both on intra- and inter-fractional basis, and can be of a substantial magnitude. Interfraction baseline variations have been shown to have a standard deviation of ∼4 mm Citation[34], and amplitude variations have been shown to be slightly smaller Citation[11] although still of a substantial magnitude. Intrafractional baseline and amplitude variations are also reported Citation[11], Citation[16], and phase shift variations can be as large as several tenths of seconds intra- and inter-fractionally Citation[16], Citation[7]. Some examples of synchronous external surrogate and tumour motion measurements are shown in (from Citation[17]).
Figure 3. Three examples of synchronous recordings of external optical marker (blue) and tumour respiratory motion (magenta). The optical marker (RPM™) is positioned on the chest wall of a patient, and the vertical motion is recorded. The tumour has implanted gold seeds, which have been automatically tracked in fluoroscopic movies. For two of the cases, the cranio-caudal tumour motion is shown and for one case the medio-lateral motion. (From Citation[17]).
![Figure 3. Three examples of synchronous recordings of external optical marker (blue) and tumour respiratory motion (magenta). The optical marker (RPM™) is positioned on the chest wall of a patient, and the vertical motion is recorded. The tumour has implanted gold seeds, which have been automatically tracked in fluoroscopic movies. For two of the cases, the cranio-caudal tumour motion is shown and for one case the medio-lateral motion. (From Citation[17]).](/cms/asset/7b7f137c-47dc-4af9-9f4d-9200c0e1ab5c/ionc_a_328445_f0003_b.jpg)
Accounting for these variations inter-fractionally requires image guidance immediately before beam on in each treatment fraction. Accounting for the variations intra-fractionally requires imaging during beam-on either continuously (fluoroscopic) or occasionally (single radiographic images).
In order to account for baseline (mean position) shifts, a technique is needed in which the respiratory motion of the tumour can be imaged and quantified to the extent that a baseline can be established. This requires imaging over at least one entire breathing cycle, and corresponding quantification of tumour positions within the timeframe of the images. It is imaginable that 4D cone-beam CT, respiratory correlated fluoroscopy or repeated radiographs could potentially accomplish this, given that suitable software is provided to establish a quantification of the tumour position in the images, and find a corresponding baseline to which setup can be performed.
Imaging of amplitude changes likewise requires a technique in which the respiratory motion of the tumour can be imaged and quantified, and a setup to the correct target position in the chosen gating phase of respiration can be performed.
In order to account for phase shifts, a technique is needed which allows the synchronous recording of the respiratory surrogate and the tumour motion over several breathing cycles. In our estimation, imaging over 5–10 breathing cycles will be necessary in order to establish the internal/external correlation to an extent that a possible phase shift can be quantified. Furthermore, a method and a software tool for quantifying the phase shifts is necessary. It is imaginable that respiratory fluoroscopy or repeated radiographs can accomplish this. Once a phase shift has been identified, an action has to be taken to shift the beam gating window to accommodate the phase shift with minimal tumour motion in the desired phase of respiration. The system should allow for such action to be taken and for subsequent imaging for verification.
Breakdown of the correlation between external surrogate marker and tumour motion is the most difficult type of variations to detect and account for. In order to establish a correlation model, synchronous recording of the respiratory surrogate and the tumour motion over several breathing cycles (5–10) is necessary, and a method for performing the modelling must also be available. Model breakdowns can be detected by repeated imaging of this type, combined with software for detection of deviations from expected conditions. In order to account for a model breakdown, a new correlation should be established based on synchronous recording of the respiratory surrogate and the tumour motion. The correct gating window should then be re-identified on the surrogate respiratory signal based on this new model.
Model breakdowns and amplitude variations may be counter-acted by use of coaching of patients to regular breathing patterns. Audio and visual coaching may enhance regularity of breathing, by stabilizing amplitude and frequency magnitudes Citation[15], Citation[6]. The success of coaching strategies depends to a large degree of patient compliance, and lung cancer patients may have some difficulties following instructions due to their poor lung function.
A solution covering all of these issues directly would be gating directly based on imaging the position of internal markers as described in Citation[33]. However, this solution is not an off-the-shelf commercially available system (although it was manufactured by Mitsubishi Electronics Co, Ltd), and it requires continuous imaging during treatment which may add a significant amount of dose (especially skin dose) to the patient (in our own measurements on a simulator – Acuity, Varian Medical Systems – one minute of fluoroscopic imaging gave approximately 70 mGy skin dose).
Discussion
Breathing adaptation is a relatively new addition to external radiotherapy for tumour sites affected by the movements related to breathing. It will potentially improve therapy outcome by allowing reduced treatment margins and/or increased anatomical separation leading to enhanced tumour control and lower complication rates Citation[13]. Respiratory beam gating is the most commonly used breathing adaptation technique, and is offered in several commercially available systems. Other breathing adaptation techniques include breath-hold techniques (voluntary and controlled) Citation[41] and dynamic motion tracking in which the beam “chases” the target during its respiratory motion Citation[12], Citation[24]. In the simpler end, motion inclusion techniques can be based on 4DCT scans to cover the target respiratory motion with treatment field margins on a patient individual basis Citation[40].
The systems most frequently investigated for respiratory gating are those employing spirometry or external marker monitoring to represent the position of the internal anatomy. Each system has specific advantages that appear to be applicable to specific cancer types, e.g. free breathing appears to be more appealing to lung cancer patients than forced breath-holding.
Treating patients using respiratory gating implies subtle clinical decisions, such as whether to treat during expiration or inspiration and how much target motion can be reduced at the expense of increased treatment time. The optimal parameters for use of the techniques in each cancer group are still in the process of being established Citation[10].
The benefits of respiratory gating are best documented for the case of breast cancer. Significant reductions in cardiac and pulmonary doses can be obtained, not so much due to target immobilization, but owing to organ separation caused by lung inflation in the inspiration phase of breathing Citation[20]. The clinical feasibility of the technique (both breath-hold and free breathing) has been shown in several studies Citation[19], Citation[27].
Lung cancer is the site most frequently studied for respiratory gating. The potential benefits are clear, but challenges are many and some still unsolved. Respiratory gating can provide target immobilization and thereby in principle reduction of doses to organs at risk and/or tumour dose escalation Citation[36]. However, owing to the complex mobility of lung tumours with respiration, the accurate tumour position during respiratory gating is presently only well-known if internal markers for real-time tracking are used Citation[10]. Methods to correlate internal and external breathing motion and coaching for achieving regular breathing patterns are presently being explored. Furthermore, it is still not well-established whether inspiration or expiration is the most favourable breathing phase for respiratory gated lung radiotherapy. Despite all these complications, respiratory gating is used clinically for lung cancer at several centers in conjunction with either direct tumour tracking or extensive positional verification (such as frequent portal imaging) Citation[37].
For all cancer sites, there are only few studies reporting clinical outcome, owing primarily to the small number of patients yet treated with the techniques, and the limited time span yet available for outcome evaluation.
Declaration of interest: This study has beeb partly funded by a research grant from Varian Medical Systems, Inc.
References
- ICRU Report 62, Prescribing, recording and reporting photon beam therapy (Supplement to ICRU Report 50). 1999;62.
- Baumann P, Nyman J, Lax I, et al. Factors important for efficacy of stereotactic body radiotherapy of medically inoperable stage I lung cancer. A retrospective analysis of patients treated in the Nordic countries. Acta Oncol 2006; 45: 787–95
- Byhardt RW, Scott C, Sause WT, et al. Response, toxicity, failure patterns, and survival in five Radiation Therapy Oncology Group (RTOG) trials of sequential and/or concurrent chemotherapy and radiotherapy for locally advanced non-small-cell carcinoma of the lung. Int J Radiat Oncol Biol Phys 1998; 42: 469–78
- Enmark M, Korreman S, Nottrup TJ, et al 3 years clinical routine breathing adapted radiotherapy for breast cancer: Was it clinically feasible? Proceedings of the 11th Varian European Users Meeting 2007.
- Erridge SC, Seppenwoolde Y, Muller SH, et al. Portal imaging to assess set-up errors, tumor motion and tumor shrinkage during conformal radiotherapy of non-small cell lung cancer. Radiother Oncol 2003; 66: 75–85
- George R, Chung TD, Vedam SS, et al. Audio-visual biofeedback for respiratory-gated radiotherapy: Impact of audio instruction and audio-visual biofeedback on respiratory-gated radiotherapy. Int J Radiat Oncol Biol Phys 2006; 65: 924–33
- Hoisak JD, Sixel KE, Tirona R, Cheung PC, Pignol JP. Correlation of lung tumor motion with external surrogate indicators of respiration. Int J Radiat Oncol Biol Phys 2004; 60: 1298–306
- Hooning MJ, Botma A, Aleman BM, et al. Long-term risk of cardiovascular disease in 10-year survivors of breast cancer. J Natl Cancer Inst 2007; 99: 365–75
- Huntzinger C, Munro P, Johnson S, et al. Dynamic targeting image-guided radiotherapy. Med Dosim 2006; 31: 113–25
- Jiang SB. Technical aspects of image-guided respiration-gated radiation therapy. Med Dosim 2006; 31: 141–51
- Juhler NT, Korreman SS, Pedersen AN, et al. Intra- and interfraction breathing variations during curative radiotherapy for lung cancer. Radiother Oncol 2007; 84: 40–8
- Keall PJ, Joshi S, Vedam SS, Siebers JV, Kini VR, Mohan R. Four-dimensional radiotherapy planning for DMLC-based respiratory motion tracking. Med Phys 2005; 32: 942–51
- Keall PJ, Mageras GS, Balter JM, et al. The management of respiratory motion in radiation oncology report of AAPM Task Group 76. Med Phys 2006; 33: 3874–900
- Keall PJ, Starkschall G, Shukla H, et al. Acquiring 4D thoracic CT scans using a multislice helical method. Phys Med Biol 2004; 49: 2053–67
- Kini VR, Vedam SS, Keall PJ, Patil S, Chen C, Mohan R. Patient training in respiratory-gated radiotherapy. Med Dosim 2003; 28: 7–11
- Korreman S, Mostafavi H, Le QT, Boyer A. Comparison of respiratory surrogates for gated lung radiotherapy without internal fiducials. Acta Oncol 2006; 45: 935–42
- Korreman SS, Juhler-Nottrup T, Boyer AL. Respiratory gated beam delivery cannot facilitate margin reduction, unless combined with respiratory correlated image guidance. Radiother Oncol 2008; 86: 61–8
- Korreman SS, Pedersen AN, Aarup LR, Nottrup TJ, Specht L, Nystrom H. Reduction of cardiac and pulmonary complication probabilities after breathing adapted radiotherapy for breast cancer. Int J Radiat Oncol Biol Phys 2006; 65: 1375–80
- Korreman SS, Pedersen AN, Josipovic M, et al. Cardiac and pulmonary complication probabilities for breast cancer patients after routine end-inspiration gated radiotherapy. Radiother Oncol 2006; 80: 257–62
- Korreman SS, Pedersen AN, Nottrup TJ, Specht L, Nystrom H. Breathing adapted radiotherapy for breast cancer: Comparison of free breathing gating with the breath-hold technique. Radiother Oncol 2005; 76: 311–8
- Kubo HD, Hill BC. Respiration gated radiotherapy treatment: A technical study. Phys Med Biol 1996; 41: 83–91
- Minohara S, Kanai T, Endo M, Noda K, Kanazawa M. Respiratory gated irradiation system for heavy-ion radiotherapy. Int J Radiat Oncol Biol Phys 2000; 47: 1097–103
- Nehrke K, Bornert P, Manke D, Bock JC. Free-breathing cardiac MR imaging: Study of implications of respiratory motion--initial results. Radiology 2001; 220: 810–5
- Neicu T, Berbeco R, Wolfgang J, Jiang SB. Synchronized moving aperture radiation therapy (SMART): Improvement of breathing pattern reproducibility using respiratory coaching. Phys Med Biol 2006; 51: 617–36
- Ohara K, Okumura T, Akisada M, et al. Irradiation synchronized with respiration gate. Int J Radiat Oncol Biol Phys 1989; 17: 853–7
- Paszat LF, Mackillop WJ, Groome PA, Schulze K, Holowaty E. 2 Mortality from myocardial infarction following postlumpectomy radiotherapy for breast cancer: A population-based study in Ontario, Canada. Int J Radiat Oncol Biol Phys 1999; 43: 755–62
- Remouchamps VM, Letts N, Vicini FA, et al. Initial clinical experience with moderate deep-inspiration breath hold using an active breathing control device in the treatment of patients with left-sided breast cancer using external beam radiation therapy. Int J Radiat Oncol Biol Phys 2003; 56: 704–15
- Remouchamps VM, Vicini FA, Sharpe MB, Kestin LL, Martinez AA, Wong JW. Significant reductions in heart and lung doses using deep inspiration breath hold with active breathing control and intensity-modulated radiation therapy for patients treated with locoregional breast irradiation. Int J Radiat Oncol Biol Phys 2003; 55: 392–406
- Rowell NP, Williams CJ. Radical radiotherapy for stage I/II non-small cell lung cancer in patients not sufficiently fit for or declining surgery (medically inoperable): A systematic review. Thorax 2001; 56: 628–38
- Schultz-Hector S, Trott KR. Radiation-induced cardiovascular diseases: Is the epidemiologic evidence compatible with the radiobiologic data?. Int J Radiat Oncol Biol Phys 2007; 67: 10–8
- Seppenwoolde Y, Berbeco RI, Nishioka S, Shirato H, Heijmen B. Accuracy of tumor motion compensation algorithm from a robotic respiratory tracking system: A simulation study. Med Phys 2007; 34: 2774–84
- Seppenwoolde Y, Shirato H, Kitamura K, et al. Precise and real-time measurement of 3D tumor motion in lung due to breathing and heartbeat, measured during radiotherapy. Int J Radiat Oncol Biol Phys 2002; 53: 822–34
- Shirato H, Shimizu S, Kunieda T, et al. Physical aspects of a real-time tumor-tracking system for gated radiotherapy. Int J Radiat Oncol Biol Phys 2000; 48: 1187–95
- Sonke JJ, Lebesque J, van HM. Variability of four-dimensional computed tomography patient models. Int J Radiat Oncol Biol Phys 2008; 70: 590–8
- Tada T, Minakuchi K, Fujioka T, et al. Lung cancer: Intermittent irradiation synchronized with respiratory motion--results of a pilot study. Radiology 1998; 207: 779–83
- Underberg RW, Van Sornsen de Koste JR, Lagerwaard FJ, Vincent A, Slotman BJ, et al. A dosimetric analysis of respiration-gated radiotherapy in patients with stage III lung cancer. Radiat Oncol 2006;18, –
- Van Sornsen de Koste JR, Cuijpers JP, de Geest FG, Lagerwaard FJ, Slotman BJ, Senan S. Verifying 4D gated radiotherapy using time-integrated electronic portal imaging: A phantom and clinical study Radiat Oncol 2007;232–
- Vedam SS, Keall PJ, Kini VR, Mostafavi H, Shukla HP, Mohan R. Acquiring a four-dimensional computed tomography dataset using an external respiratory signal. Phys Med Biol 2003; 48: 45–62
- Wang Y, Riederer SJ, Ehman RL. Respiratory motion of the heart: Kinematics and the implications for the spatial resolution in coronary imaging. Magn Reson Med 1995; 33: 713–9
- Wolthaus JW, Schneider C, Sonke JJ, et al. Mid-ventilation CT scan construction from four-dimensional respiration-correlated CT scans for radiotherapy planning of lung cancer patients. Int J Radiat Oncol Biol Phys 2006; 65: 1560–71
- Wong JW, Sharpe MB, Jaffray DA, et al. The use of active breathing control (ABC) to reduce margin for breathing motion. Int J Radiat Oncol Biol Phys 1999; 44: 911–9