Abstract
Purpose. To investigate the impact of prone versus supine patient set-up and use of various image-guidance protocols on residual set-up error for radiation therapy of pelvic malignancies. We aim to identify an optimal frequency and protocol for image-guidance. Materials. Using daily online image-guidance mega-voltage CT data from 30 patients (829 MVCT; 299 prone set-up on belly board, 530 supine set-up), we retrospectively assessed the impact of various image-guidance protocols on residual set-up error. We compared daily image-guidance with three different No Action Level protocols (NAL), alternate day image-guidance with running mean and weekly image-guidance. Results. Of 5 IGRT protocols analyzed, the protocol with the highest imaging frequency, alternate day imaging with a running mean (50% imaging frequency), provided the best set-up error reduction. This protocol would have reduced the average length of 3D corrective vector shifts derived from daily image-guidance from 15.2 and 13.5 mm for prone and supine set-up, to 5 and 5.4 mm, respectively. A NAL protocol, averaging shifts of the first 3 fractions (NAL3), would have reduced the respective set-up variability to 6.3 (prone), and 7.5 mm (supine). An extended NAL (eNAL) protocol, averaging shifts of the first 3 fractions plus weekly imaging, would have reduced the daily positioning variability to 6 mm for both prone and supine set-ups. Daily image-guidance yielded set-up corrections >10 mm in 64.3% for prone and 70.3% for supine position. Use of the NAL3 protocol would have reduced the respective frequency to 14.4%, and 21.2% for prone, and supine positioning. In comparison, the alternate day running mean protocol would have reduced the frequency of shifts >10 mm to 5.5% (prone), and 8.3% (supine), respectively. Discussion. In this comparison, high frequency image-guidance provided the highest benefit with respect to residual set-up errors. However, both NAL and eNAL protocols provided significant set-up error reduction with lowered imaging frequency. While the mean 3D vector of corrective shifts was longer for prone set-up compared to the supine set-up, using any image-guidance protocol would have reduced shifts for prone set-up to a greater extent than for the supine set-up. This indicates a greater risk for systematic set-up errors in prone set-up, and larger random errors using a supine patient set-up.
The advantages of prone versus supine set-up for pelvic radiation therapy have been extensively studied with respect to normal tissue radiation exposure reduction, namely the reduction of the small bowel dose in favor of prone patient set-up on a Belly Board Citation[1–6]. Early reports found prone set-up to be comfortable, and highly reproducible Citation[3]. Despite the long-term clinical experience in the use of prone set-up with “false” table tops, this set-up strategy is not globally accepted. Concerns regarding patient acceptance and intra-fraction target motion affecting the accuracy of dose delivery in a prone set-up have been supported by peer-reviewed reports Citation[7–9]. Prone patient positioning has been documented as a more difficult set-up for the radiation technologists, and has been perceived as being less reproducible, potentially yielding the risk for compromised local tumor control Citation[5], Citation[7], Citation[10]. Also, the advent of modern radiation planning and delivery techniques, namely the clinical implementation of intensity-modulated radiation therapy (IMRT) is considered by parts of the clinical community to sufficiently compensate for positional geometric advantages provided by prone set-up.
Regardless of patient set-up techniques, the increasing use of target conformal radiation techniques for treatment of pelvic tumors with steep dose gradients between the tumor and surrounding tissues, and/or the use of reduced safety margins for planning target volume (PTV) creation, yield the risk of missing a target on any given day of a radiation treatment course, unless means of assessing optimal target set-up are employed. Image-guided radiation therapy (IGRT) affords assessment of target positioning with adjustments to positional set-up errors Citation[11–14]. However, image-guidance adds to the overall treatment time and – when using x-ray based techniques – exposes the patient to an additional, albeit small dose of radiation Citation[15]. Given these considerations, and an often limited availability of radiation delivery units with volumetric x-ray based image-guidance capabilities, it appears necessary to compare the advantages of daily image-guidance to protocols with lower imaging frequency Citation[16], Citation[17].
In the treatment of pelvic radiation target volumes, image-guidance allows to re-visit the question of benefits provided by prone compared to supine patient set-up. Volumetric, three-dimensional image data sets afford assessment of translational set-up optimization along the principal room axes. In this study, daily mega-voltage CT (MVCT) imaging with a helical tomotherapy unit (HiArt, TomoTherapy Inc., Madison, WI, USA) was used to collect patient position data for pelvic neoplasms in either supine or prone set-ups. Besides comparing the set-up accuracy of the respective patient position, we studied the impact of different imaging frequencies on residual set-up errors. The aim of this study was to assess benefits and tradeoff of supine versus prone patient position with respect to daily target set-up accuracy, and to identify an optimal image-guidance protocol, with specific emphasis on the possibility to reduce imaging frequency.
Materials & methods
Using volumetric mega-voltage CT (MVCT) imaging, acquired on a helical tomotherapy unit (TomoTherapy HiArt), image-guidance data was collected for 30 patients treated by pelvic IMRT. Patient set-up for simulation and treatment was prone in 11 and supine in 19 patients. Prone set-up always involved a belly board. A total of 829 MVCT image-guidance studies (299 prone, 530 supine) were acquired. Target volumes studied here had a close relationship with the patient's bony pelvic anatomy. Thus, the bony skeletal patient set-up was representative of target set-up (). Typical radiation target volumes with close relationship to the pelvic bony anatomy include nodal treatments for prostate cancer, and GYN tumors, as well as rectal and anal cancers in the pelvic region. All patients with rectal or anal cancers (n = 11) were treated in the prone position. All other cancers were treated in the supine position. Prostate cancer was treated in 13 patients, large pelvic sarcoma in 3 patients and one patient each for uterine, pelvic and bladder cancers.
Figure 1. Typical MVCT image-guidance for deep seated rectal cancer. Upper figure depicts the simulation CT with target and structure outlines (CTV in white, PTV in red, femoral heads in green, anterior dose tuning structure in yellow); the relationship between target volume and bony patient anatomy is apparent. The lower figure represents a corresponding image-guidance MVCT following set-up correction. The simulation CT derived structure outlines are superimposed onto the MVCT.
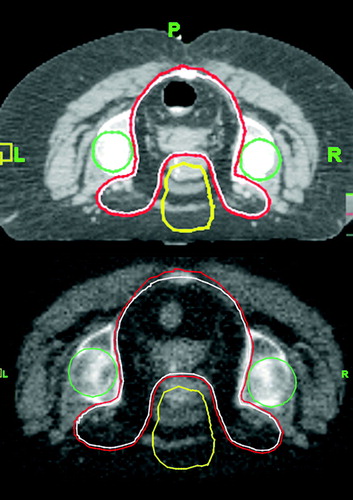
Average target set-up optimization shifts compared daily image-guidance MVCTs with the simulation CT study. Shifts along the three principal directions (x, y & z) were calculated over the entire number of fractions. Shifts were calculated for relative data (positive and negative around a common origin) and also as absolute data. The average shift for each patient was then averaged over the entire patient set. The 3D vector of target set-up optimization shift was calculated using the equation below:
While all clinical cases were treated according to a daily online image-guided protocol with patient set-up to skin marks defined at the time of simulation (Protocol A), we retrospectively studied the potential impact of other image-guidance strategies on residual set-up error.
Protocol A represents the raw data on which other protocols B – F were applied for comparison (see for imaging frequencies of the respective protocols). The data reported are the corrective shifts executed on the respective treatment days, using online MVCT image-guidance. For the purpose of this analysis, it is assumed that these shifts represent the true set-up error with no residual set-up errors.
Table I. Different protocols used for retrospective analysis.
Protocol B represents a No Action Level protocol (NAL) Citation[18]. For this NAL protocol, imaging is acquired for the first three consecutive days of a radiation treatment course (NAL3). The targets location and set-up optimization shifts are averaged over these 3 days, and all subsequent set-ups are adjusted for those shifts. No additional image-guidance studies are obtained. Since imaging is done on the first three treatment days, the imaging frequency for an assumed course of 30 fractions is 10%. No positional correction is applied for the first three fractions when imaging data is being collected.
Protocol C is similar to the NAL3 protocol except the first five fractions are imaged instead of the first three (NAL5). For this protocol imaging for five out of 30 fractions yields an imaging frequency of 17%. Similar to Protocol B no positional correction is applied for the first 5 fractions.
Protocol D corresponds to once a week imaging (every 5th fraction). Shifts derived from each imaging instance are applied to the subsequent 4 fractions. Weekly set-ups are typically only corrected for subsequent fractions when a defined threshold of set-up uncertainty is exceeded, for the purpose of this study; this threshold was set to 5 mm along any room axis. This reflects typical clinical practice in which imaging is acquired on the first day of treatment, and then once weekly. Again assuming a 30 fraction treatment course, the imaging frequency is 17%.
Protocol E is a combination of the NAL3 protocol and Protocol D and is referred to as the extended No Action Level (eNAL) protocol Citation[18], Citation[19]. With this protocol, imaging is done for the first three days, followed by weekly imaging. A threshold of 5 mm for weekly imaging was defined. If the patient set-up during weekly imaging would be within 5 mm of the simulation set-up, no further correction would be made. Set-up corrections larger than 5 mm would be averaged with the shifts of the first 3 fractions, and constitute a new baseline correction for all subsequent fractions. The imaging frequency for Protocol E in this study would be 23%.
Protocol F represents image-guidance every other day with a running mean used to calculate the positional error (alternate day IGRT with running mean). No imaging threshold was applied. The imaging frequency was 50%.
The imaging protocols (B – F) were applied to the raw data (protocol A) to calculate the different 3D vector shifts for target set-up optimization. These shifts or displacements were then compared for different protocols. Shifts were classified, in prone and supine set-up, as vertical (anterior-posterior), lateral (left-right) and longitudinal (cephalic-caudal).
Error calculations were done using systematic and random error formulae Citation[20]. Systematic set-up error was calculated for each patient over all fractions. Random set-up error for each patient is the standard deviation of the systematic error. The average random error is the root mean square of the random set-up errors for each patient.
Results
Analysis of clinical image-guidance data (Protocol A)
Prone patient positioning resulted in average daily set-up correction shifts of 1.2 mm (standard deviation, σ = 1.2 mm), −4.9 mm (σ = 1.2 mm) and 11.5 mm (σ = 1.1 mm) along the x-, y-, and z-axis, respectively. For supine patient position, the respective corresponding x, y, and z shifts were −2.1 mm (σ = 1.1 mm), −2.2 mm (σ = 1.4 mm), and 10.0 mm (σ = 0.9 mm). Both prone and supine patient set-up showed the greatest displacement error in the z-direction (anterior-posterior), with x- and y-directions having relatively smaller shifts. Mean absolute vertical (z-direction) shifts in prone position were 10.5 mm (σ = 3.9); the corresponding shift for supine positioning was 11.4 mm (σ = 5.3 mm). The 3D vector of prone set-up optimization shifts was 15.4 mm (σ = 4.1 mm); the respective vector length for supine position was 12.9 mm (σ = 4.9 mm). Although the systematic set-up error for prone set-up in y-, and z-direction was greater than for supine patient position, random set-up errors were smaller along all room axes for prone set-up than for supine patient position (see also and , and ).
Figure 2. Comparison of corrective shifts along the x-, y-, and z-axes, and 3D vector shifts for prone and supine patient set-up.
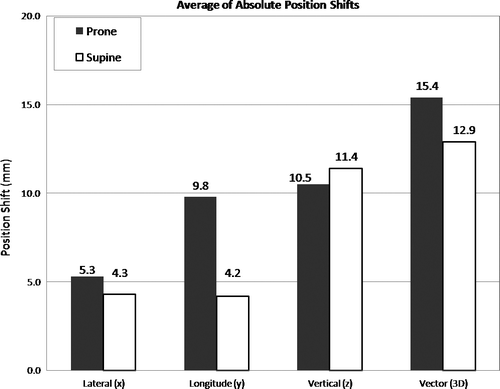
Table II. Systematic and Random errors for Relative and Absolute shifts derived from clinical daily online image guidance for prone and supine patient positioning.
Analysis of various image-guidance scheduling protocols
All image-guidance protocols applied were capable of reducing daily patient set-up errors to various degrees compared with pre-correction set-up errors from the clinical dataset where daily initial set-up was aligned to simulation skin marks, without adjustments for a systematic set-up error component. summarizes the respective length of the average 3D vector shift of residual daily set-up errors and also the percentage of daily alignments with set-up errors exceeding 10, 15 and 20 mm, respectively. It is noted that both NAL protocols provide average 3D set-up error improvements of up to 66% and 51%, for prone and supine patient position, respectively. Increasing the initial imaging frequency from 3 to 5 image-guidance session provided a minor incremental benefit in the present analysis. Comparing systematic and random residual set-up errors between the NAL3 and NAL5 protocols, reveals relative further reduction by up to 14%. While protocols C (NAL5) and protocol D (weekly imaging) have nominally the same imaging frequency, assuming a 30 fraction treatment course, the NAL5 protocol yielded superior residual set-up error benefits largely for patients in prone treatment set-up, whereas the two protocols provided arguably comparable benefits for patients in supine treatment position. This finding confirms the effectiveness of NAL protocols to correct for systematic error dominated target set-up variations, along with a vulnerability for random set-up changes occurring during later phases of the treatment course, as observed more frequently and to a larger degree in supine set-ups than for prone set-ups. As documented in and , both systematic and random components of the set-up error increased for both prone as well as supine patient position beyond fraction 20. The fact that the NAL5 protocol applies early treatment course systematic set-up corrections throughout the entire subsequent treatment course, while a weekly imaging protocol may fail to fully adjust for systematic set-up errors early on, but can catch up with such changes later in the treatment course, indicates the particular limitations of either IGRT protocol. Combining both NAL and weekly imaging (Protocol E) yielded benefits over either strategy alone for both prone and supine patient position. Conduct of image-guidance on alternating treatment days (Protocol F), with offline analysis and running mean set-up adjustments, not only yielded the second highest imaging frequency (50%) but also the most pronounced residual set-up error reduction, whereas not to a level smaller than 2 mm as would be a reasonable to expection resulting from daily online image-guidance.
Discussion
The present study analyzed the impact of prone versus supine patient positioning on daily set-up accuracy of radiation target volumes in close anatomical relationship with the pelvic bony anatomy. Thus, all data presented reflect in essence the inter-fraction variability of bony skeletal patient set-up. Shift of target volumes relative to the patients bony anatomy had not to be considered, this stands in clear contrast to a number of studies researching the impact of prone or supine patient set-up for prostate cancer, a disease in which the targets location may not reflect the patient's bony set-up on any given treatment day Citation[7], Citation[9], Citation[10].
Based on clinical experience and patient feedback, it would have been expected to find supine patient set-up more consistent with possibly less systematic, but certainly lesser random set-up errors, when compared with prone set-ups. Consistent with this expectation, we observed larger systematic set-up errors when placing patients prone on a belly board. This finding confirms data reported previously for repositioning accuracy of prostate and gynecological tumors Citation[1], Citation[7], Citation[10]. However, we were surprised to find supine set-up as being related with larger random set-up errors than prone set-up. This finding was certainly unexpected, and provides for some related, albeit novel findings in the context of schedule, or protocol of image-guidance employed.
While all patients who's data confound the basis of this comparative analysis were treated under daily image-guidance using volumetric mega-voltage CT imaging, and were consistently aligned by laser set-up to skin marks, this concept led to the preservation of relatively large systematic set-up errors over the course of radiation therapy delivery. Systematic set-up errors in AP direction of larger than 10 mm raise the question of cause. We hypothesize that systematic table sag of the TomoTherapy HiArt unit is at least partially responsible for this finding. In a recent report, the MD Anderson, Orlando group characterized the systematic sag on their unit as 8 mm Citation[16]. Additionally a systematic supero-inferior table shift of 2 mm was observed. Assuming comparable systematic table sag on the San Antonio unit, systematic set-up errors in AP direction would have been similar or even smaller than systematic set-up errors observed along the other room axes.
Explaining the surprisingly large random set-up errors observed for supine patient set-up may be less straightforward. While daily set-up was always performed to skin marks or tattoos, it may be conceivable that supine patient set-up confers larger variability or mobility of the patient's skin, leading to an increase in random set-up variability. A standardized patient set-up protocol to a fixed table height may reduce the residual AP set-up variability, a factor that was not studied here. However, this strategy would still not address the observed random set-up errors along the remaining room axes, which were consistently larger in patients in supine vs. prone position.
Besides the fact that any image-guidance strategy would reduce the residual set-up errors, the key finding in the present study was, that protocols that predominantly correct for systematic set-up errors that are manifest early in the treatment course, benefit patients with prone set-up more than patients with supine set-up. The larger random errors associated with supine set-up would require higher frequency image-guidance, afforded by protocols with some initial (NAL like) systematic set-up component correction and continued imaging adjustment over the entire course of treatment. For treatment courses exceeding 20 fractions, distributed imaging protocols may provide incremental benefits. Such a protocol could constitute to include weekly corrections after initial NAL implementation, here studied as Protocol E. Prospective evaluation could also address two blocks of image-guidance assessments, a NAL assessment at the initiation of therapy, followed by another set of three sequential corrections and re-averaging of set-up correction in the second half or last third of a radiation treatment course.
While lower frequency image-guidance would facilitate the clinical workflow and require lesser clinical resources, the observed residual set-up errors would still confer a risk for target miss if the planning target volume margin strategy would not be accordingly adjusted.
The findings in the present study are consistent with recent findings regarding the use and set-up optimization afforded by various image-guidance protocols for targeting of prostate cancer Citation[16]. Comparable to our findings, higher frequency protocols in the study conferred the highest benefit with respect to residual target set-up error. Similar to findings in the cohort of patients treated in supine position, only high-frequency, actual daily image-guidance, was sufficient to correct for unpredictable, yet clinically unacceptable variations in treatment set-up.
Conclusions
In the present study, prone patient set-up was associated with a larger systematic set-up error but smaller random set-up errors when compared to supine patient set-up. Thus, image-guidance protocols controlling largely for systematic set-up errors, such as NAL protocols, benefited patients with prone set-up more than patients in supine position. While supine patient set-up conferred smaller systematic set-up errors, increased random set-up variability would require the use of higher frequency image-guidance. Irrespective of patient set-up, high frequency image-guidance, performed optimally daily with online evaluation yield the highest set-up correction benefit. Declaration of interest: The materials presented in the paper have not been submitted to or published in other peer-reviewed journals. Dr. Martin Fuss was a recipient of research funding, consultant to and speaker for TomoTherapy Inc., Madison, WI. Dr. Niko Papanikolaou is a consultant and speaker for TomoTherapy Inc., Madison, WI, USA. Dr. Papanikolaou is a recipient of research funding from TomoTherapy Inc., Madison, WI.
References
- Olofsen-van Acht M, van den Berg H, Quint S, de Boer H, Seven M, van Soemsen de Koste J, et al. Reduction of irradiated small bowel volume and accurate patient positioning by use of a bellyboard device in pelvic radiotherapy of gynecological cancer patients. Radiother Oncol 2001; 59: 87–93
- Ghosh K, Padilla LA, Murray KP, Downs LS, Carson LF, Dusenbery KE. Using a belly board device to reduce the small bowel volume within pelvic radiation fields in women with postoperatively treated cervical carcinoma. Gyn Oncol 2001; 83: 271–5
- Shanahan TG, Mehta MP, Bertelrud KL, Buchler DA, Frank LE, Gehring MA, et al. Minimization of small bowel volume within treatment fields utilizing customized “belly boards”. Int J Radiat Oncol Biol Phys 1990; 19: 469–76
- Pinkawa M, Gagel B, Demirel C, Schmachtenberg A, Asadpour B, Eble MJ. Dose-volume histogram evaluation of prone and supine patient position in external beam radiotherapy for cervical and endometrial cancer. Radiother Oncol 2003; 69: 99–105
- Adli M, Mayr NA, Kaiser HS, Skwarchuk MW, Meeks SL, Mardirossian G, et al. Does prone positioning reduce small bowel dose in pelvic radiation with intensity-modulated radiotherapy for gynecologic cancer?. Int J Rad Oncol Biol Phys 2003; 57: 230–8
- Koelbl O, Richter S, Flentje M. Influence of patient positioning on dose-volume histogram and normal tissue complication probability for small bowel and bladder in patients receiving pelvic radiation: A prospective study using a 3D planning system and a radiobiological model. Int J Rad Oncol Biol Phys 1999; 45: 1193–8
- Bayley AJ, Catton CN, Haycocks T, Kelly V, Alasti H, Bristow R, et al. A randomized trial of supine vs. prone positioning in patients undergoing escalated dose conformal radiotherapy for prostate cancer. Radiother Oncol 2004; 70: 37–44
- Bentel GC, Munley MT, Marks LB, Anscher MS. The effect of pressure from the table top and patient position on pelvic organ location in patients with prostate cancer. Int J Rad Oncol Biol Phys 2000; 47: 247–53
- Kitamura K, Shirato H, Seppenwoolde Y, Onimaru R, Oda M, Fujita K, et al. Three-dimensional intrafractional movement of prostate measured during real-time tumor-tracking radiotherapy in supine and prone treatment positions. Int J Rad Oncol Biol Phys 2002; 53: 1117–23
- Weber DC, Nouet P, Rouzaud M, Miralbell R. Patient positioning in prostate radiotherapy: is prone better than supine?. Int J Rad Oncol Biol Phys 2000; 47: 365–71
- de Boer HC, van Soernsen de Koste JR, Creutzberg CL, Visser AG, Levendag PC, Heijmen BJ. Electronic portal image assisted reduction of systematic set-up errors in head and neck irradiation. Radiother Oncol 2001; 61: 299–308
- Fuss M, Shi C, Papanikolaou. Tomotherapeutic stereotactic body radiation therapy: Techniques and comparison between modalities. Acta Oncol 2006; 45: 953–60
- Dawson LA, Jaffray DA. Advances in image-guided radiation therapy. J Clin Oncol 2007; 25: 938–46
- Dawson LA, Sharpe MB. Image-guided radiotherapy: Rationale, benefits, and limitations. Lancet Oncol 2006; 7: 848–58
- Shah AP, Langen KM, Ruchala KJ, Cox A, Kupelian PA, Meeks SL. Patient dose from megavoltage computed tomography imaging. Int J Rad Oncol Biol Phys 2008; 70: 1579–87
- Kupelian PA, Lee C, Langen KM, Zeidan OA, Manon RR, Willoughby TR, et al. Evaluation of image-guidance strategies in the treatment of localized prostate cancer. Int J Rad Oncol Biol Phys 2008; 70: 1151–7
- de Boer HC, Heijmen BJ. A protocol for the reduction of systematic patient set-up errors with minimal portal imaging workload. Int J Rad Oncol Biol Phys 2001; 50: 1350–65
- de Boer HC, van Os MJ, Jansen PP, Heijmen BJ. Application of the no action level (NAL) protocol to correct for prostate motion based on electronic portal imaging of implanted markers. Int J Radiat Oncol Biol Phys 2005; 61: 969–83
- de Boer HC, Heijmen BJ. eNAL: An extension of the NAL set-up correction protocol for effective use of weekly follow-up measurements. Int J Rad Oncol Biol Phys 2007; 67: 1586–95
- van Herk M. Different styles of image-guided radiotherapy. Semin Radiat Oncol 2007; 17: 258–67