Abstract
Background. Doubling time (DT) of tumor volume has been widely used to estimate the growth rate of tumors. However, DT gives incorrect estimates of the average growth rate of tumors when the uncertainty of growth rate is considerable. Specific growth rate (SGR) is less affected by uncertainties and is a more relevant parameter. Optimized imaging techniques and prolonged interval between observations can reduce the uncertainty of growth rate estimation. DT is also used for defining changes in tumor marker level. The aim of this study was to compare DT and SGR as measures of growth rate when the uncertainty is negligible. Methods. Mathematical analysis and computer simulations were carried out assuming no uncertainty of growth rate estimation. Data from two previously published clinical studies were assessed by both variables. Results. Due to the non-linear relationship between DT and SGR, using these variables does not give similar results. The variation of DT is not uniformly indicating variations of the growth rate. DT largely overestimates the difference in growth rate of slowly growing tumors and underestimates the difference in growth rate of rapidly growing tumors. On the other hand, SGR uniformly indicates the difference between growth rates throughout all ranges. Quantitative analysis of clinical observations can lead to contradictory results depending on the variable used for growth rate. Conclusion. The growth rate of tumor volume should be expressed by SGR, or percentage increase per unit time, regardless of the level of the uncertainty of growth rate estimation. This conclusion is also valid for changes in tumor marker level, whether it is correlated with the growth rate of tumor volume or not.
Growth rate is a quantifiable character of a tumor, which depends on different factors (e.g., cell type, growth fraction, cell loss rate, and clinical stage) Citation[1], Citation[2]. Tumor volume doubling time (DT) has been widely used for measurement of tumor growth rate since 1956 Citation[3]. Tumor growth rate can also be quantified by the specific growth rate (SGR, %/d) Citation[4]. If the tumor volume (V) is measured at times t1 and t2, SGR can be calculated as:A
The relationship between DT and SGR is:B
Equation B shows that either DT or SGR can be used as measure of tumor growth rate, but the relationship between these variables is not linear. The non-linear relationship between DT and SGR is important from different aspects, e.g., differences in the effect of measurement uncertainties on these variables, or when studying the relationship between growth rate and other variables. We have already studied the effect of the measurement uncertainties of tumor volume on DT and SGR Citation[4]. The uncertainty of tumor growth rate causes an asymmetrical frequency distribution of DT, which in turn causes a deviation of mean DT from the true average growth rate of tumors Citation[4]. SGR was then suggested to be a more suitable measure of tumor growth rate when there is considerable uncertainty in the estimation of growth rate. We also showed that SGR is biologically more relevant than DT to interpret heterogeneities within the tumor tissue, e.g., stromal and tumor cell populations Citation[4].
The uncertainty of the estimated growth rate of a tumor (DT or SGR) depends on the uncertainty of the measurement of tumor volume as well as the time interval between two measurements, i.e. t2-t1 in Equation A Citation[4]. Optimized imaging techniques with higher resolution and prolonged measurement time interval can decrease the uncertainty of the estimated growth rate of tumors. The mathematical differences between DT and SGR, regardless of the level of the uncertainty of growth rate value, have not yet been studied.
Similar to the growth rate of tumor volume, changes in the level of a tumor marker can also be defined using DT or SGR. The mathematical differences between DT and SGR are the same as for the tumor growth rate. The tumor marker level may, however, not be correlated with the tumor volume.
The aim of this study was to compare SGR and DT by analysis of previously published clinical data and computer simulations.
Materials and methods
Variation of DT per unit SGR
According to equation B, the variation of DT with SGR is:C
It shows that the variation of DT per unit SGR is not constant for the whole range of SGR; it quickly decreases with increasing the absolute value of SGR. Variation of DT per unit SGR was plotted for SGR values between −5%/d and +5%/d, corresponding to DT values of −14 days to −∞ and 14 days to +∞, respectively.
Comparison of growth rate between groups of tumors
Two groups of tumors, e.g. affected by different therapeutics, were supposed for comparison including 100 tumors in each group. The average SGR of the first group was assumed to be 1%/d with a standard deviation of 0.5%/d. The second group was assumed to have an average SGR of 1.5%/d with a standard deviation of 0.5%/d. The corresponding DT values of the average SGR are 69 d and 46 d in group 1 and 2, respectively. The variation of growth rate in both groups was assumed to be solely due to the biological differences between tumors with no error in the estimation of SGR or DT values. The SGR values were generated by the random number generation function in Microsoft Excel (Microsoft) and assumed to be normally distributed in both groups. The corresponding DT values were calculated for all tumors in both groups using Equation B. Arithmetic mean and standard deviation of the simulated SGR and DT values were obtained for both groups. The growth rate of two groups was compared with Student's t-test using SGR and DT as measures of tumor growth rate.
In order to compare the frequency distribution of SGR and DT, the computer simulation for group 1 was repeated assuming 10 000 tumors in the group. The frequency distribution of the SGR and the corresponding DT values was then plotted.
Clinical data
Two examples from previously published articles were found that could represent the difference between the results of statistical analyses based on DT and SGR. In the first study, the authors found statistically significant difference between DT of prostate specific antigen (PSA) before and after treatment in each of 9 of 12 patients Citation[5]. Using the signed rank test, they could also detect a significant positive shift in the frequency distribution of DT after treatment. In the present study, the increase rate of PSA level before and after treatment was compared in 12 patients using DT as well as SGR of PSA level by Student's t-test. (Note: The authors of the original article used a method to study PSA level variations in each patient, while in the current study the PSA change in the group of patients was studied). In the second study, the authors examined the DT of serum CA 19-9 level in patients with pancreatic cancer Citation[6]. A significant correlation was found between the DT of the serum level of CA 19-9 and the DT of tumor volume in 11 of 75 patients, where both DT values were available. In the present study, the corresponding SGR values of the DT of the tumor marker level as well as the DT of tumor volume were calculated and the correlation between the two variables was examined.
Results
shows the variation of DT per 1%/d change in SGR based on Equation C. Each %/d of SGR corresponds to a change in DT of 3 days when the SGR is ±5%/d. With decreasing the absolute value of SGR, each %/d change of SGR corresponds to a higher value on the DT scale, with 69 days at ±1%/d and approaching infinity at SGR = 0. A DT of 1 day does not represent the same growth rate when the tumor is slowly growing as when the tumor is rapidly growing (). For a slowly growing tumor with low SGR, DT increases considerably with a slight decrease in SGR. For a rapidly growing tumor with high SGR, DT decreases slightly even with a large increase in SGR. DT understates the growth rate of slowly growing tumors and overstates the growth rate of rapidly growing tumors.
Figure 1. Change in DT per %/d change in SGR, dDT/dSGR, versus SGR. dDT/dSGR changes slightly for rapidly growing tumors, whereas it changes largely for slowly growing tumors and approaches ∞ when SGR approaches zero, i.e., DT approaches ∞.
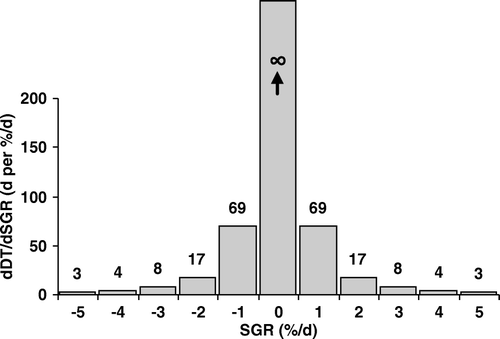
The computer simulation was run for a few times until the generated results could well describe the possible contradictory inferences of the statistical testing of SGR and DT. The selected simulation result of the SGR and corresponding DT values of the two hypothetical tumor groups are presented in . Negative SGR and DT values were allowed and observed in the results. The mean of the simulated SGR values in each group was not identical to, but was close to the assumption made: 1.02%/d versus 1%/d in group 1 and 1.38%/d versus 1.5%/d in group 2, respectively. The standard deviation of the simulated SGR values was also close to the assumption: 0.46%/d in group 1 and 0.50%/d in group 2 versus 0.50%/d for both groups. The mean DT of tumors in groups 1 and 2 was 66 d and 63 d, which was close to the assumption in group 1 (69 d) and was largely different from the assumption in group 2 (46 d). Maximum deviation of DT from the mean DT was much higher than the maximum deviation of SGR from the mean SGR in both groups: 2 227% versus 135% in group 1 and 481% versus 86% in group 2, respectively ().
Table I. Results of computer simulation. SGR and DT values are presented for all tumors in both groups, assuming mean SGR values of 1%/d and 1.5%/d in group 1 and 2, respectively, and a standard deviation of 0.5%/d in both groups.
The difference between the growth rates of two groups was statistically significant when SGR was used (p < 0.0000001) and not significant when DT was used (p > 0.4). With the symmetrical frequency distribution of SGR, the frequency distribution of DT was positively skewed (). The maximum frequency of SGR and DT were located at 1%/d and 60 d-70 d, respectively. Large positive and negative DT values, when SGR approached zero, caused two smaller peaks at negative and positive ends of the frequency distribution of DT. Such values are usually excluded in clinical studies Citation[4].
Figure 2. Frequency distribution of SGR (panel-A) and corresponding DT values (panel-B). The computer simulation was done similar to the simulation for the tumors in group 1 (Table I), but the number of tumors was assumed to be 10 000 in order to obtain a better statistics.
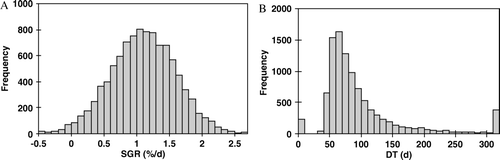
For the clinical studies, the difference between DT of PSA level before and after treatment was not statistically significant (p > 0.1), but the difference between SGR of PSA level before and after treatment was statistically significant (p < 0.002) (). In addition, the correlation between DT of CA 19-9 level and DT of tumor volume was statistically significant (p < 0.0001), but the correlation between SGR of CA 19-9 and SGR of tumor volume was not statistically significant (p > 0.3) ().
Table II. Increase rate of PSA level before and after treatment initiation. DT values were retrieved from a previously published clinical study, Guess et al. (2003) [5], and the corresponding SGR values were calculated (Equation B). The difference between DT of PSA level before and after treatment is not statistically significant (p > 0.1). The difference between SGR of PSA level before and after treatment is statistically significant (p < 0.002). Note: Patient ID is the same ID used in the original paper. Data reprinted with permission of John Wiley & Sons, Inc.
Table III. Growth rate of tumor volume as well as the increase rate of serum CA 19-9 in 11 patients with pancreatic cancer. DT values were retrieved from a previously published clinical study, Nishida et al. (1999) [6], and the corresponding SGR values were calculated (Equation B). The correlation between DT of CA 19-9 level and DT of tumor volume is statistically significant (p < 0.0001). The correlation between SGR of CA 19-9 and SGR of tumor volume is not statistically significant (p > 0.3). Note: Patient no. is the case number used in the original paper. Data reprinted with permission of John Wiley & Sons, Inc.
Discussion
Our results clearly show that selecting a proper variable for tumor growth rate and change in tumor marker level is crucial. Recalculation of the previously published clinical data, as well as computer simulation and mathematical analysis, showed that quantitative analyses can lead to contradictory results depending on the variable used: SGR or DT.
The quantity of tumor growth rate is used in a wide range of studies, e.g., when classifying tumors according to their growth rate Citation[7], or when the correlation between tumor growth rate with other factors is studied, e.g. patient survival Citation[7–10], radionuclide concentration in tumor Citation[10], Citation[11], therapeutic effectiveness Citation[12], and histological characteristics of tumor tissue Citation[2]. The results of quantitative studies based on growth rate and marker level changes may be different by using SGR or DT. Note that we do not discuss clinical data from a medical point of view and we have only used the published DT values and calculated corresponding SGR values. These examples were selected, since contradictory results using SGR and DT could clearly be demonstrated. In general, the theoretical basis for the difference between DT and SGR are valid for any variable that might be measured with DT or SGR.
Tumor growth is a result of cell duplication over time (t). Therefore, the growth rate of a tumor is proportionally related to the number of proliferating cells (and tumor volume, V):D
where SGR is the growth constant. Solving the differential Equation D results in the well known exponential growth model:E
Equations A and E are mathematically identical. SGR is the growth constant of tumor, which is the correct measure of tumor growth rate. When the growth rate of a tumor is measured with DT, the scale of measurement is nonlinearly transformed from the correct scale of SGR to the incorrect scale of DT (Equation B). The SGR of a tumor in clinical observations is in the order of a few tenths %/d to a few %/d Citation[4]. DT does not uniformly indicate the difference between growth rates of tumors throughout all ranges. In addition, DT does not have an absolute zero in its scale; DT is not defined when the growth rate of tumor is zero, i.e., V1=V2 in Equation A and SGR = 0 in Equation B. Therefore, DT is not a proper variable for measurement of tumor growth rate.
Tumors may follow non-exponential growth model, e.g., Gompertzian model. DT and SGR values are estimates of the amount of tumor growth rate in a specific time, or a specific size, calculated from two tumor volume measurements (Equation A). If the growth model is exponential, the growth rate will be constant and can be quantified by a single value (DT or SGR). Non-exponential growth models assume that the growth rate depends on time or size of tumor. The growth model of a non-exponentially growing tumor cannot be explained by a single value, and more parameters are needed to describe the true growth model of each tumor. As a result, the non-exponential growth characteristics of tumors cannot be observed with only two volume measurements. Here, we study the difference between DT and SGR as quantities of tumor growth rate (at any time point or size), and therefore, the results are valid whether the growth rate is constant (exponential growth) or varying by time or size of tumor (non-exponential growth). However, non-exponential growth is not usually observed in natural growth of tumors in clinical studies, because the tumors can be followed only for a short time before the start of treatment.
We assumed that SGR is normally distributed and we showed that DT distribution will then be positively skewed. This result is expected according to the nonlinear relationship between DT and SGR (Equation B). We used this method to demonstrate the asymmetry induced by transforming growth rate values from SGR to DT. It doesn't mean that SGR is normally distributed in clinical observations, e.g., for non-exponentially growing tumors with declining growth rate, the frequency distribution of SGR will deviate from normal distribution according to the extent of growth decline, because larger tumors will have lower growth rates. Such information is more difficult to retrieve from the asymmetric DT distribution. In addition, we have already shown that growth rate variations due to measurement uncertainty that are symmetrically propagated to SGR cause an asymmetrical frequency distribution of DT Citation[4]. Studies have shown that the frequency distribution of DT in clinical observations is positively skewed, similar to , and the logarithmic transformation of DT, which is used by some researchers Citation[8], Citation[9], Citation[13–16], can not fully compensate for the asymmetry of DT distribution Citation[4]. Data for the real frequency distribution of SGR in clinical observations is not available, because DT has been the variable used for quantification of tumor growth rate so far. There is, however, a future opportunity to compare the expected frequency distribution of SGR for different growth models with biological/clinical data to reveal the most appropriate growth model in each case. In the present paper we used Student's t-test in our analyses although the prerequisite is that the parameter is normally distributed. The reason is that in most previously published studies Student's t-test is usually utilized although the true frequency distribution of growth rate is not known Citation[17], Citation[18].
In conclusion, the present study demonstrated that analysis of the growth rate of tumor volume using doubling time can give opposite results to an analysis based on the specific growth rate. The specific growth rate is the more appropriate quantity for tumor growth rate. This conclusion is also valid for quantification of change in tumor marker level.
Acknowledgements
This study was supported by grants from the Swedish Cancer Society (4956) and the King Gustav V Jubilee Clinic Cancer Research Foundation, Göteborg, Sweden.
References
- Holland JF. Cancer medicine. Lea & Febiger, Philadelphia 1993
- Lindell RM, Hartman TE, Swensen SJ, Jett JR, Midthun DE, Tazelaar HD, et al. Five-year lung cancer screening experience: CT appearance, growth rate, location, and histologic features of 61 lung cancers. Radiology 2007; 242: 555–62
- Collins VP, Loeffler RK, Tivey H. Observations on growth rates of human tumors. Am J Roentgenol Radium Ther Nucl Med 1956; 76: 988–1000
- Mehrara E, Forssell-Aronsson E, Ahlman H, Bernhardt P. Specific growth rate versus doubling time for quantitative characterization of tumor growth rate. Cancer Res 2007; 67: 3970–5
- Guess B, Jennrich R, Johnson H, Redheffer R, Scholz M. Using splines to detect changes in PSA doubling times. Prostate 2003; 54: 88–94
- Nishida K, Kaneko T, Yoneda M, Nakagawa S, Ishikawa T, Yamane E, et al. Doubling time of serum CA 19-9 in the clinical course of patients with pancreatic cancer and its significant association with prognosis. J Surg Oncol 1999; 71: 140–6
- Arai T, Kuroishi T, Saito Y, Kurita Y, Naruke T, Kaneko M. Tumor doubling time and prognosis in lung cancer patients: Evaluation from chest films and clinical follow-up study. Japanese Lung Cancer Screening Research Group. Jpn J Clin Oncol 1994; 24: 199–204
- Kuroishi T, Tominaga S, Morimoto T, Tashiro H, Itoh S, Watanabe H, et al. Tumor growth rate and prognosis of breast cancer mainly detected by mass screening. Jpn J Cancer Res 1990; 81: 454–62
- Balmukhanov SB, Turdugulov I, Karibjanova Z, Revesz L. The growth rate of bone sarcomas and survival after radiotherapy with tourniquet-induced hypoxia: A clinical study. Cancer 1982; 49: 1597–604
- Shiomi S, Nishiguchi S, Ishizu H, Iwata Y, Sasaki N, Tamori A, et al. Usefulness of positron emission tomography with fluorine-18-fluorodeoxyglucose for predicting outcome in patients with hepatocellular carcinoma. Am J Gastroenterol 2001; 96: 1877–80
- Duhaylongsod FG, Lowe VJ, Patz EF, Jr, Vaughn AL, Coleman RE, Wolfe WG. Lung tumor growth correlates with glucose metabolism measured by fluoride-18 fluorodeoxyglucose positron emission tomography. Ann Thorac Surg 1995; 60: 1348–52
- Tsunoda A, Shibusawa M, Tsunoda Y, Yasuda N, Koike T. Reduced growth rate of dimethylhydrazine-induced colon tumors in rats. Cancer Res 1992; 52: 696–700
- Spratt JS, Jr. The lognormal frequency distribution and human cancer. J Surg Res 1969; 9: 151–7
- Usuda K, Saito Y, Sagawa M, Sato M, Kanma K, Takahashi S, et al. Tumor doubling time and prognostic assessment of patients with primary lung cancer. Cancer 1994; 74: 2239–44
- Shackney SE, McCormack GW, Cuchural GJ, Jr. Growth rate patterns of solid tumors and their relation to responsiveness to therapy: An analytical review. Ann Intern Med 1978; 89: 107–21
- Spratt JS, Jr, Spratt TL. Rates of growth of pulmonary metastases and host survival. Ann Surg 1964; 159: 161–71
- Lee JY, Kim CK, Choi D, Park BK. Volume doubling time and growth rate of renal cell carcinoma determined by helical CT: A single-institution experience. Eur Radiol 2008; 18: 731–7
- Satkauskas S, Batiuskaite D, Salomskaite-Davalgiene S, Venslauskas MS. Effectiveness of tumor electrochemotherapy as a function of electric pulse strength and duration. Bioelectrochemistry (Amsterdam Netherlands) 2005; 65: 105–11