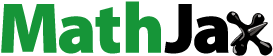
Introduction
Low dose envelope of pencil ion beam has attracted more and more concerns recently, which is located far away from the beam’s central axis and mainly consists of particles undergoing multiple Coulomb scattering, as well as secondary particles produced by nuclear interactions [Citation1–5]. As a result of the low dose envelope, the dose output depends on the size of the irradiation field, which has already been extensively investigated [Citation3,Citation4,Citation6,Citation7]. To the best of our knowledge, the impact of the low dose envelope on the total dose is considerable for high-energy beams, especially at the medium penetration depths for small fields [Citation7,Citation8]. However, for stereoscopic target volumes under various conditions, the dosimetric effect of the low dose envelope for carbon-ion therapy is rarely mentioned, and reports on the relevance of this effect for different analytical dose calculation models in diverse stereoscopic target volumes are also scarce. It has been reported that even small deviations in characterizing the low dose envelope of individual pencil beams may accumulate to a clinically significant dose error when the dose contributions from all beam spots are superimposed in spot scanning technology [Citation4,Citation7]. Therefore, many pencil beam models considering the low dose envelope have been extensively investigated for carbon-ion and proton pencil beams, like double and triple Gaussian models [Citation4,Citation9], which have been applied in some commercial and researched treatment planning systems (TPSs) for proton or carbon-ion therapy. However, the single Gaussian model is still used in many initial versions of TPS, which does not take the low dose envelope into consideration [Citation10]. In our previous work, a novel double Gaussian-logistic model was proposed to depict the lateral dose distribution of carbon-ion pencil beam in water, which could adequately modeled the low dose envelope [Citation8]. The present study was intended to evaluate the dosimetric effect of the low dose envelope for carbon-ion pencil beam on various target volumes and conduct comparison about the calculation accuracy between the single, double and triple Gaussian models and the double Gaussian-logistic one, whilst analyzing in which scenario our newly-proposed beam model has its greatest advantage. This provides a basis for the future application of our novel beam model in TPS for carbon-ion therapy.
Material and methods
Monte Carlo simulation
The feature of the low dose envelope was shown through virtual homocentric spherical target volumes in diameters of 3 cm, 5 cm and 8 cm, located at the penetration depth of 150.5 mm in water tank, and the same-sized spherical targets in diameter of 3 cm at the depths of 114.5 mm, 150.5 mm and 186.5 mm in water irradiated with carbon-ion pencil beams. The targets were all filled with water. These configurations are complex enough to simulate realistic treatment scenarios varying from small to large target volumes in carbon-ion therapy. Based on GATE 7.1/GEANT4 10.01 [Citation11], the optimized therapy beam line of the Heavy Ion Research Facility in Lanzhou (HIRFL) at the Institute of Modern Physics (IMP), Chinese Academy of Sciences [Citation12] was adopted to obtain the three-dimensional dose distributions of carbon-ion pencil beams in water, which could satisfy the requirements on the FWHM of beam spots and dose flatness at the isocentre for the active spot-scanning beam delivery system at HIRFL. Detailed description about the layout of the optimized beam line and the basic simulation settings were mentioned in our previous work [Citation8]. The spot spacings for spot-scanning beam delivery were all set to 3 mm and 4 mm in the lateral and longitudinal directions, respectively. Then, the iso-energy slices in the spherical target volumes were confirmed, which required the pencil beams with 25 energies from 240 MeV/u to 344 MeV/u, covering the range from 102.5 mm to 198.1 mm in water. In each simulation, the initial statistic of carbon ions was 107.
Dose calculation model
Pencil beam algorithm was used in the present work, in which the lateral dose distributions were calculated with the single, double and triple Gaussian models and the double Gaussian-logistic one, respectively. EquationEquation (1)(1)
(1) is the expression of the double Gaussian-logistic model, where x and y are the lateral distances from the pencil beam axis in x and y directions,
and
are the positions of the pencil beam axis in x and y directions, and z is the corresponding depth in water. In addition,
or
and
are the lateral spread and weight. The subscripts represent different parts in the equations, and the sum of the weights of all parts is 1. For the sake of simplicity, the parameters of each model were all obtained by fitting to the MC data, using ‘fminsearch’ code in MATLAB (The Mathworks Inc., USA), which were elaborated in our previous work [Citation8].
(1)
(1)
Dose optimization of spherical target volumes for spot-scanning beam delivery
The iterative least square method was adopted for the optimization of the spot weights in our programing implementation, which has been applied in pion, photon and proton plan optimizations [Citation13] and is also suited for carbon-ion dose optimization. Without considering the biologically effective dose, the purpose of the field optimization was to reach the prescribed physical doses in the target volumes only. The calculated dose deviated from the prescribed physical dose was set within ±5%. If the optimization result met this dose deviation requirement, the iteration was terminated. Otherwise, the iteration continued until the end, which depended on the preset iteration number. 5000 iterations were set in the present work.
The workflow is listed below:
3D carbon-ion pencil beams with various energies in water tank were obtained via the MC simulations;
Parameters of the different beam models were acquired by fitting to the MC data with MATLAB;
The spot weights were optimized for the spherical target volumes with the iterative least square method, based on a certain beam model with the parameters obtained from the step (2);
The ‘simulated’ spherical target volumes were calculated with the optimized spot weights from the step (3) and individual pencil beams from the step (1).
In view of a long simulation time for a three-dimensional target, the simulated dose distributions of single pencil beams with various energies, weighted by the optimized spot weights which were based on a certain beam model in corresponding iso-energy slices, were deemed as the dose distribution across the target volume. This procedure was just a simple imitation about the actual beam delivery according to treatment planning calculated with a certain beam model in TPS.
Results
The average dose deviations between the simulated and planned doses together with standard deviations were counted at the perpendicular planes of the diverse spherical target volumes with the different beam models at various depths along the beam incident axis. The depths for estimating the deviations included the plateau region, the mid-point of the spread-out Bragg peak (SOBP) (the center of the target volume) and the tail region (5 mm behind the spherical target volume). The results are listed in . These deviations are a result of the insufficient description about the low dose envelope, which accounted for a part of the dose away from the beam central axis. It was found that the simulated doses were closer to the planned ones through the calculations using the triple Gaussian model and the double Gaussian-logistic one. Compared with the existing triple Gaussian model, our newly-proposed model was on a par with it and even better in most cases except for a small part of the scenarios marked in bold in the table. Furthermore, the calculation accuracy improvement using the double Gaussian-logistic model compared with the single, double and triple Gaussian models in the center of SOBP for the various scenarios were analyzed and is given in . The data in were all based on the results from . The larger was the value in , the obvious was the advantage of our newly-proposed beam model. Therefore, the superiority of the double Gaussian-logistic model was more obvious for the smaller target volume, especially at the deeper depth.
Table 1. Comparison of the accuracy of calculations with the single (SG), double (DG), triple Gaussian (TG) models and the double Gaussian-logistic (DG-L) one in the plateau, peak and the tail regions for the spherical target volumes in diameters of 3 cm, 5 cm and 8 cm located at the depth of 150.5 mm and for the spherical target volumes in diameter of 3 cm, located at the depths of 114.5 mm, 150.5 mm and 186.5 mm.
Table 2. The calculation accuracy improvement using the double Gaussian-logistic model (DG-L) compared with the single (SG), double (DG) and triple Gaussian (TG) models in the center of SOBP for homocentric spherical target volumes with different diameters and for spherical target volumes in diameter of 3 mm located at various depths.
The dosimetric effect of the low dose envelope can be reflected indirectly via the deviation calculated with the single Gaussian model in . This effect was regular for the same-sized spherical target volumes at the different penetration depths in water on account of only one variable, beam energy. It increased in depth along the beam incident direction. However, due to the diversity of the calculation conditions, such as the number of scan spots and beam energy, there was no obvious pattern about this effect in the center of the SOBP for the concentric spherical target volumes with the various diameters.
Discussion
For the parameters in the beam models with multiple functions, the spot spread of the first Gaussian component is generally calculated by analytical algorithms in TPS such as the Fermi-Eyges theory or the Molière formula [Citation2,Citation14] and Highland formula [Citation15], while the parameters in the remain parts of the model are transported empirically using measurement or MC simulation data. For the sake of simplicity, all the 3 D dose distributions in water obtained from the MC simulations were fitted using the MATLAB tool for a theoretical study in the present work. The parameters of the beam model in TPS are specific for particular therapy facility. Due to unformulated parameters of the double Gaussian-logistic model, database is required for its application, which can be obtained from measurement or simulation. Like the triple Gaussian model, 4 parameters (
) are needed to be stored as the database in the TPS. However, more parameters will lead to more calculation time and memory space, which have been discussed in our previous work [Citation8]. Necessary work will be launched to overcome this defect.
Simultaneous comparison of various beam models in one TPS is difficult to realize now. Thus, manual programing was implemented to make simple treatment plans based on different beam models. As a theoretical study, Monte Carlo (MC) simulation was used to produce a database for carbon-ion spot scanning beam delivery. For the calculation accuracy of the different beam models, comparisons on the dose errors among the different cases calculated with the same beam model are difficult to evaluate, but the dose deviations calculated with the different beam models in the same case are worth being analyzed. For the smaller target volume, the superiority of the double Gaussian-logistic model was more obvious, especially at the deeper depth. However, the precision of calculation for larger target is more important in clinical application. Although the diminution of the deviations calculated with our new model for larger target was not more notable than that for smaller target, the results derived from the new model were absolutely acceptable. In any event, the double Gaussian-logistic model is a good candidate for improving the dose calculation accuracy in TPS.
The present work was all conducted in water but is helpful to comprehend the dosimetric impact of the low dose envelope for carbon-ion pencil beam on various virtual target volumes, even makes ones be aware of the significance of the precise beam model, especially for small target volumes at deeper penetration depths. Nevertheless, inhomogeneous media are often encountered in clinical scenarios, which will be studied in our future work. After a series of theoretical studies, the application of the double Gaussian-logistic model should also be combined with experiments, which will be served as the database in TPS.
Disclosure statement
The authors declare that they have no conflict of interest.
Additional information
Funding
References
- Parodi K, Mairani A, Sommerer F. Monte Carlo-based parametrization of the lateral dose spread for clinical treatment planning of scanned proton and carbon ion beams. J Radiat Res. 2013;54:91–96.
- Bellinzona EV, Ciocca M, Embriaco A, et al. A model for the accurate computation of the lateral scattering of protons in water. Phys Med Biol. 2016;61:N102–N117.
- Sawakuchi GO, Zhu XR, Poenisch F, et al. Experimental characterization of the low-dose envelope of spot scanning proton beams. Phys Med Biol. 2010;55:3467–3478.
- Schwaab J, Brons S, Fieres J, et al. Experimental characterization of lateral profiles of scanned proton and carbon ion pencil beams for improved beam models in ion therapy treatment planning. Phys Med Biol. 2011;56:7813–7827.
- Sawakuchi GO, Titt U, Mirkovic D, et al. Monte Carlo investigation of the low-dose envelope from scanned proton pencil beams. Phys Med Biol. 2010;55:711–721.
- Kusano Y, Kanai T, Yonai S, et al. Field-size dependence of doses of therapeutic carbon beams. Med Phys. 2007;34:4016–4022.
- Li Y, Zhu RX, Sahoo N, et al. Beyond Gaussians: a study of single-spot modeling for scanning proton dose calculation. Phys Med Biol. 2012;57:983–997.
- Zhang H, Dai Z, Liu X, et al. A novel pencil beam model for carbon-ion dose calculation derived from Monte Carlo simulations. Phys Medica. 2018;55:15–24.
- Hirayama S, Takayanagi T, Fujii Y, et al. Evaluation of the influence of double and triple Gaussian proton kernel models on accuracy of dose calculations for spot scanning technique. Med Phys. 2016;43:1437–1450.
- Tommasino F, Fellin F, Lorentini S, et al. Impact of dose engine algorithm in pencil beam scanning proton therapy for breast cancer. Phys Medica. 2018;50:7–12.
- Jan S, Santin G, Strul D, et al. GATE: a simulation toolkit for PET and SPECT. Phys Med Biol. 2004;49:4543.
- Yuanlin Y, Xingguo L, Tingyan F, et al. Monte Carlo simulation study on optimizing the active beam delivery system at HIRFL. Nucl Phys Rev. 2016;33:105–111.
- Lomax A. Intensity modulation methods for proton radiotherapy. Phys Med Biol. 1999;44:185–205.
- Bethe HA. Moliere’s theory of multiple scattering. Phys Rev. 1953;89:1256–1266.
- Highland VL. Some practical remarks on multiple scattering. Nucl Instruments Methods. 1975;129:497–499.