Abstract
Background: The gross tumor volume (GTV) definition for malignant pleural mesothelioma (MPM) is ill-defined. We therefore investigated which imaging modality is optimal: computed tomography (CT) with intravenous contrast (IVC), positron emission tomography-CT (PET/CT) or magnetic resonance imaging (MRI).
Material and methods: Sixteen consecutive patients with untreated stage I–IV MPM were included. Patients with prior pleurodesis were excluded. CT with IVC, 18FDG-PET/CT and MRI (T2 and contrast-enhanced T1) were obtained. CT was rigidly co-registered with PET/CT and with MRI. Three sets of pleural GTVs were defined: GTVCT, GTVCT+PET/CT and GTVCT+MRI. Quantitative and qualitative evaluations of the contoured GTVs were performed.
Results: Compared to CT-based GTV definition, PET/CT identified additional tumor sites (defined as either separate nodules or greater extent of a known tumor) in 12/16 patients. Compared to either CT or PET/CT, MRI identified additional tumor sites in 15/16 patients (p = .7). The mean GTVCT, GTVCT+PET/CT and GTVCT+MRI [±standard deviation (SD)] were 630.1 cm3 (±302.81), 640.23 cm3 (±302.83) and 660.8 cm3 (±290.8), respectively. Differences in mean volumes were not significant. The mean Jaccard Index was significantly lower in MRI-based contours versus all the others.
Conclusion: As MRI identified additional pleural disease sites in the majority of patients, it may play a role in optimal target volume definition.
Malignant pleural mesothelioma (MPM) is a rare asbestos-related tumor. The prognosis is dismal: the median overall survival times range between 11 and 12 months with 4–6 cycles of cis-/carboplatin and antifolate chemotherapy, which is currently considered the standard of care [Citation1–4]. Nevertheless, the best treatment option remains controversial [Citation1,Citation4–6]. The survival benefit of very aggressive surgical techniques, such as extrapleural pneumonectomy (EPP), is unclear [Citation5]. Furthermore, the interest towards the use of novel systemic agents (i.e. targeted agents or immunotherapy) together with cis-/carboplatin and antifolate chemotherapy in MPM is growing [Citation7]. In particular, the combination of radiotherapy (RT) with immune treatment is appealing, also for MPM [Citation8,Citation9].
In order to investigate appropriately delivered RT in combination with novel agents in the non-surgical setting, RT should be delivered safely to the hemi-thoracic pleura without undue lung damage. Lung-sparing intensity modulated radiation therapy (IMRT) (alone or after P/D) has been proven feasible and safe [Citation10–16].
However, lung-sparing IMRT needs to be further optimized. The optimal gross tumor volume (GTV) definition has not been defined yet. According to the recommendations of the Asbestos Diseases Research Institute, 18F-fluorodeoxyglucose positron emission tomography/computed tomography (18F-FDG-PET/CT) is considered the standard technique for the N- and M-staging in MPM (level of the recommendation A) [Citation17]. However, there is increasing evidence that magnetic resonance imaging (MRI) can be helpful in the pre-operative staging (especially the T-staging) [Citation18,Citation19]. It is therefore likely that, similar to the staging (in particular the T-staging), the integration of MRI in GTV definition will improve the accuracy for tumor delineation (especially the pleural component), hence reducing the probability of geographical miss and increasing the probability of tumor control.
The aim of the present study is to investigate the effect of incorporating MRI findings into the GTV definition in MPM patients treated with hemi-thoracic lung-sparing IMRT. As the standard N-staging consists of a combination of PET/CT and, in some cases, from invasive procedures (e.g. mediastinoscopy), and not MRI, the GTV definition of the lymph nodes was excluded, and is beyond the scope of this article.
Material and methods
Patients
Patients newly diagnosed with biopsy-proven and previously untreated stage I–IV MPM between January 2010 and December 2013 at our institution were retrospectively identified from a prospective institutional database. Eligibility criteria were: to have a staging CT scan with intravenous contrast (IVC), MRI and 18F-FDG-PET/CT, all performed in our institution. Patients with prior pleurodesis were excluded.
The institutional database consisted of 66 patients: 16 of them met the eligibility criteria and were included in the study. The overwhelming majority of non-eligible patients did not undergo staging MRI or PET/CT at our institution (42 patients), whereas eight patients were excluded because they underwent pleurodesis before staging MRI or PET/CT staging.
Patients were staged using the staging system of the International Mesothelioma Interest Group [Citation20].
Thoracic imaging and imaging processing
CT scans with IVC and PET/CT were acquired in free breathing. Patients were scanned in supine position, with both arms raised above their head. MRI was acquired in breath hold and patients were scanned in supine position, with both arms down positioned.
PET/CT scans were acquired with an integrated PET/CT scanner (Biograph TruePoint V; Siemens Healthcare, Erlangen, Germany) including a 40-section CT scanner. Patients received an intravenous injection of 18F-FDG, and rested for 60 minutes before imaging [activity (Bq) = (body weight*4) + 20]. Each examination consisted of a whole body spiral CT scan with IVC followed by a whole body PET acquisition, obtained in the identical transverse plane, and co-registration between the two sets of images was provided. The PET imaging data were reconstructed using an ordered subset expectation maximization algorithm and attenuation corrections derived from CT data. Slice thickness was 3 mm.
MRI scans were performed with a 3-Tesla whole body system (Achieva; Philips Healthcare, Best, The Netherlands) with the manufacturer’s 16-channel phased array torso coil (Sense XL Torso; Philips Healthcare) for signal reception. Non-enhanced T2-weighted turbo spin-echo sequence, a T1-weighted fat-suppressed sequence and a contrast-enhanced T1 sequence were performed in the transverse and coronal plane. Slice thickness was 3 mm.
CT with IVC, PET/CT and MRI scans were retrieved from the hospital images archiving and communication system, exported and saved in an image fusion workstation (MIM Software Inc., Cleveland, OH, USA) for image registration and contouring. Two different sets of rigid co-registrations were created: the first one between CT with IVC and PET/CT and the second one between CT with IVC and MRI. The rigid registration has been performed automatically with the MIM algorithm (an intensity-based algorithm) (MIM Software Inc.). The region of interest encompassed the ipsilateral thorax.
GTV definition and contours’ analysis
Only the GTV of the primary tumor was taken into account and contoured. As the standard N-staging consists of a combination of the functional PET/CT information and, in some cases, from invasive procedures such as mediastinoscopy, and not MRI, the GTV definition of the lymph nodes was excluded, and is beyond the scope of this article.
For each patient, three different GTVs were contoured separately by a radiation oncologist experienced in thoracic oncology, and independently checked by a second radiation oncologist. In case of variation, a consensus was agreed upon:
A CT-based GTV (GTVCT), manually outlined on the CT with IVC scan;
A PET/CT-based GTV (GTVCT+PET/CT), manually outlined on the CT with IVC scan rigidly co-registered to the PET/CT scan;
An MRI-based GTV (GTVCT+MRI), manually outlined on the CT with IVC scan rigidly co-registered to the MRI images (T1, T2 and contrast-enhanced T1-sequences).
The first volume to be contoured was always the GTVCT, followed by the contouring of the GTVCT+PET/CT and GTVCT+MRI. The GTVCT was contoured without knowing the PET/CT scan and the MRI scan data, thus resulting into a blinded interpretation of the raw CT data.
Included into the GTVCT was any pleural thickening (nodular, mediastinal, parietal, circumferential) on the CT scan [Citation21,Citation22].
The GTVCT+PET/CT and GTVCT+MRI were respectively a hybrid CT + PET/CT volume and a hybrid CT + MRI volume. These hybrid volumes were not strictly the union between the CT and the PET or the CT and the MRI target volumes: the initially performed GTVCT was modified on the basis of the findings on PET/CT scans and MRI scans. Included into the GTVCT+PET/CT was any pleural thickening, with any abnormal FDG uptake defined visually. A non-FDG-avid pleural thickening was included into the GTVCT+PET/CT, given the low diagnostic sensitivity of the FDG-PET in defining MPM disease [Citation21]. No SUV threshold was used, as to our knowledge there is no consensus about the most appropriate SUV threshold to discriminate benign from malignant lesions in MPM [Citation23,Citation24]. Included into the GTVCT+MRI was any moderately hyperintense pleural thickening (relatively to muscle on T1 or T2 images) or enhancing after administration of gadolinium-based contrast material.
Statistics
GTVCT, GTVCT+PET/CT and GTVCT+MRI were compared quantitatively and qualitatively.
A quantitative comparison was carried out by investigating the following parameters:
Mean, median, standard deviation (SD) and range of the different GTVs per imaging modality.
Coefficient of variation (COV), a normalized measure of the dispersion of a distribution. This is defined as the ratio of the SD to the mean: COV = SD/mean. Three COVs were obtained: COV GTVCT, COV GTVCT+PET/CT and COV GTVCT+MRI.
Jaccard Index (JI), a measure of the overlap between two GTVs. All possible combinations for JI calculations were exploited, and three pairwise comparisons were thus identified, as follows: GTVCT versus GTVCT+PET/CT, GTVCT versus GTVCT+MRI, GTVCT+PET/CT versus GTVCT+MRI.
Those quantitative parameters were identified according to the paper published by Fotina et al. on the more adequate metrics to report inter-observer variability in target delineation in RT contouring studies [Citation25].
A qualitative comparison was performed through visual assessment. For each patient, three comparisons were made:
PET/CT versus CT with IVC;
MRI versus CT with IVC;
MRI versus PET/CT.
For each comparison, the radiation oncologists identified the patients in whom PET/CT (a) and the MRI (b and c) showed at least one additional tumor site (or additional tumor location within a site) compared to the CT with IVC (a and b) and the PET/CT (c), respectively.
Eight sites were identified: diaphragm, parietal pleura, mediastinal pleura, pericardial pleura, fissural pleura, chest wall, mediastinal fat and costo-phrenic sinus.
The additional tumor site or the additional tumor location within a site were defined either as new site separate (not identified before) or as new locations within a site (i.e. new nodules or greater extent of known tumors).
Statistical analysis was performed using the software package Statistica 11TM (StatSoft Inc., Tulsa, OK). A two-tailed paired t-test was performed to compare continuous variables (once the normality of the distribution of the means was confirmed). A χ2-test was used for categorical variables. Bonferroni correction for multiple testing was applied; a p-value <0.016 (alpha error/3) was considered statistically significant.
Ethics
This was a retrospective contouring study, and patients were diagnosed and treated previously according to standard institutional guidelines. No informed consent was needed according to the policy of the local clinical trial center.
Results
Relevant patients and tumor characteristics are summarized in . Sixteen patients were included, 12 men and four women, nine right-sided and seven left-sided. Their median age was 62 years (range: 64–69 years).
Table 1. Patients and tumor characteristics.
Additional tumor sites (qualitative evaluation)
A detailed description is provided in and and an example is showed in . PET/CT identified additional tumor sites in 12/16 patients, compared to CT-based GTV definition. This new tumor site was the diaphragm in 7/12 cases.
Figure 1. Images in 69-year-old woman with a newly diagnosed left-sided malignant pleural mesothelioma (epithelioid histology). (a and b): Coronal CT (a) and FDG-PET (b) show a diffuse pleural thickening with diffuse FDG-avid zones, with the exception of a portion of parietal pleura indicated by the arrows (in a and b). (c and d): T2-weighted SPAIR image (c) and contrast-enhanced T1-weighted VIBE image (d) show a possible mesothelioma deposit along the whole lateral pleural surface (arrow in c and d): the region that was negative on the CT (a) and FDG-PET (b) appears to be hyperintense in c and d. Moreover, in c and d the pericardium is hyperintense (arrowhead in c and d), while in a and b is slightly thickened and the FDG-avidity is not clearly visible.
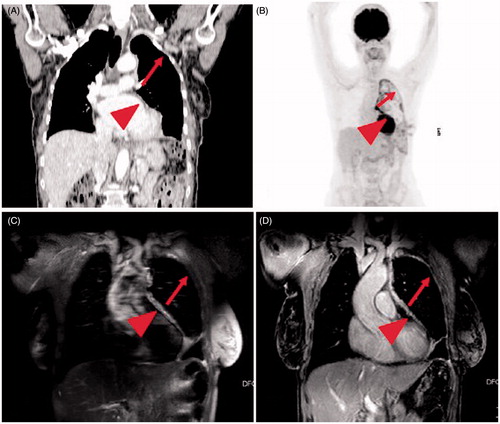
Table 2. Number of patients with additional tumor sites and location details per imaging modality.
Table 3. Visual assessment of the additional tumor sites identified via PET/CT and MRI compared to CT with IVC per patient.
MRI identified additional tumor sites in 15/16 patients compared to either CT scan or PET/CT-based GTV definition. MRI managed to identify more additional tumor sites on CT scans compared to those identified through PET/CT, in particular in the diaphragm (13/15 patients; p = .001), the parietal pleura (11/15 patients; p = .001) and the chest wall (5/15 patients; p = .07) (). When MRI was compared to PET/CT, new tumor sites were: parietal pleura (9/15 patients), diaphragm (7/15 patients) and chest wall (5/15 patients). The number of additional tumor sites identified by MRI on PET/CT compared to those identified by MRI on CT was not statistically significant ().
Quantitative evaluation
An overview of the different GTVs according to the imaging modality is provided in . The mean GTVCT, GTVCT+PET/CT and GTVMRI were 630.1 cm3 (SD: 302.81), 640.23 cm3 (SD: 302.83) and 660.8 cm3 (SD: 290.8), respectively. The differences between the mean volumes were not statistically significant ().
Table 4. GTV Delineation: volume per imaging modality.
COV values of 0.44 were lower in the GTVCT+MRI (compared to 0.49 for GTVCT and 0.48 for GTVCT+PET/CT), indicating a slightly lower dispersion of the values in the MRI-based volumes ().
When examining the concordance between volumes with the three pairwise comparisons, the mean JI ± SD was 0.97 ± 0.02 for GTVCT versus GTVCT+PET/CT, 0.91 ± 0.06 for GTVCT versus GTVCT+MRI and 0.91 ± 0.05 for GTVCT+CT/PET versus GTVCT+MRI. Supplementary Table 1 (available online at http://www.informahealthcare.com) shows the results of the t-test, indicating a lower (and statistically very significant) concordance between MRI-based volume versus non-MRI-based volumes (p = .0007 and p = .0001).
Discussion
MPM is still a devastating disease with only a very limited proportion of patients experiencing long-term survival. As the prognosis remains poor even after a very extensive surgery such as EPP and given the lack of survival benefit of P/D,there is growing interest in combining RT with novel interventions such as immune therapy [Citation8,Citation9]. In the latter case, a therapeutic RT dose to the tumor, together with immune interventions, may lead to a systemic cancer effect. RT should be delivered to an adequately high dose, without harming the underlying lung and the others organs at risk.
Although GTV definition is crucial for optimal IMRT, to the best of our knowledge, this has never been specifically investigated in lung-sparing IMRT for MPM so far. In all published experiences of lung-sparing IMRT in MPM, irradiation of the whole pleura has been performed [Citation10–16]. The clinical target volume (CTV) consisted of the whole pleural space, contoured from the lung apex to the diaphragm insertion (T12-L2). Median doses to the planning target volume ranged from 47 to 50 Gy [Citation10–16]. In all cases, PET/CT was frequently (but not routinely) used to modify the CTV, with the inclusion of any FDG-avid area. None of the abovementioned studies included MRI in the GTV/CTV definition.
However, in diagnostic radiology, MRI is considered the gold standard for the T-staging process (in particular the T-staging for the chest wall and the diaphragm) [Citation1,Citation18,Citation19]. It is therefore likely that, as for the staging (in particular the T-staging), the integration of MRI in GTV definition will improve the accuracy for tumor delineation (especially the pleural component).
In our study, MRI allowed to avoid potential sites of geographical miss in 15/16 CT-based and PET/CT-based GTVs. Those differences in MRI-based GTV delineation were confined to the diaphragm (dome and insertion), the parietal pleura and the chest wall. Although a JI ranging from 0.91 to 0.97 indicates a very high overlap between volumes, the lowest concordance was registered when MRI-based volumes were compared to CT- and PET/CT-based volumes (with a high statistical significance). Differences between volumes were mild and not statistically significant.
Including the MRI in the pleural GTV definition for lung-sparing IMRT for pleural mesothelioma may allow a geographic miss to be avoided (especially in the diaphragm, in the parietal pleura and the chest wall), even if the difference in terms of volumes is small and not significant. In the aforementioned cases, those parts of the tumor that would not be included in the GTV would only receive a very low radiation dose, which might decrease the chance for obtaining tumor control substantially.
Caveats of our study include: the relatively small sample size, the retrospective nature of the study, the absence of gated images and the rigid co-registration between different imaging modalities (sub-optimal fusion). Concerning the registration method, we used a rigid registration method. The use of deformable image registration (DIR) in multimodal registration is far from being without uncertainties [Citation26]. Moreover, MPM is a very challenging tumor scenario, with differences in breathing status between the imaging modalities and the large tumor volumes. This dramatically increases the uncertainties in image registration [Citation26]. To our knowledge, only one group reported on experimental state-of-the-art DIR solutions for multimodal CT-MRI registration in the thoracic region [Citation27]. They concluded that the quantitative results showed ‘a large variation of the target registration for all methods’, showing differences in registration of up to 3 cm in the cranio-caudal direction [Citation27]. Therefore, we used a rigid registration for the CT-MRI co-registration, and visually assessed the accuracy of the contours slice by slice. Moreover, an intrinsic deficiency of the study is the assumption that additional potential tumor sites discovered with imaging actually represent additional areas of disease. Ideally, the gold standard should be represented by a pathologic confirmation, with a complete mapping of the parietal and visceral pleura, and a comparison with CT with IVC, FDG-PET and MRI, but such a study will be very complex (if not impossible) to realize. However, the studies that correlated pathologic and radiologic findings [Citation18,Citation19] were mostly designed to distinguish between resectable (i.e. T3) and unresectable (i.e. T4) tumors, whereas for target volume definition the exact boundaries of the tumor should be known.
Keeping these shortcomings in mind, the integration of T1/T2-weighted MRI into the gross tumor definition would have potentially avoided geographical miss in 15/16 patients. Therefore, this study supports the integration of thoracic MRI in pleural GTV definition for lung-sparing IMRT for pleural mesothelioma.
Supplementary_table_220816.docx
Download MS Word (20.7 KB)Supplementary_material_23082016.docx
Download MS Word (11.6 KB)Disclosure statement
The authors report no conflicts of interest. The authors alone are responsible for the content and writing of this article.
References
- Baas P, Fennell D, Kerr KM, ESMO Guidelines Committee, et al. Malignant pleural mesothelioma: ESMO Clinical Practice Guidelines for diagnosis, treatment and follow-up. Ann Oncol 2015;26S5:v31–9.
- Van Meerbeeck JP, Gaafar R, Manegold C, et al. Randomized phase III study of cisplatin with or without raltitrexed in patients with malignant pleural mesothelioma: an intergroup study of the European Organisation for Research and Treatment of Cancer Lung Cancer Group and the National Cancer Institute of Canada. J Clin Oncol 2005; 23:6881–9.
- Vogelzang NJ, Rusthoven JJ, Symanowski J, et al. Phase III study of pemetrexed in combination with cisplatin versus cisplatin alone in patients with malignant pleural mesothelioma. J Clin Oncol 2003; 21:2636–44.
- Scherpereel A, Astoul P, Baas P, et al. European Respiratory Society/European Society of Thoracic Surgeons Task Force. Guidelines of the European Respiratory Society and the European Society of Thoracic Surgeons for the management of malignant pleural mesothelioma. Eur Respir J 2010; 35:479–95.
- Cao C, Tian D, Park J, et al. A systematic review and meta-analysis of surgical treatments for malignant pleural mesothelioma. Lung Cancer 2014; 83:240–5.
- Bovolato P, Casadio C, Billè A, et al. Does surgery improve survival of patients with malignant pleural mesothelioma? A multicenter retrospective analysis of 1365 consecutive patients. J Thorac Oncol 2014; 9:390–6.
- Reynders K, Illidge T, Siva S, et al. The abscopal effect of local radiotherapy: using immunotherapy to make a rare event clinically relevant. Cancer Treat Rev 2015;41:503–10.
- Marcq E, Pauwels P, van Meerbeeck JP, et al. Targeting immune checkpoints: new opportunity for mesothelioma treatment? Cancer Treat Rev 2015;41:914–24.
- Haas AR, Sterman DH. Malignant pleural mesothelioma: update on treatment options with a focus on novel therapies. Clin Chest Med 2013;34:99–111.
- Minatel E, Trovo M, Polesel J, et al. Tomotherapy after pleurectomy/decortication or biopsy for malignant pleural mesothelioma allows the delivery of high dose of radiation in patients with intact lung. J Thorac Oncol 2012; 7:1862–6.
- Minatel E, Trovo M, Polesel J, et al. Radical pleurectomy/decortication followed by high dose of radiation therapy for malignant pleural mesothelioma. Final results with long-term follow-up. Lung Cancer 2014;83:78–82.
- Rosenzweig KE, Zauderer MG, Laser B, et al. Pleural intensity-modulated radiotherapy for malignant pleural mesothelioma. Int J Radiat Oncol Biol Phys 2012; 83:1278–83.
- Fodor A, Fiorino C, Dell'oca I, et al. PET-guided dose escalation tomotherapy in malignant pleural mesothelioma. Strahlenther Onkol 2011; 187:736–43.
- Feigen M, Lee ST, Lawford C, et al. Establishing locoregional control of malignant pleural mesothelioma using high-dose radiotherapy and (18) F-FDG PET/CT scan correlation. J Med Imaging Radiat Oncol 2011;55:320–32.
- Rimner A, Spratt DE, Zauderer MG, et al. Failure patterns after hemithoracic pleural intensity modulated radiation therapy for malignant pleural mesothelioma. Int J Radiat Oncol Biol Phys 2014;90:394–401.
- Chance WW, Rice DC, Allen PK, et al. Hemithoracic intensity modulated radiation therapy after pleurectomy/decortication for malignant pleural mesothelioma: toxicity, patterns of failure, and a matched survival analysis. Int J Radiat Oncol Biol Phys 2015;91:149–56.
- Van Zandwijk N, Clarke N, Henderson D, et al. Guidelines for the diagnosis and treatment of malignant pleural mesothelioma. J Thorac Dis 2013;5:E254–307.
- Heelan RT, Rusch VW, Begg CB, et al. Staging of malignant pleural mesothelioma: comparison of CT and MR imaging. AJR Am J Roentgenol 1999; 172:1039–47.
- Patz EF, Jr, Shaffer K, Piwnica-Worms DR, et al. Malignant pleural mesothelioma: value of CT and MR imaging in predicting resectability. Am J Roentgenol 1992;159:961–6.
- Rusch VW. A proposed new international TNM staging system for malignant pleural mesothelioma. From the International Mesothelioma Interest Group. Chest 1995; 108:1122–8.
- Nickell LT, Jr.,Lichtenberger JP, 3rd, Khorashadi L, et al. Multimodality imaging for characterization, classification, and staging of malignant pleural mesothelioma. Radiographics 2014;34:1692–706.
- Hallifax RJ, Haris M, Corcoran JP, et al. Role of CT in assessing pleural malignancy prior to thoracoscopy. Thorax 2015;70:192–3.
- Bénard F, Sterman D, Smith RJ, et al. Metabolic imaging of malignant pleural mesothelioma with fluorodeoxyglucose positron emission tomography. Chest 1998;114:713–22.
- Klabatsa A, Chicklore S, Barrington SF, et al. The association of 18F-FDG PET/CT parameters with survival in malignant pleural mesothelioma. Eur J Nucl Med Mol Imaging 2014;41:276–82.
- Fotina I, Lütgendorf-Caucig C, Stock M, et al. Critical discussion of evaluation parameters for inter-observer variability in target definition for radiation therapy. Strahlenther Onkol 2012;188:160–7.
- Brock KK, Dawson LA. Point: principles of magnetic resonance imaging integration in a computed tomography-based radiotherapy workflow. Semin Radiat Oncol 2014;24:169–74.
- Heinrich MP, Jenkinson M, Gleeson FV, et al. Deformable multimodal registration with gradient orientation based on structure tensors. Ann BMVA 2011;2:1–11.