Abstract
Purpose: To evaluate the treatment-induced toxicity (as primary endpoint) and the efficacy (as secondary endpoint) of stereotactic body radiation therapy (SBRT) in the treatment of mediastinal lymph nodes (LNs) in the so-called no-fly zone (NFZ) in cancers with various histology.
Material and methods: Forty-two patients were retrospectively analyzed. Institutional dose/volume constraints for organs at risk (OARs) derived by published data were strictly respected. The correlation between treatment-related variables and toxicity was investigated by logistic regression, Chi-squared test or Fisher’s exact test. Overall survival (OS), cause-specific survival (CSS), progression-free survival (PFS) and local control (LC) were collected from the follow-up reports. The impact of potential predictive factors on LC, PFS and OS were estimated by Cox proportional-hazard regression.
Results: Median follow-up time was 16 months (range 1–41). Four patients had esophageal G1 toxicity. Ten and six patients had G1 and G2 pulmonary toxicity, respectively. Treatment site and irradiation technique were significantly correlated with G ≥ 2 and G ≥ 1 toxicity, respectively. OS probability at 19 months was 88.3% and corresponded to CSS. LC probability at 16 months was 66.3% (median LC duration: 22 months, range 1–41). Fifteen patients (35.7%) were disease-free at 25 months (median time, range 1–41). The biologically effective dose (BED) and the target dose coverage indexes were significantly correlated with LC.
Conclusions: SBRT can be considered as a safe treatment option for selected patients with oligo-metastases/recurrences in the NFZ, if strict dose/volume constraints are applied.
Introduction
Stereotactic ablative radiation therapy (SBRT) is a high-precision radiation therapy (RT) technique that is typically delivered in few fractions (from 1 to 5) with a high dose per fraction, requiring high accuracy in patient positioning and target definition. This is now a good option for the treatment of clinically inoperable early-stage non-small cell lung cancer (NSCLC), with 2-year local control rates ranging from 80 to 97% [Citation1–4].
Primarily, it has been used in patients with peripherally located lung tumors, since high-dose RT of centrally located tumors, adjacent to critical organs (such as bronchial tree, heart, esophagus, large vessel and lung) may cause severe toxicities [Citation2]. Tumors within a 2-cm radius of the proximal bronchial tree, described as the no-fly zone (NFZ), were therefore excluded from the landmark Radiation Therapy Oncology Group (RTOG) 0236 trial [Citation5] and are currently being studied separately in a phase I/II trial (RTOG 0813) [Citation6], testing SBRT (50–60 Gy in 5 fractions for definitive treatment of early-stage centrally located NSCLC) and aiming to determine the maximum tolerated dose in central lung tumor treatment.
Some single institution series were published so far, suggesting that SBRT of central lesions adjacent to the NFZ appears safe [Citation7,Citation8]. They mainly used the risk-adapted fractionation schemes, in which daily dose-fraction of 7.5 Gy or lower were used, resulting in good local control rate with minimal toxicity. However, a large variation in SBRT scheme exists within community-based practices and academic centers. Of note, there are only few studies focusing on SBRT of tumors or lymph node (LN) metastasis directly abutting the central airway [Citation9–11]. The treatment of such tumors has been considered risky, including a previous report of grade 5 toxicity (according to the Common Terminology Criteria for Adverse Events, CTCAE v4.0) [Citation12–14]. While the optimal SBRT fractionation regimens and doses to normal tissues are agreed for the treatment of peripheral tumors, it appears critical to reach a clinical evidence to define a consensus for centrally located tumors [Citation9,Citation15]. Recurrent LN cancer is considered a sign of systemic disease and consequently it is rarely approached with surgery. The gold standard for LN recurrent cancer is the systemic treatment (i.e., chemo-, endocrine- and biological therapy). Few experiences have been published on SBRT for mediastinal LN lesions [Citation10,Citation11]. In our department, SBRT has been used since 2003 for the treatment of several pathologies in different anatomical sites [Citation16–18] and this long-lasting experience pushed us to investigate its use in the NFZ.
The aim of our retrospective study was to evaluate the feasibility of SBRT as a therapeutic approach for mediastinal LN recurrence in cancers with various histology. The primary endpoint of this study was feasibility, namely the evaluation of toxicity induced by SBRT, and the secondary endpoint was the evaluation of the efficacy of the treatment.
Material and methods
Study protocol
The inclusion criteria for this retrospective study were as follows: 1) patients who underwent SBRT for mediastinal LN recurrence treated in our department between 2012 and 2015; 2) patients who gave consent for use of the data for research purpose; 3) clinical and dosimetric data were available for retrospective analysis. The study was part of the ongoing research program on stereotactic radiotherapy notified to the institutional Ethical Committee (notification no. 93/11).
SBRT procedures
Eligibility criteria for SBRT
Eligibility criteria for SBRT included: age >18 years; prior biopsy and histologic confirmation of cancer; Karnofsky performance score (KPS) ≥70; radiological evidence of mediastinal LN recurrence, described as an increase in size on 2 successive computed tomography (CT) and/or positive at fluorine-18 fludeoxyglucose positron emission tomography (18F-FDG PET) scan; size of the lesions between 1 and 6 cm; written informed consent to the treatment.
Tumor location
The mediastinal LN metastases were classified according to regional LN map, published by the International Association for the Study of Lung Cancer (IASLC) [Citation19]. The mediastinal LNs were divided into 7 zones including 14 different LN stations: supraclavicular zone, upper zone (superior mediastinal LN), aortopulmonary zone, subcarinal zone, lower zone (inferior mediastinal LN), hilar and interlobar zone (pulmonary LN) and peripheral zone (pulmonary LN). The distance between gross tumor volume (GTV) and trachea was also considered as a tumor location descriptor.
Treatment delivery
The simulation CT was performed with patient lying supine on Posirest™ (CIVCO Medical Solution, Orange City, IA, USA), with arms raised above the head, with head standing on a headrest and legs extended. CT scans were acquired on a GE Light Speed (GE Medical System, Fairfield, CT, USA) with voxel resolution of 0.94 × 0.94 × 2.5 mm. Two CT scans were acquired in free-breathing modality, before and after the injection of iodine contrast agent. Treatment plans were performed on Iplan® version 4.5 (BrainLab AG, Feldkirchen, Germany), and consisted of 1–3 non-coplanar 6 MV conformal dynamic arcs or multiple coplanar 6 MV intensity-modulated radiotherapy (IMRT) fields. Treatments were delivered with the VERO system (Mitsubishi Heavy Industries Ltd., Japan and BrainLab AG, Feldkirchen, Germany) every other day. Before each treatment session, the patient was clinically evaluated in terms of general conditions and pre-medicated with drugs (i.e., dexamethasone or paracodine). Patients were positioned with the aid of the optoelectronic system ExacTrac (BrainLab AG, Feldkirchen, Germany) and the target was localized by means of a kV-cone-beam CT. The adequacy of the image fusion between the cone-beam CT and the simulation CT was evaluated by the radiation oncologist.
Target volume, irradiation dose and dosimetric constraints
The GTV was delineated on axial CT images acquired with contrast agent. Clinical target volume (CTV) corresponds to GTV. Planning target volume (PTV) was obtained as a 5 mm isotropic expansion of the GTV. The planning objective was 95% isodose curve covering the 95% of the PTV.
Different fractionations and then different cumulative doses were given according to the size of the lesions, their location and their proximity to organs at risk (OARs). For the definition of tolerated doses of the OARs, we referred to Timmerman [Citation20], and the used constraints are summarized in .
Table 1. Patient and treatment characteristics.
The considered OARs were lungs, heart, esophagus, trachea and main bronchi and were delineated on axial CT images acquired without contrast agent. Trachea and main bronchial tree were contoured including the organ wall and considered as a single structure with the same dosimetric constraint.
In selected cases, the PTV coverage was decreased in order to respect the dose constraints to OARs. In order to compare the dose to the OARs across the various fractionation schemes, all treatment schedules were converted into biologically effective dose (BED) using , where D is the total dose, d is the dose per fraction and α/β was considered equal to 3 Gy for OARs and to 10 Gy for tumor.
Post-treatment evaluation
Generally, after the end of RT, a chest CT with contrast agent injection was performed every 3 months. When indicated, or in ambiguous cases, 18FDG-PET/CT was also performed. Due to the heterogeneity of histology and stage of the treated patients, the follow-up scheme and the required examinations varied largely.
Pulmonary and esophageal toxicity were scored according to the CTCAE v4.0. [Citation12]. For pulmonary acute and late toxicity, symptoms such as cough, dyspnea, hemoptysis, hoarseness, dysphonia were evaluated. Pain, altered gastrointestinal function and dysphagia were considered for esophageal toxicity evaluation.
Toxicity developing in the first 3 months was considered acute.
Tumor response was evaluated as changes in maximal tumor diameter on axial CT image and determined by Response Evaluation Criteria in Solid Tumors (RECIST, mono-dimensional) [Citation21]. In the evaluation of local tumor control, stable disease, partial response and complete response were defined as tumor response.
Data collection and statistical analysis
The primary endpoint of this study was the evaluation of toxicity induced by SBRT for the treatment of mediastinal LN metastases. The evaluation of the efficacy of the treatment was the secondary endpoint.
Overall survival (OS), cause-specific survival (CSS), progression-free survival (PFS), and local control (LC), along with their time of onset, were collected for each patient from the follow-up reports available. Clinical and dosimetric factors potentially related to the evaluation of the endpoints were collected as well. In particular, the clinical factors were: treatment site, irradiation technique (Dynamic Arc or IMRT), systemic treatments (chemo- or hormono-therapy administered before, during or after SBRT), previous irradiation.
The dosimetric parameters were: number of fractions, dose per fraction, total dose and BED; GTV and PTV volumes; GTV maximum diameter; maximum dose to GTV; GTV homogeneity index, defined as HI ; mean and maximum dose to lungs; the volumes of lungs receiving the 10% (V10%) and 5% (V5%) of the prescription dose; the maximum dose and the dose to 5% (D5%) of esophagus; the maximum dose and the dose to 4 cm3 (D4cc) of trachea; the distance between the GTV and the main close OARs, such as heart, trachea, spinal cord and esophagus. The lungs were considered as a unique structure, even if both one-lung and two-lung patients were present in our cohort. Lastly, for all patients and all segmented structures, the dose volume histograms (DVHs) were obtained from the treatment planning system.
The correlation between treatment-related variables and toxicity was investigated by logistic regression for continuous variables, Chi-squared test or Fisher’s exact test for categorical variables. The rates of LC, PFS and OS distributions were estimated by Kaplan–Meier survival analysis and Cox proportional-hazard regression.
The systematic analysis of DVHs was performed. The correlation between volume receiving a given dose and toxicity was investigated for esophagus, trachea and lungs. In this analysis, doses were varied between 1 Gy and the maximum dose for each subset of OAR considered (in 1 Gy step); volumes were varied between 1 cm3 and the maximum volume for each subset of OAR considered (in 1 cm3 step for trachea and esophagus, and 10 cm3 step for the lungs). Acute and late toxicities with a cutoff at grade G > 1 were examined. A p value matrix was generated for each OAR to represent the statistical significance of the correlation between each dose-volume point and the toxicity outcome investigated by Fisher’s exact test. DVH regions of potential interest corresponded to a p value <.05. This is a simple approach, already presented in Jereczek-Fossa et al. [Citation22], that allows examining a large amount of data in an automated way.
Results
Patient and tumor characteristics
Forty-two patients treated at the European Institute of Oncology (Milan, Italy) with SBRT from April 2012 to October 2015 for NFZ LN metastasis satisfied the inclusion criteria for this study and were retrospectively analyzed. All patients gave written informed consent for treatment and for use of their anonymous data for research and education purposes. Our series included patients with various histology, not only lung cancer. Consequently, each patient underwent different RT schedules, with or without concurrent chemotherapy according to the stage and disease characteristics. Number of fractions ranged from 3 to 5, dose per fractions varied from 5 to 12 Gy and total dose from 21 to 50 Gy. For 11 patients, this treatment was a second irradiation. The clinical and treatment characteristics of our population, along with the distribution of treated patients and the distance between GTV and the main OARs, are summarized in .
Analysis of toxicity
Median follow-up time was 16 months (range 1–41). Considering esophageal toxicity, the majority of patients (38 out of 42 patients, including all patients who underwent re-irradiation) reported no toxicity. Of the four patients with esophageal toxicity, three patients had acute toxicity G1 (two patients with esophageal pain, one patient with dysphagia for solid foods) and one patient had late G1 toxicity (dysphagia for solid and semisolid foods at 12 months after treatment). No statistically significant correlations were found between clinical/treatment-related characteristics and esophageal toxicity, with the exception of esophagus D5%, that reached the boundary of statistical significance (p = .051).
Regarding the pulmonary toxicity, 26 out of 42 patients (61.9%) reported no toxicity. Ten patients were G1 with five cases of acute and five cases of late toxicity. In particular, three patients with acute G1 toxicity were asymptomatic with actinic pneumonitis at CT images, two patients with cough and mild dyspnea without evidence of actinic pneumonitis at CT images. Four late G1 events were symptomatic with mild cough. Only one patient with late G1 toxicity had actinic pneumonitis at CT images. The remaining six events were G2 with five cases of acute and only one case of late toxicity. Two patients with acute G2 toxicity showed actinic pneumonitis and atelectasis at CT images, three patients had dyspnea and cough without radiological modification. The patient with late G2 toxicity had cough, dysphonia with laryngeal paralysis. No case of G3 or superior toxicity was observed for both reported pulmonary and esophageal toxicity. Even among the 11 patients who underwent retreatment in the thoracic region, there was no increase in observed toxicity. In particular, no case of esophageal toxicity was observed in the re-irradiation subgroup, whereas three and one patients showed G1 and G2 pulmonary toxicity, respectively.
No statistically significant correlations were found between clinical/treatment-related characteristics and pulmonary toxicity, as reported in , considering the threshold of G1 toxicity (G ≤ 1 vs. G ≥ 2), with the exception of the treatment site (p = .036) since the events occurred more frequently in upper zone irradiation (4 out of 6 patients). If subgroups of patients presenting pulmonary toxicity G = 0 and G ≥ 1 were considered, the correlation with irradiation technique was statistically significant (p = .03), with IMRT subgroup showing the higher probability of pulmonary G ≥ 1 toxicity (). In the IMRT subgroup, patients with G0 pulmonary toxicity were 6 out of 14 (43%) whereas in the Dynamic Arc subgroup they were 20 out 18 (71%). However, it must be noted that this difference has little clinical impact as both groups had low and comparable G2 toxicity (14% in both groups).
Figure 1. Cox proportional-hazard regression of lung toxicity, considering G ≥ 1, for different irradiation techniques.
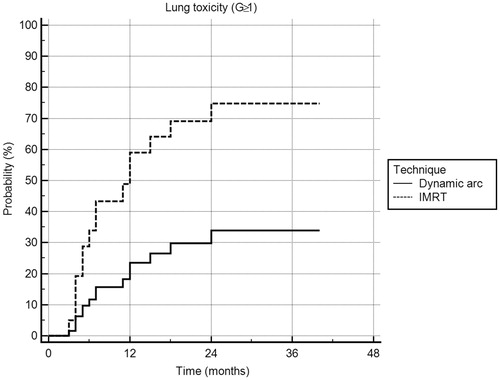
Table 2. Statistical analysis of pulmonary toxicity.
The systematic DVH analysis did not show statistically significant correlations for any of the OARs (lung, esophagus and trachea).
Outcome analysis
OS probability at 19 months was 88.3%. Five patients died at a median time of 19 months after the end of the treatment (range 6–29 months). OS corresponded to CSS, since all the patients died for disease progression. LC probability at 16 months was 66.3% (28/42 patients), and median LC duration was 22 months (range 1–41 months). In 14 patients (33.3%), local progression was observed at a median time of 6 months (range 2–29 months). Fifteen patients (35.7%) were disease free at a median time of 25 months (range 1–41 months). In 27 out of 42 patients (64.3%), disease progression was observed at a median time of 8 months (range 0–37 months). Data were analyzed for correlations between potential predictors and survival endpoints. Our results showed that histology, concomitant treatments, disease stage, disease extent (dimension of GTV and PTV) and GTV homogeneity index did not correlate with any endpoint (OS, CSS, PFS and LC). Interestingly, BED showed a statistically significant correlation with LC (HR = 0.94, 95% CI: 0.90–0.99, p = .002). At a sub-analysis, 3 BED sub-classes were defined: BED ≤40 Gy, 40 Gy < BED <60 Gy and BED ≥60 Gy and our population was stratified accordingly, thus obtaining three sub-populations of 16, 12 and 14 patients, respectively. Statistically significant differences in terms of LC were found between these groups (Chi-squared test, p = .004), with LC increasing with increased BED, as showed in . Moreover, better LC was associated to increased dose-fraction (p = .0001). In particular, at Cox-regression analysis, 8-Gy fractions corresponded to statistically significant higher control (LC: HR = 0.18, CI: 0.0379–0.8223, p = .03). Combining dose-fraction and BED, the statistical significance was decreased, even if a trend persisted for 8-Gy fraction with higher BED (8 Gy per 5 fractions corresponding to BED of 72 Gy with respect to 8 Gy per 3 fractions corresponding to BED of 43.2 Gy, p = .07). Dose coverage indexes showed a statistically significant correlation with LC: mean dose to GTV, mean dose to PTV, maximum dose to GTV, dose to 98% of GTV, dose to 95% of GTV, dose to 50% of GTV indicated that higher dosimetric coverage was associated with higher LC (). As far as PFS was considered, no statistically significant correlation was found with BED or total dose (p values were .49 and .74, respectively). Considering the OS, a comprehensive statistical analysis was not performed due to insufficient follow-up length.
Figure 2. Histogram of local control distribution at increasing BED. Three subpopulations were identified, corresponding to patients treated with BED ≤40 Gy, 40 Gy < BED <60 Gy and BED ≥60 Gy. Local control is defined as 0 (there is not local disease progression, black columns). Otherwise, local control is defined as one if local disease progression was found (grey columns).
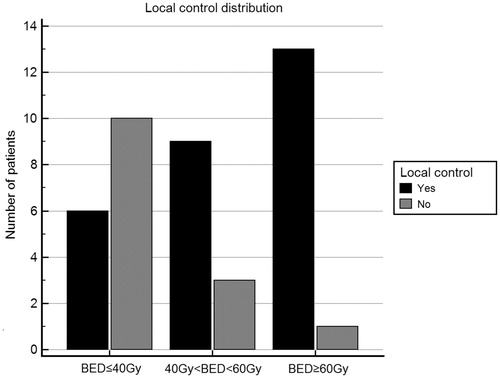
Table 3. Cox proportional-hazard regression analysis of the effect of dosimetric parameters upon local control.
Outcome analysis at 6 months
In order to better assess the LC in the proposed treatment, we examined the outcome of our population at 6 months after the end of the treatment, corresponding to the median time of local progression observed in our population. Only 36 patients had a follow-up >6 months. Thus, six patients were not included in this analysis. In this subpopulation, the median follow-up was 19 months with range 6–41 months. Considering the local response of the treated LN, our results showed that LC was obtained in 25/36 (69.4%), while patients with local progressing disease were 11/36 (30.6%). Radiologic response evaluated according to RECIST criteria at 6 months is presented in .
Table 4. Radiologic response evaluated according to RECIST criteria [21] at 6 months.
Discussion
Our study including 42 patients showed satisfactory local control (about 70% at 6 months) and low toxicity profile after SBRT for NFZ primary or secondary malignancies.
Loco-regional recurrence of NSCLC after surgery occurs in approximately 20% of patients with stage I disease and in up to 50% of patients with stage III disease [Citation23,Citation24]. Also, the nodal mediastinal metastasis from other type of cancer is common and in some cases cause symptoms such as dysphagia, dyspnea, bleeding, dysphonia, vessel compression, neurological symptoms. For this reason, symptomatic RT may be indicated.
Generally, the treatments with SBRT are short and with low toxicity profile. The proximity to critical structures has limited the use of SBRT for mediastinal LNs. For this reason, this type of treatment has always been a challenge for the clinicians.
The main limitations of this study are its retrospective nature, the inclusion of heterogeneous groups of patients including different primary tumors, different therapeutic intent (curative vs. palliative), different disease extent, inhomogeneous RT history (some patients already underwent RT), fractionation regimens and eventual concomitant systemic therapies. For this reason, we focused the attention on treatment feasibility, thus analyzing toxicity results, rather than efficacy with the OS evaluation. The analysis of LC was performed with a particular focus on the 6-month situation. Our results must be intended as hypothesis generating and further prospective analysis should be performed to infer definite conclusions.
The toxicity profile observed was low and in line with the literature data [Citation25]. In our patient series, IMRT was preferred if the PTV was near to OARs, such as esophagus or heart, at the expenses of a higher irradiation of the lung causing increased G1 toxicity. It is worthwhile to observe that, due to the heterogeneity in patient population, BED ≥100 Gy was rarely administered (7% of cases), which might explain the good toxicity profile observed in this patient series.
Treatment-related deaths were not observed in our population. In all cases of toxicity, G2 symptoms were solved with medical treatments and there was no need for hospitalization. In all cases, the strict DVH constraints were respected. Interestingly, new constraints based on the dose-response models providing quantitative estimates of risk as a function of dose and volume have been recently proposed [Citation26–28]. In the future studies these new constraints need to be validated.
The correlation between toxicity and DVH was systematically analyzed in our series. Every point of the dose-volume area was put into correlation with toxicity results, in order to identify statistically significant dose-volume points that could potentially predict toxicity. In our dataset, no correlation between dose-volume points and toxicity was found. This could be due to both insufficient sample size, in term of total number of patients and total number of high-grade toxicity events, and to the safety of the constraints used for treatment planning.
Different from previously presented series [Citation29], no clear association of SBRT schedule or chemotherapy with esophageal toxicity was observed. However, we have to consider that the median BED in our dataset is low. In our analysis, we did not observe different toxicity tendencies based on treatment site as reported in the few data available in literature. In particular, differently from previously presented series [Citation11], we found no correlation between the subcarinal LNs as treatment site or patients who underwent re-irradiation and toxicity. Two patients were treated for subcarinal LN and it was the first RT at mediastinal LNs. They received 25 Gy and 35 Gy in 5 fractions, corresponding to BED of 37.5 and 59.5 Gy, respectively. Considering the esophageal and pulmonary toxicity, one patient had acute G1 toxicity characterized by esophageal pain and one patient presented acute G1 pulmonary side effects, namely cough and atelectasis. In these patients, we have not scored late esophageal and pulmonary toxicity. However, it can be observed that in our population, 17 out of 42 patients (40.5%) have metastatic tumors that do not originate from lung primary tumors. Therefore, it could be expected that these patients generally have better respiratory function as compared to a population of patients with lung cancer only.
As expected, we obtained a higher LC in the group of patients treated with BED >60 Gy. However, it should be considered that, at present time, no data to support this finding were available in clinical literature, since all the available data refer to primary tumors and not to LN metastases. Similarly, Onishi et al. described a cohort of 245 patients from multiple Japanese institutions and found that a BED of 100 Gy significantly improved both LC (92% vs. 74%, p < .05) and 3-year OS (88% vs. 69%, p < .05). In addition, LC was not improved further if patients received a BED higher than 120 or 140 Gy [Citation30]. Additional studies in which central and peripheral tumors were analyzed together, also found that a BED over 100 Gy improved LC [Citation14,Citation31]. However, few data are available for LNs metastatic lesion, and even less studies discuss which range of BED has to be used for patients with the characteristics of our population.
It is interesting to note that, in our series, 8-Gy fractions corresponded to statistically significant higher LC. This dose is very similar to the treatment schedule used for central lung lesions in other studies [Citation25] and can support the hypothesis that this dose can be considered as ablative threshold dose.
Based on our data, we can assert that SBRT is a safe treatment and can be considered as an option for a selected patient with oligo-metastases or oligo-recurrences in NFZ. The dose constraints for OARs employed in our series should be further validated by prospective studies.
Hypofractionation and re-irradiation can be considered also in the NFZ, if precise treatment with strict dosimetric constraints is used.
Further studies are needed to evaluate confounding parameters, including age, performance status, histology, primary tumor site and genetic differences between the primary tumor and metastases. Further investigation is warranted to identify the patients who benefit most from this treatment modality. Considering the scarcity of data in the clinical literature about this type of treatment, this work gives evidence of its feasibility and it demonstrates that the use of a local treatment before or concomitantly to systemic treatment could be useful to increase the LC with a low toxicity profile.
Supplemental Material
Download MS Word (15.7 KB)Acknowledgments
The sponsors did not play any role in the study design, collection, analysis and interpretation of data, nor in the writing of the manuscript, nor in the decision to submit the manuscript for publication.
Disclosure statement
No potential conflict of interest was reported by the authors.
Additional information
Funding
References
- Fakiris AJ, McGarry RC, Yiannoutsos CT, et al. Stereotactic body radiation therapy for early-stage non-small-cell lung carcinoma: four-year results of a prospective phase II study. Int J Radiat Oncol Biol Phys. 2009;75:677–682.
- Timmerman R, Paulus R, Galvin J, et al. Stereotactic body radiation therapy for inoperable early stage lung cancer. JAMA. 2010;303:1070–1076.
- Falkson CB, Vella ET, Yu E, et al. Radiotherapy with curative intent in patients with early-stage, medically inoperable, non-small-cell lung cancer: a systematic review. Clin Lung Cancer. 2017;18:105–121.
- Loganadane G, Martinetti F, Mercier O, et al. Stereotactic ablative radiotherapy for early stage non-small cell lung cancer: a critical literature review of predictive factors of relapse. Cancer Treat Rev. 2016;50:240–246.
- Radiation Therapy Oncology Group RTOG 0236. A Phase II trial of stereotactic body radiation therapy (SBRT) in the treatment of patients with medically inoperable stage I/II non-small cell lung cancer. 2014. Available from: http://www.rtog.org/ClinicalTrials/ProtocolTable/StudyDetails.aspx?study
- NRG Oncology RTOG 0813. Seamless Phase I/II study of stereotactic lung radiotherapy (SBRT) for early stage, centrally located, non-small cell lung cancer (NSCLC) in medically inoperable patients. 2014. Available from: http://www.rtog.org/ClinicalTrials/ProtocolTable/StudyDetails.aspx?study
- Chang JY, Balter PA, Dong L, et al. Stereotactic body radiation therapy in centrally and superiorly located stage I or isolated recurrent non-small-cell lung cancer. Int J Radiat Oncol Biol Phys. 2008;72:967–971.
- Modh A, Rimner A, Williams E, et al. Local control and toxicity in a large cohort of central lung tumors treated with stereotactic body radiation therapy. Int J Radiat Oncol Biol Phys. 2014;90:1168–1176.
- Song SY, Choi W, Shin SS, et al. Fractionated stereotactic body radiation therapy for medically inoperable stage I lung cancer adjacent to central large bronchus. Lung Cancer. 2009;66:89–93.
- Wang HH, Zaorsky NG, Meng MB, et al. Stereotactic radiation therapy for oligometastases or oligorecurrence within mediastinal lymph nodes. Oncotarget. 2016;7:18135–18145.
- Meng MB, Wang HH, Zaorsky NG, et al. Clinical evaluation of stereotactic radiation therapy for recurrent or second primary mediastinal lymph node metastases originating from non-small cell lung cancer. Oncotarget. 2015;6:15690–15703.
- Common Terminology Criteria for Adverse Events and Common Toxicity Criteria. National Cancer Institute Cancer Therapy Evaluation Program. 2009. Available from: http://ctep.cancer.gov/protocolDevelopment/electronic_applications/ctc.htm
- Corradetti MN, Haas AR, Rengan R. Central-airway necrosis after stereotactic body-radiation therapy. N Engl J Med. 2012;366:2327–2329.
- Rowe BP, Boffa DJ, Wilson LD, et al. Stereotactic body radiotherapy for central lung tumors. J Thorac Oncol. 2012;7:1394.
- Chaudhuri AA, Tang C, Binkley MS, et al. Stereotactic ablative radiotherapy (SABR) for treatment of central and ultra-central lung tumors. Lung Cancer. 2015;89:50–56.
- Jereczek-Fossa BA, Fanetti G, Fodor C, et al. Salvage stereotactic body radiotherapy for isolated lymph node recurrent prostate cancer: single institution series of 94 consecutive patients and 124 lymph nodes. Clin Genitourin Cancer. 2017;15:e623.
- Orecchia R, Surgo A, Muto M, et al. VERO® radiotherapy for low burden cancer: 789 patients with 957 lesions. Ecancermedicalscience. 2016;10:677.
- Garibaldi C, Piperno G, Ferrari A, et al. Translational and rotational localization errors in cone-beam CT based image-guided lung stereotactic radiotherapy. Phys Med. 2016;32:859–865.
- El-Sherief AH, Lau CT, Wu CC, et al. International association for the study of lung cancer (IASLC) lymph node map: radiologic review with CT illustration. RadioGraphics. 2014;34:1680–1691.
- Timmerman RD. An overview of hypofractionation and introduction to this issue of Seminars in Radiation Oncology. Semin Radiat Oncol. 2008;18:215–222.
- Eisenhauer EA, Therasse P, Bogaerts J, et al. New response evaluation criteria in solid tumours: revised RECIST guideline (version 1.1). Eur J Cancer. 2009;45:228–247.
- Jereczek-Fossa BA, Ciardo D, Ferrario S, et al. No increase in toxicity of pelvic irradiation when intensity modulation is employed: clinical and dosimetric data of 208 patients treated with post-prostatectomy radiotherapy. Br J Radiol. 2016;89:20150985.
- Trodella L, Trodella L, Granone P, et al. Adjuvant radiotherapy in non-small cell lung cancer with pathological stage I: definitive results of a phase III randomized trial. Radiother Oncol. 2002;62:11–19.
- Dautzenberg B, Arriagada R, Chammard AB, et al. A controlled study of postoperative radiotherapy for patients with completely resected non small cell lung carcinoma. Groupe d’Etude et de Traitement des Cancers Bronchiques. Cancer. 1999;86:265–273.
- Lagerwaard FJ, Haasbeek CJ, Smit EF, et al. Outcomes of risk adapted fractionated stereotactic radiotherapy for stage I non-small-cell lung cancer. Int J Radiat Oncol Biol Phys. 2008;70:685–692.
- Duijm M, Schillemans W, Aerts JG, et al. Dose and volume of the irradiated main bronchi and related side effects in the treatment of central lung tumors with stereotactic radiotherapy. Semin Radiat Oncol. 2016;26:140–148.
- Xue J, Kubicek G, Patel A, et al. Validity of current stereotactic body radiation therapy dose constraints for aorta and major vessels. Semin Radiat Oncol. 2016;26:135–139.
- Kimsey F, McKay J, Gefter J, et al. Dose-response model for chest wall tolerance of stereotactic body radiation therapy. Semin Radiat Oncol. 2016;26:129–134. doi: 10.1016/j.semradonc.2015.11.003
- Stephans KL, Djemil T, Diaconu C, et al. Esophageal dose tolerance to hypofractionated stereotactic body radiation therapy: risk factors for late toxicity. Int J Radiat Oncol Biol Phys. 2014;90:197–202.
- Onishi H, Araki T, Shirato H, et al. Stereotactic hypofractionated high-dose irradiation for stage I nonsmall cell lung carcinoma. Clinical outcomes in 245 subjects in a Japanese multiinstitutional study. Cancer. 2004;101:1623–1631.
- Taremi M, Hope A, Dahele M, et al. Stereotactic body radiotherapy for medically inoperable lung cancer: prospective, single-center study of 108 consecutive patients. Int J Radiat Oncol Biol Phys. 2012;82:967–973.