Cardiac mortality in breast cancer patients treated with radiotherapy (RT) has been addressed in the literature and prompted changes in clinical practice [Citation1]. The Quantitative Analysis of Normal Tissue Effects in the Clinic (QUANTEC) effort provided guidelines for normal tissue sparing based on available outcomes data and reported models for dose-volume response [Citation2]. QUANTEC recommended keeping the volume of heart receiving at least 25 Gy (V25) less than 10% to keep the risk of cardiac mortality under 1% [Citation3].
Research quantifying the risk of coronary artery disease or myocardial infarction requires long-term follow-up given the time required to manifest this complication of radiation. The most detailed data to date come from lymphoma and breast cancer patients. As the risks of radiation-induced toxicity are small, research requires large patient populations with detailed dose-volume data. In practice, when outcomes for a large number of patients are available, e.g., a Surveillance, Epidemiology and End Results (SEER) study [Citation4], we lack individual dosimetric data. These studies often rely either on natural randomization and compare cardiac mortality in patients treated for right-sided cancer against left-sided [Citation5] or are based on comparison with the general population [Citation6].
Further important information is provided by large case control studies with long follow-up [Citation7,Citation8] where dosimetric information is estimated from details within treatment charts. Darby et al. found in a large case control study that the rate of major coronary events increased linearly with mean heart dose (MHD), and that the increase started within the first five years after RT and continued for at least 20 years [Citation7]. Two of the more robust studies evaluating cardiac mortality after radiation found a weak volume response [Citation9,Citation10], suggesting that the high dose region of a dose-volume histogram (DVH) drives the risk of cardiac mortality. Therefore, using any single point on a DVH has substantial limitations. The guideline suggested by QUANTEC, V25 <10%, does not protect from 9.9% of the heart volume receiving 50 Gy, which is a typical prescription dose for breast cancer.
In this study we explored QUANTEC guideline compliance for heart dose among left breast RT plans from two institutions to guide routine day-to-day clinical work of dosimetrists and physicians. Furthermore, we evaluated the correlation between predicted cardiac mortality and dosimetric parameters such as V25, D2 (minimum dose to the hottest 2% of the volume), MHD, and maximum dose.
Methods
We retrospectively identified patients who received RT for left breast cancer with tangent fields between 2010 and 2014 at the University of California San Diego (UCSD) and Karolinska University Hospital in Stockholm. Institutional ethics board approvals were obtained from both institutions. Clinical databases were searched for consecutive patients who received RT with tangent fields only to the left breast with a prescribed dose of 50 Gy in 2 Gy fractions or 50.4 Gy in 1.8 Gy fractions. Patients with treatment directed at the supraclavicular, axillary or internal mammary nodes were excluded. Treatment with hypofractionation was the main reason for exclusion, approximately 26% of the initial cohort. In total, 109 patients were included in the final analysis, 44 from Karolinska and 65 from UCSD.
Patients were treated in one of three positions: supine (UCSD and Karolinska), prone, or supine with deep inspiration breath hold (DIBH) (UCSD). At UCSD prone treatment is used for patients with pendulous breasts. All other patients undergo supine simulation, both DIBH and free-breathing, and distance between the heart and beam edge is assessed. Supine DIBH requires multiple breath holds of up to 40 seconds so this technique was typically used in younger women under 60, although preexisting conditions, e.g., lung disease or smoking may preclude use of DIBH. Free-breathing supine (FBS) techniques with or without a heart block were used for all others.
The primary planning objective was to deliver the desired dose to whole breast. Hot spots were kept to <10% and the volume receiving >105% was minimized. The 95% isodose line at a minimum had to cover lung/chest wall interface. Multileaf collimator compensation, dynamic wedge and field-in-field techniques were used to achieve these objectives.
The heart was contoured on a computed tomography (CT) scan by the treating physician according to the RTOG B-39 protocol normal tissue delineation guidelines. The heart block was designed by giving a 2–3 mm margin on the heart contour seen on the beam’s eye view (BEV) if proximity to the tumor bed allowed. As there is no known threshold dose for cardiac injury [Citation4,Citation7] the goal of treatment was to keep the cardiac dose as low as possible by using a ‘no heart in primary beam’ rule whenever possible. With this conservative approach no additional adjustment for other factors, e.g., smoking habits, are made. In addition, UCSD attempted to keep MHD <2 Gy, and Karolinska aimed to keep normal tissue complication probability (NTCP) < 1%. MHD from the boost to the tumor bed was calculated for 15 randomly selected patients and was 0.11 Gy, which is 10% of the dose from tangent fields, 1.11 Gy. The dose from the boost was not included in this analysis. There were 28 patients treated with a FBS technique, 23 supine DIBH and 14 prone from UCSD. All patients at Karolinska were treated with a FBS technique. RT was planned with the Eclipse (Varian Medical Systems, Palo Alto, CA, USA) treatment planning system, and dose was calculated using the analytic anisotropic algorithm accounting for tissue inhomogeneity.
Differential DVHs for the heart were exported from the treatment planning system with a dose bin size of 0.1 Gy. DVHs were further normalized one dose bin at a time to equivalent dose in 2 Gy fractions assuming α/β = 3 Gy. Dosimetric endpoints analyzed included maximum point dose, D2, MHD, and V25. Probability of cardiac mortality, NTCP, for each patient was calculated using the s-model [Citation11]:
where the product is over differential DVH dose bins, with volumes vi receiving dose Di, D50 is the dose corresponding to 50% probability of complication for the whole organ, γ is maximum normalized slope and parameter s describes relative seriality of the organ. Model parameters were set to values obtained specifically for breast cancer patients: D50=52.4 Gy, γ = 1.28 and s = 1.00 [Citation10]. Linear and non-linear correlations between individual dosimetric endpoints and the probability of toxicity were assessed with Pearson’s and Spearman’s rank order correlation coefficients, respectively.
Results
shows DVHs for patients treated at UCSD. DVHs were depicted pictorially with a logarithmic scale to make differences in the low volume region more discernable. DVHs for two patients exhibited pronounced tails for doses >20 Gy because of surgical cavity location. MHD was always under 4 Gy (). D2 varied over a wide range for patients treated with the FBS technique at both UCSD and Karolinska, but was always below 10 Gy for patients treated prone or DIBH (). Median maximum point dose for patients at UCSD was 29.9 Gy (range 4.5–50.9 Gy) for those treated with the FBS technique, 6.4 Gy (9.4–43.1 Gy) for those treated with DIBH, and 3.9 Gy (7.7–42.4 Gy) for those treated prone. The median maximum point dose for patients treated at Karolinska was 49.1 Gy (4.9–51.5 Gy). For all patients full compliance with the QUANTEC recommendation of V25 <10% was observed and in fact V25 was less than 5% for all patients and <0.5% for patients treated prone or DIBH.
Figure 1. (A) Heart DVH for patients treated at UCSD. (B, C and D) mean heart doses, D2 and V25, for patients treated at UCSD and Karolinska.
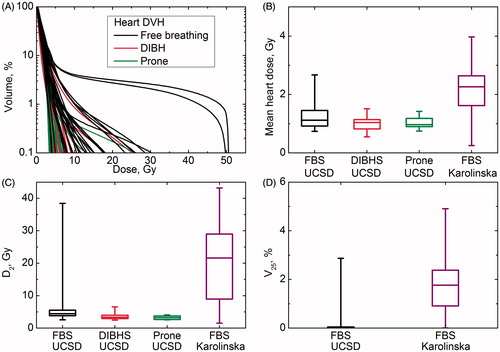
The predicted probability of cardiac mortality was less than 0.01% for all patients treated DIBH or prone. Although none of the patients treated with FBS showed predicted probability of cardiac mortality >1%, this probability exceeded 0.05% in 37 of 72 patients and was above 0.5% in 11 patients.
shows the relationship between the predicted probability of cardiac mortality and considered dosimetric endpoints for patients with NTCP >0.05%. The overall trend was confirmed with Spearman’s correlation coefficient which was 0.95 for both V25 and D2, and 0.76 for MHD, p < .01 in all cases. This correlation was not as pronounced for maximum dose, coefficient equal to 0.38, p = .02. Numerical correlation was moderate for V25 and D2, with R2 = 0.81 (.70 for NTCP >0.25%) and 0.86 (0.90), respectively. This correlation was modest for MHD R2 = .54 (0.37), and no correlation was observed for maximum point dose, R2 = .095 (0.02).
Discussion
This study estimated the risk of cardiac mortality for patients treated with a variety of radiation delivery techniques common to modern practice of radiation oncology. All DVHs fell well within the QUANTEC guideline V25 <10% and all patients had a MHD of less than 4 Gy. Predicted risk of cardiac mortality was less than 1% for all patients. Although our findings align well overall with QUANTEC predictions, the risk of cardiac mortality for some patients was >0.5%. Model parameters in this study came from research in breast cancer [Citation10] patients, however, further validation of model parameters is required.
One important factor to consider with cardiac toxicity prediction relates to the method by which one anatomically defines the organ at risk. This study defined and contoured the entire heart as the organ at risk, which is in compliance with the QUANTEC guidelines, and also reflects common practice. However, all anatomical regions of the heart are clearly not equal in the role they play for the occurrence of toxicity. The majority of cases of cardiac mortality are likely the result of radiation-induced coronary vascular disease in the major arteries supplying the right and left ventricle. Although it is clear that techniques that include coronary arteries in the primary radiation beam lead to a higher risk of stenosis in those vessels [Citation12], there is no clear dose-response data to guide the planning objectives.
shows a broader range for all dosimetric parameters among patients treated at Karolinska and larger MHD for patients treated with FBS. This difference may be related to the ‘no heart in BEV’ approach versus ‘NTCP-driven’ approach. However, at the time of data collection DIBH and prone techniques were not used at Karolinska.
Awareness of cardiac morbidity and mortality has led to changes in clinical practice with the goal of reducing radiation dose to the heart. The use of CT simulation and three-dimensional (3D) treatment planning to better define patient anatomy has increased dramatically over the last 10–15 years. Prone positioning or motion management techniques such as respiratory gating and breath hold techniques further reduce the dose to the heart. Probability of cardiac mortality in excess of 0.1% was seen only in patients treated with FBS. This is not a comparative planning study therefore we cannot conclude that DIBH or prone positioning produces superior heart sparing, however, other research supports this claim [Citation13,Citation14].
A more comprehensive endpoint, i.e., risk of major coronary events was shown by Darby et al. to increase linearly with MHD in breast cancer patients [Citation7]. On one hand, the linear relationship between MHD and toxicity appears inconsistent with the model-predicted weak volume response for cardiac mortality. On the other hand, dosimetric characteristics are often correlated, which means there may not be a conflict between these observations. One of the questions which still needs an answer is the impact of irradiation in specific cardiac subregions.
In summary, this study shows that for studied breast cancer patients compliance with the QUANTEC recommendation of V25 <10% was indeed observed and predicted risk of cardiac mortality was <1%. However, predicted probability of cardiac mortality exceeded 0.5% for 11 of 72 patients treated with a FBS technique. As a result of heart sensitivity to small volumes receiving large doses, no single dose-volume cutoff will serve as a sufficient guideline to keep this risk of cardiac mortality below a desired level. V25 <10% alone does not ensure probability of cardiac mortality below 1%. In fact the probability of cardiac toxicity approached 1% for a few patients despite V25 being below 5%.
Disclosure statement
The authors report no conflicts of interest. The authors alone are responsible for the content and writing of this article.
References
- Giordano SH, Kuo Y, Freeman JL, et al. Risk of cardiac death after adjuvant radiotherapy for breast cancer. J Natl Cancer Inst 2005;97:419–24.
- Marks LB, Yorke ED, Jackson A, et al. Use of normal tissue complication probability models in the clinic. Int J Radiat Oncol Biol Phys 2010;76:S10–S19.
- Gagliardi G, Constine LS, Moiseenko V, et al. Radiation dose-volume effects in the heart. Int J Radiat Oncol Biol Phys 2010;76:S77–S85.
- Darby SC, McGale P, Taylor CW, et al. Long-term mortality from heart disease and lung cancer after radiotherapy for early breast cancer: prospective cohort study of about 300 000 women in US SEER cancer registries. Lancet Oncol 2005;6:557–65.
- Harris EER, Correa C, Hwang W-T, et al. Late cardiac mortality and morbidity in early-stage breast cancer patients after breast-conservation treatment. J Clin Oncol 2006;24:4100–6.
- Hooning MJ, Botma A, Aleman BMP, et al. Long-term risk of cardiovascular disease in 10-year survivors of breast cancer. J Natl Cancer Inst 2007;99:365–75.
- Darby SC, Ewertz M, McGale P, et al. Risk of ischemic heart disease in women after radiotherapy for breast cancer. N Engl J Med 2013;368:987–98.
- Van Nimwegen FA, Schaapveld M, Cutter DJ, et al. Radiation Dose-response relationship for risk of coronary heart disease in survivors of Hodgkin lymphoma. J Clin Oncol 2015;34:235–43.
- Eriksson F, Gagliardi G, Liedberg A, et al. Long-term cardiac mortality following radiation therapy for Hodgkin’s disease: analysis with the relative seriality model. Radiother Oncol 2000;55:153–62.
- Gagliardi G, Lax I, Ottolenghi A, et al. Long-term cardiac mortality after radiotherapy of breast cancer: application of the relative seriality model. Br J Radiol 1996;69:839–46.
- Kallman P, Agren A, Brahme A. Tumour and normal tissue responses to fractionated non-uniform dose delivery. Int J Radiat Biol 1992;62:249–62.
- Nilsson G, Holmberg L, Garmo H, et al. Distribution of coronary artery stenosis after radiation for breast cancer. J Clin Oncol 2012;30:380–6.
- Nissen HD, Appelt AL. Improved heart, lung and target dose with deep inspiration breath hold in a large clinical series of breast cancer patients. Radiother Oncol 2013;106:28–32.
- Mulliez T, Veldeman L, Speleers B, et al. Heart dose reduction by prone deep inspiration breath hold in left-sided breast irradiation. Radiother Oncol 2015;114:79–84.