Abstract
Background
The purpose of this study was to estimate the additional risk of side effects attributed to internal mammary node irradiation (IMNI) as part of regional lymph node irradiation (RNI) in breast cancer patients and to compare it with estimated overall survival (OS) benefit from IMNI.
Material and methods
Treatment plans (n = 80) with volumetric modulated arc therapy (VMAT) were calculated for 20 patients (4 plans per patient) with left-sided breast cancer from the prospective GATTUM trial in free breathing (FB) and in deep inspiration breath hold (DIBH). We assessed doses to organs at risk ((OARs) lung, contralateral breast and heart) during RNI with and without additional IMNI. Based on the OAR doses, the additional absolute risks of 10-year cardiac mortality, pneumonitis, and secondary lung and breast cancer were estimated using normal tissue complication probability (NTCP) and risk models assuming different age and risk levels.
Results
IMNI notably increased the mean OAR doses. The mean heart dose increased upon IMNI by 0.2–3.4 Gy (median: 1.9 Gy) in FB and 0.0–1.5 Gy (median 0.4 Gy) in DIBH. However, the estimated absolute additional 10-year cardiac mortality caused by IMNI was <0.5% for all patients studied except 70-year-old high risk patients (0.2–2.4% in FB and 0.0–1.1% in DIBH). In comparison to this, the published oncological benefit of IMNI ranges between 3.3% and 4.7%. The estimated additional 10-year risk of secondary cancer of the lung or contralateral breast ranged from 0–1.5% and 0–2.8%, respectively, depending on age and risk levels. IMNI increased the pneumonitis risk in all groups (0–2.2%).
Conclusion
According to our analyses, the published oncological benefit of IMNI outweighs the estimated risk of cardiac mortality even in case of (e.g., cardiac) risk factors during VMAT. The estimated risk of secondary cancer or pneumonitis attributed to IMNI is low. DIBH reduces the estimated additional risk of IMNI even further and should be strongly considered especially in patients with a high baseline risk.
Introduction
Large randomized studies (EORTC 22922/10925, MA.20) demonstrated better regional and distant control rates as well as a better disease-specific survival after regional lymph node irradiation (RNI) in high-risk breast cancer patients [Citation1,Citation2]. In these randomized trials, RNI comprised both the supra-/infraclavicular region and the internal mammary node irradiation (IMNI) as a constant component. Therefore, the additional contribution of IMNI during RNI remains unclear in the randomized EORTC 22922/10925 and MA.20 trials. Yet, several studies have been conducted to separately investigate the role of IMNI during RNI in breast cancer radiation therapy. In prospective nonrandomized trials by Stemmer et al. [Citation3] and the Danish Breast Cancer Group (DBCG) [Citation4], IMNI resulted in a significant improvement of disease-free survival (DFS; by 21% [Citation3]) and overall survival (by 3.7% [Citation4]). This benefit could be confirmed in a recently published study by Luo et al. [Citation5] (DFS +13.4%, OS +4.8%). In the only randomized trial on IMNI (French trial [Citation6]) a non-significant trend toward an improved OS (by 3.3%) was observed after 11.3-year follow-up. Even if this study failed to demonstrate a clear survival benefit of IMNI, a recent network meta-analysis including the French, the EORTC 22922, and the MA.20 trial showed that prolongation in survival due to regional nodal irradiation is only achieved when the internal mammary chain is included [Citation7].
Due to the proximity of the internal mammary chain to the heart and lung, several guidelines advise against IMNI in the case of cardiac risk factors [Citation8,Citation9] and many radiation oncologists are reluctant to use IMNI during RNI [Citation10]. However, reliable clinical evidence for IMNI toxicity is missing, particularly for modern irradiation techniques such as deep inspiration breath-hold (DIBH) with volumetric modulated arc therapy (VMAT), which allows a better sparing of the OARs [Citation11–14]. Notably, a recent metanalysis by the EBCTCG indicates that improvements in the irradiation technique over the years resulted in better outcomes of RNI [Citation15].
Since concerns regarding toxicity remain the limiting factor for the implementation of IMNI in clinical practice, it is crucial to quantify the risk of additional side effects in the OARs and to estimate the pros and cons of different scenarios. The aim of the current study was to compare the absolute risk of side effects in the heart, lung and contralateral breast during RNI with IMNI vs. no IMNI using VMAT and DIBH techniques.
Material and methods
Treatment planning
20 patients with left-sided breast cancer receiving whole-breast irradiation after lumpectomy from the prospective GATTUM trial [Citation16] were included in the present analyses. The GATTUM trial is a monocentric study including patients with left-sided breast cancer. The aim of this study is to detect parameters that predict the advantage of DIBH. The patients’ characteristics are summarized in the supplementary file (1). All patients gave informed consent for treatment and the study was approved by the local ethics committee. For each patient, a planning computed tomography scan (CT) was acquired on a Somatom Emotion 16 scanner (Siemens Healthineers, Erlangen, Germany) in DIBH and free-breathing (FB) using the Real-time Position Management (RPM, Varian, Palo Alto, California, USA). The clinical target volume (CTV) of the breast and the lymph node levels were contoured according to the ESTRO contouring recommendations (the caudal border of the internal mammary region was defined as the cranial side of the 4th rib) [Citation17]. In addition, the OARs including the lungs, the contralateral breast, and the heart were contoured in the treatment planning software (Eclipse, Varian, Palo Alto, CA). For contouring of the heart and its substructures (left anterior descending artery [LAD], right and left ventricle [RV, LV]) the cardiac contouring atlas for radiation therapy by Duane et al. [Citation18] was used. In each CT (DIBH and FB) two clinical target volumes (CTV) were defined:
As comprising the breast and the supra-/infraclavicular region (−IMNI)
As in (1) plus the internal mammary region (+IMNI)
The CTV to PTV margin for the lymph node regions was 5 mm and for the breast 10 mm. These PTVs were used for plan generation in FB and DIBH (four treatment plans per patient). The treatment plans were calculated using the VMAT technique with the TPS Eclipse 15.6 (Varian Medical Systems, Palo Alto, CA). Treatment plans consisted of 3 partial arcs (290°–170°) and the photon beam energy was 6 MV. To compensate for the intrafractional PTV movement, treatment plan optimization was performed adding a ‘virtual bolus’ of 12 mm. The bolus was removed before the final dose calculation. The dose was calculated with the anisotropic analytical algorithm (AAA) and a grid size of 2.5 × 2.5 mm2. No automatization tools were used. Treatment plans were calculated by two different physicists, but the four plans for one patient were always calculated by the same physicist. The prescription dose to the PTV was 50.4 Gy delivered in 1.8 Gy per fraction and the plans were normalized to a median PTV dose corresponding to the prescription dose. In addition to the planning PTV, an ‘evaluation PTV’ was created by excluding any lung tissue and a 5 mm skin layer. This evaluation PTV was used for DVH constraints and analyses: 95% of the evaluation PTV should be covered by the 95% isodose line. The 95% isodose line encompassed the dorsal limit of the PTV (assessed by visual inspection) to avoid dose reduction in the internal mammary region. Dose maximum should not exceed 110% and preferably not 107%. The dose to OARs was kept as low as possible, without compromising the PTV dose. The treatment plans analyzed in this study were calculated solely for scientific purposes and all patients had already completed the treatment prior to initiation of the study. The treatment of the patients was carried out in accordance with the specifications of the GATTUM trial [Citation16].
Dose evaluation and risk models
The aim of risk modeling was to estimate the additional absolute risks due to IMNI for the endpoints of cardiac mortality, radiation pneumonitis, secondary lung cancer and contralateral breast cancer applying NTCP or risk models. For this, we estimated the radiation-induced toxicities assuming different ages (40, 50, 60 or 70 years) and risk factors (high risk and low risk; ) based on the doses in the OARs for the 20 patients. The risk scenarios (high vs. low) were defined separately for each OAR, since identified risk factors vary among the organs, and common risk factors may even affect the organ-specific risks in opposing ways (smoking enhances the risk of radiation-induced cardiac mortality but reduces the risk of radiation pneumonitis). The risk models and calculations used for each endpoint are listed in and explained in detail in the supplementary file. For cardiac mortality, lung and contralateral breast cancer, radiation risk models were applied that were derived from studies on breast cancer patients. Absolute radiation risk was calculated by the product of the 10-year baseline risk and the radiation excess relative risk (ERR). In contrast, absolute 10-year radiation pneumonitis risks are directly evaluated from a study on lung cancer patients [Citation23]. A detailed description can be found in the supplementary file. All these risk estimates used in the present study are based on mean organ doses (Dmean) to the heart, total lung or contralateral breast. However, some risk models published in the literature (cf. last column in ) work with alternative dose metrics such as Dmax (minimum dose in the cubic centimeter with the highest dose), V20 or V5 (volume fractions with doses above 20 Gy or 5 Gy, respectively) or OED (organ equivalent dose derived with a plateau dose-response relationship proposed by Schneider et al. [Citation29]). Therefore, in supplementary material, we report in addition to mean OAR doses also these dose metrics. The dose values were calculated in MATLAB based on dose-volume histograms (DVHs) exported from the treatment planning software. All presented values are median values based on the 20 patients. To estimate the statistical significance of differences between RNI with and without IMNI and FB vs. DIBH, the Wilcoxon signed-rank test was used. This pairwise test is sensitive to the existence of plan differences but independent of the magnitude of this difference. p-Values <.05 were considered statistically significant.
Table 1. Definition of risk groups, overview of the risk models used for calculation of absolute risks attributed to IMNI, and references to approaches based on alternative dose metrics.
Results
Eighty-two-and-half percent of the treatment plans fulfilled all dose prescription criteria. In 14 treatment plans (8 with and 6 without IMNI) small deviation from V95% were tolerated in the PTV (median V95%: 94.3% (92–94.9%)). The dose distribution of an exemplary patient in FB ± IMNI and DIBH ± IMNI is delineated in .
Figure 1. Dose distribution of an exemplary patient in free breathing without (A) and with (C) IMNI and in DIBH without (B) and with (D) IMNI. Level: sternocostal joint of the third rip.
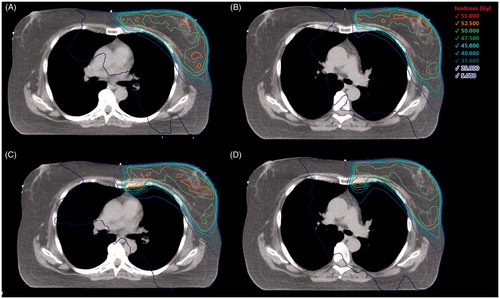
Cardiac dose and cardiac mortality
RNI with IMNI (+IMNI) led to a significant increase of the dose to the heart and heart substructures compared to RNI without IMNI (−IMNI). In free-breathing, the heart Dmean (median over the 20 patients) increased by 33.3% (5.4 vs. 3.6 Gy) and the (median) LAD Dmean by 40.0% (14.5 vs. 8.7 Gy). For the left ventricle, only small differences were observed. In DIBH, the additional doses caused by IMNI were smaller compared to FB (p < .001). However, IMNI still resulted in significantly higher doses to the heart. The (median) heart Dmean increased by 18.1% (p < .001) and the (median) LAD Dmean by 15.9% (p = .09). In 13 out of 20 patients, the (median) dose in DIBH including IMNI was still lower compared to FB without IMNI (ΔDmean20 = −0.5 Gy (−1.5 to −1.4 Gy)) The dose distribution in the heart and heart substructures is summarized in and the supplementary file (2).
Figure 2. Box-plot diagrams delineating the dose difference between DIBH and FB ± IMNI. Comparison of dose to the heart (a), total lung (c, d) and the contralateral breast (b) during RNI with (+) and without (−) IMNI in free breathing (FB) and deep inspiration breath hold (DIBH). (a–c) Mean dose in gray (Gy); (d) volume fraction receiving at least 20 Gy in % (V20Gy). First and second box: positive dose difference means that OAR dose is lower for − IMNI. Third and fourth box: negative dose difference means that OAR dose is lower in DIBH.
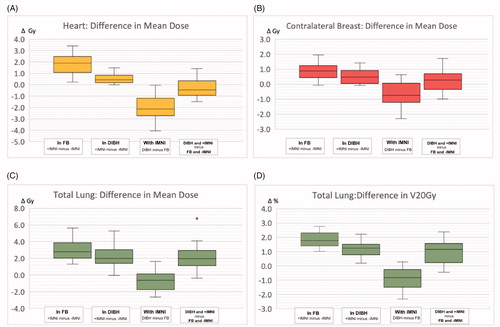
The estimated absolute 10-year cardiac mortality that resulted from the additional doses during IMNI for different age levels (40 years and 70 years) and risk levels are delineated in . The estimated absolute risk for all age levels is summarized in . The estimated additional absolute risk due to IMNI was for all assumed scenarios smaller than 0.3% (both in DIBH and in FB), except for older (70 years) high-risk patients. However, even for a 70-year-old high-risk patient, the estimated additional 10-year cardiac mortality (0.2–2.5%) was clearly lower than the published 8- or 10-year OS-benefit of IMNI (ranging between 3.3% [Citation6] and 4.8% [Citation4,Citation5]). Patients undergoing DIBH and IMNI had a slightly lower risk attributed to radiation therapy compared to patients undergoing FB without IMNI ().
Figure 3. Box-plot diagrams delineating the estimated additional risk of IMNI Estimated 10-year increase of cardiac mortality attributed to IMNI for different age and risk levels compared to the published (8-year or 10 year) overall survival (OS) benefit of IMNI [Citation4,Citation6]. Estimated increase of absolute 10-year contralateral breast cancer risk (b) additional 10-year absolute risks for pneumonitis (c) or secondary lung cancer (d) due to IMNI for different age and risk levels. Baseline risks in parentheses.
![Figure 3. Box-plot diagrams delineating the estimated additional risk of IMNI Estimated 10-year increase of cardiac mortality attributed to IMNI for different age and risk levels compared to the published (8-year or 10 year) overall survival (OS) benefit of IMNI [Citation4,Citation6]. Estimated increase of absolute 10-year contralateral breast cancer risk (b) additional 10-year absolute risks for pneumonitis (c) or secondary lung cancer (d) due to IMNI for different age and risk levels. Baseline risks in parentheses.](/cms/asset/c1ea2dac-4b56-4f5a-8f39-bc70302c7bb1/ionc_a_1787509_f0003_c.jpg)
Table 2. Estimated toxicity after RNI ± IMNI.
Lung dose and toxicity
The mean dose and the V20 of the lung (ipsilateral and bilateral) increased significantly when IMNI was included during RNI. The additional doses in the OARs attributed to IMNI were smaller for DIBH compared to FB, but still significant. The dose values are summarized in and the supplementary file (3).
Radiation pneumonitis
The additional absolute risk for pneumonitis attributed to IMNI was estimated to be <1.7% for all scenarios when DIBH was applied. In FB, the estimated additional risk ranged between 0.2–2.2% depending on patient anatomies, age and risk factors (). These additional values are small compared to the risk that already results from RNI without IMNI ().
Secondary lung cancer
The estimated additional absolute risk for secondary lung cancer was highly dependent on the baseline risk. For nonsmokers, the additional risk for pulmonary cancer resulting from IMNI was generally smaller than 0.1% (both in FB and DIBH). For high-risk patients (smokers) the estimated additional risk reached up to 1.5% in FB and 1.2% in DIBH. The estimated risk for secondary lung cancer in dependence on age and risk factors is delineated in and .
Secondary contralateral breast cancer
Additional IMNI led to a significant increase of the contralateral (median) breast Dmean (or OED, respectively, cf. supplementary material). The additional (median) Dmean attributed to IMNI was 0.9 Gy (FB) and 0.5 Gy (DIBH), respectively (). The additional estimated absolute 10-year risk of contralateral breast cancer attributed to IMNI ranged up to 2.8% in dependence on the baseline risk ( and ).
Discussion
According to our data, the estimated additional (absolute) 10-year cardiovascular mortality attributed to IMNI is very low for most patients. Even in patients with a high cardiovascular baseline risk, the estimated 10-year-mortality caused by IMNI is clearly smaller than the published 10-year overall survival benefit of IMNI. With regard to the 10-year risk of secondary cancer (lung and breast) the estimated additional risk also was small for most scenarios. DIBH lowered significantly the estimated additional risk due to IMNI for all endpoints. Dose reduction to the OARs from DIBH outweighed the dose increase to OARs from IMNI in most patients.
Data on the toxicity of IMNI is very limited in the literature. Most of the available data is derived from the randomized trials that investigated the effect of RNI on the oncologic outcome (EORTC 22922–10925, MA.20, French trial) [Citation1,Citation2,Citation6]. In the MA.20 study, RNI included the supra-/infraclavicular and internal mammary region. RNI increased the rates of acute pneumonitis significantly (1.2 vs. 0.2%) [Citation2]. For delayed events, there were significant increases in lymphedema, fibrosis and telangiectasia in the RNI group. No increase in cardiac disease or secondary cancer was found. However, the follow-up time of 62 months of the MA.20 trials is considered insufficient to exclude relevant late toxicity [Citation33]. For the EORTC 22922-10925 trial, toxicity data after 10 years follow-up is available [Citation1]. Pulmonary fibrosis occurred in 4.4% after RNI and 1.7% in the control group. No significant increase in the cardiac disease was observed (6.5 vs. 5.6%, p = .25). Secondary cancers occurred more often after RNI (n = 222 vs. 191). It is unclear to which extent the observed toxicity can be attributed to IMNI because the treatment groups received in both studies IMNI and supra-/infraclavicular irradiation. The French trial [Citation6] on the other hand focused on the effect of additional IMNI und thus allows an estimation of the toxicity attributed to IMNI. Even though no significant differences regarding toxicity were found in the French trial, grade 3–4 late side effects of radiation were more common in the IMNI group (3.1 vs. 2.3%) and also late cardiac events occurred more often in the IMNI group (2.2%, 15/672 vs. 1.7%, 11/662). A major limitation of that study is outdated irradiation techniques. In general, none of the randomized studies mentioned before used 3D-CT, IMRT or VMAT as a standard modality. However, the treatment technique has an important impact on the dose in the organs at risk. Studies by Ranger et al. [Citation11] and Osman et al. [Citation14] compared the dose distribution between VMAT and 3D-CRT during RNI in left-sided breast cancer patients. In both studies, VMAT led to lower mean heart and lung doses compared to 3D-CRT/Wide tangents and provided better target coverage. Nevertheless (wide) tangential 3D-CRT/IMRT might be advantageous in some patients, especially taking low dose exposure of OARs into account [Citation34,Citation35]. Since low doses to the OARs may actually contribute to secondary cancer risk more than implied by linear risk models [Citation18,Citation35], estimating secondary cancer risk by mean OAR doses only is a potential limitation to our study (discussed below).
In a DBCG simulation study by Thorsen et al. [Citation36] using conventional 3D-CT, IMNI resulted in an increase of the mean heart dose by 4.8%. Despite this large difference (compared to Figire 2(a)) risk calculations by the authors showed that the benefits from IMNI outweigh the costs in terms of ischemic heart death. In the DBCG study, it remains unclear whether patients with high-risk features would also benefit from IMNI. This is relevant as several guidelines advise against IMNI in case of ‘cardiac risk factors’ and reliable clinical evidence (in particular for different subgroups) is lacking.
The published oncologic benefit (OS: 3.3–4.8% [Citation3–6]) of (VMAT-) IMNI exceeds the estimated 10-year risk of cardiac mortality, even in patients with high baseline risk. Even though a comparison of the other endpoints (secondary cancer, pneumonitis) with overall survival is difficult, the risk appears to be relatively low given the published OS benefit of IMNI. Notably, the oncologic benefit published in studies focusing on IMNI is higher (and statistically significant) compared to the benefit of RNI (including supra/infra-clavicular irradiation and IMNI) in the randomized MA.20 and EORTC trials. This could indicate that the estimated benefit of the IMNI the nonrandomized trials is too large, which should be considered during the interpretation of our results. However, a recently published meta-analysis including also the EORTC and MA.20 trials concludes that the OS-benefit of RNI relies on IMNI [Citation7]. Furthermore, modern irradiation techniques are likely to improve survival outcomes compared to the randomized trials, which were initiated more than 20 years ago.
Estimating the additional toxicity of IMNI based on radiation risk models is associated with uncertainties. Limitations with regard to the risk models comprise the possibility of non-linear dose-response relationships, inaccuracies of the models used in this study, and statistical uncertainties (See: supplementary file (5) – Details of the risk models). Also, it should be noted that the risk models are based on data from ‘non-VMAT treatment’ with a different dose distribution the OARs, which might impact the toxicity estimations. Despite the limitations to our methodology, the actual 10-year toxicity resulting from IMNI is likely to be lower in clinical practice than estimated in the current study: First of all, it should be noted that the choice of risk models based on mean organ dose is conservative: addition of IMNI yielded a relative increase of mean organ doses higher than the relative increase in dose metrics used for alternative risk models of cardiac mortality or secondary cancer, see supplementary files (2–4), that is, the reported qualitative findings and conclusions are stable with respect to the choice of alternative risk models. In addition, the toxicity resulting from IMNI may be even lower in clinical practice than estimated in the current study, since (for methodological reasons) we prioritized dose coverage of the IMN-CTV instead of sparing of the OARs during treatment. Moreover, some of our calculations are likely to overestimate the actual 10-year risk. For lung cancer, there is consistent evidence that radiation risk arises not earlier than about 5 years after radiation therapy [Citation28], however, this is less obvious for contralateral breast cancer and cardiac mortality. Therefore, we assumed radiation risk to set in immediately after radiation therapy for these endpoints. This implies an overestimation of risk if there is some biological latency between radiation damage and clinical endpoint.
DIBH is a frequently used technique to decrease the heart and lung dose during adjuvant radiation therapy in breast cancer [Citation37]. Previous studies suggest a lower risk of coronary events and secondary lung cancer when breast cancer patients are treated in DIBH [Citation38]. According to Nguyen et al. [Citation39] relative heart sparing benefits of the DIBH technique are retained also when IMN is included in the target volume. Our study confirms this finding and reveals that DIBH lowers the IMNI-related toxicity for all endpoints. The highest absolute 10-year risk reduction (regarding lung and heart toxicity) during DIBH was achieved in older patients with high-risk factors. In clinical practice, DIBH is often offered to younger patients with a longer life expectancy. These patients have a considerable risk of side effects beyond the period of 10 years. This risk is not explicitly addressed in the current study. Instead, we focused on the 10-year risk to allow for a direct comparison of the toxicity risks to the oncologic benefit published in the prospective trials. Furthermore, patients receiving RNI are mostly (nodal positive) high-risk patients with a less favorable outcome. Still, toxicity beyond 10 years may be relevant for some patients. For the most severe endpoints, cardiac mortality, and lung cancer, the risk strongly increases with age. As a result, cumulative risk until age 80 is not very different between a young patient and a patient at age 70, and DIBH should thus be offered to high-risk patients independent of age [Citation40]. Based on our results, even if older (risk) patients are unable to perform DIBH, IMNI in FB should be strongly considered.
In the current study, we focused only on the late effects of organs with proximity to the internal mammary region. It should be noted, that IMNI potentially also affects other endpoints such as esophageal cancer and lymphedema, which are not addressed in the current analyses.
Conclusion
The estimated risk of additional IMNI on cardiac mortality, secondary lung and breast cancer and pneumonitis is low. Even in patients with a high baseline risk, previously published oncologic benefit of IMNI outweighs the additional 10-year cardiac mortality. According to our analysis, IMNI can be safely applied even in patients with risk factors using VMAT-technique. DIBH significantly reduces the additional risk caused by IMNI for all endpoints and should be strongly considered especially for patients with a high baseline risk, irrespective of their age.
Author contributions
Statistical analysis was done by Cristoforo Simonetto, Pavel Kundrát, Markus Eidemüller, and Kai J. Borm.
Supplemental Material
Download MS Word (62.6 KB)Disclosure statement
No potential conflict of interest was reported by the author(s).
References
- Poortmans PM, Collette S, Kirkove C, et al. Internal mammary and medial supraclavicular irradiation in breast cancer. N Engl J Med. 2015;373(4):317–327.
- Whelan TJ, Olivotto IA, Parulekar WR, et al. Regional nodal irradiation in early-stage breast cancer. N Engl J Med. 2015;373(4):307–316.
- Stemmer SM, Rizel S, Hardan I, et al. The role of irradiation of the internal mammary lymph nodes in high-risk stage II to IIIA breast cancer patients after high-dose chemotherapy: a prospective sequential nonrandomized study. J Clin Oncol. 2003;21(14):2713–2718.
- Thorsen LB, Offersen BV, Dano H, et al. DBCG-IMN: a population-based cohort study on the effect of internal mammary node irradiation in early node-positive breast cancer. J Clin Oncol. 2016;34(4):314–320.
- Luo J, Jin K, Chen X, et al. Internal mammary node irradiation (IMNI) improves survival outcome for patients with clinical stage II–III breast cancer after preoperative systemic therapy. Int J Radiat Oncol Biol Phys. 2019;103(4):895–904.
- Hennequin C, Bossard N, Servagi-Vernat S, et al. Ten-year survival results of a randomized trial of irradiation of internal mammary nodes after mastectomy. Int J Radiat Oncol Biol Phys. 2013;86(5):860–866.
- Haussmann J, Budach W, Tamaskovics B, et al. Which target volume should be considered when irradiating the regional nodes in breast cancer? Results of a network-meta-analysis. Radiat Oncol. 2019;14(1):102.
- Recommendations 2020 [Internet]. Copenhagen (Denmark): AWOgyn; Diagnosis and treatment of patients with primary and metastatic breast cancer. 2020 [cited 2020 February]. Available from: www.ago-online.de
- S3-Leitlinie Früherkennung, Diagnose, Therapie und Nachsorge des Mammakarzinoms [Internet]. Berlin (Germany): Deutsche Krebgesellschaft; 2020 [cited 2018 September 15]. Available from: http://leitlinienprogramm-onkologie.de/Mammakarzinom.67.0.html
- Borm KJ, Kessel K, Devecka M, et al. Variability in lymph node irradiation in patients with breast cancer-results from a multi-center survey in German-speaking countries. Strahlenther Onkol. 2020;196(1):15–22.
- Ranger A, Dunlop A, Hutchinson K, et al. A dosimetric comparison of breast radiotherapy techniques to treat locoregional lymph nodes including the internal mammary chain. Clin Oncol (R Coll Radiol). 2018;30(6):346–353.
- Nissen HD, Appelt AL. Improved heart, lung and target dose with deep inspiration breath hold in a large clinical series of breast cancer patients. Radiother Oncol. 2013;106(1):28–32.
- Lawler G, Leech M. Dose sparing potential of deep inspiration breath-hold technique for left breast cancer radiotherapy organs-at-risk. Anticancer Res. 2017;37(2):883–890.
- Osman SO, Hol S, Poortmans PM, et al. Volumetric modulated arc therapy and breath-hold in image-guided locoregional left-sided breast irradiation. Radiother Oncol. 2014;112(1):17–22.
- D Dodwell CT, McGale P, Coles C, et al. Regional lymph node irradiation in early stage breast cancer: an EBCTCG meta-analysis of 13,000 women in 14 trials. Cancer Res. 2019;79(4):GS4-02.
- Duma M. Gated Radiotherapy in Left Sided Breast Cancer Patients (GATTUM). 2018. Available from: https://clinicaltrials.gov/ct2/show/NCT03534570.
- Offersen BV, Boersma LJ, Kirkove C, et al. ESTRO consensus guideline on target volume delineation for elective radiation therapy of early stage breast cancer, version 1.1. Radiother Oncol. 2016;118(1):205–208.
- Duane F, Aznar MC, Bartlett F, et al. A cardiac contouring atlas for radiotherapy. Radiother Oncol. 2017;122(3):416–422.
- Conroy RM, Pyorala K, Fitzgerald AP, et al. Estimation of ten-year risk of fatal cardiovascular disease in Europe: the SCORE project. Eur Heart J. 2003;24(11):987–1003.
- Darby SC, Ewertz M, McGale P, et al. Risk of ischemic heart disease in women after radiotherapy for breast cancer. N Engl J Med. 2013;368(11):987–998.
- van den Bogaard VA, Ta BD, van der Schaaf A, et al. Validation and modification of a prediction model for acute cardiac events in patients with breast cancer treated with radiotherapy based on three-dimensional dose distributions to cardiac substructures. J Clin Oncol. 2017;35(11):1171–1178.
- Semenenko VA, Li XA. Lyman-Kutcher-Burman NTCP model parameters for radiation pneumonitis and xerostomia based on combined analysis of published clinical data. Phys Med Biol. 2008;53(3):737–755.
- Appelt AL, Vogelius IR, Farr KP, et al. Towards individualized dose constraints: adjusting the QUANTEC radiation pneumonitis model for clinical risk factors. Acta Oncol. 2014;53(5):605–612.
- Marks LB, Bentzen SM, Deasy JO, et al. Radiation dose-volume effects in the lung. Int J Radiat Oncol Biol Phys. 2010;76(3):S70–S76.
- Bach PB, Kattan MW, Thornquist MD, et al. Variations in lung cancer risk among smokers. J Natl Cancer Inst. 2003;95(6):470–478.
- Thun MJ, Henley SJ, Burns D, et al. Lung cancer death rates in lifelong nonsmokers. J Natl Cancer Inst. 2006;98(10):691–699.
- Clement-Duchene C, Stock S, Xu X, et al. Survival among never-smokers with lung cancer in the Cancer Care Outcomes Research and Surveillance Study. Annals Ats. 2016;13(1):58–66.
- Taylor C, Correa C, Duane FK, et al. Estimating the risks of breast cancer radiotherapy: evidence from modern radiation doses to the lungs and heart and from previous randomized trials. JCO. 2017;35(15):1641–1649.
- Schneider U, Zwahlen D, Ross D, et al. Estimation of radiation-induced cancer from three-dimensional dose distributions: concept of organ equivalent dose. Int J Radiat Oncol Biol Phys. 2005;61(5):1510–1515.
- Chowdhury M, Euhus D, Arun B, et al. Validation of a personalized risk prediction model for contralateral breast cancer. Breast Cancer Res Treat. 2018;170(2):415–423.
- Stovall M, Smith SA, Langholz BM, et al. Dose to the contralateral breast from radiotherapy and risk of second primary breast cancer in the WECARE study. Int J Radiat Oncol Biol Phys. 2008;72(4):1021–1030.
- Boice JD, Jr., Harvey EB, Blettner M, et al. Cancer in the contralateral breast after radiotherapy for breast cancer. N Engl J Med. 1992;326(12):781–785.
- Budach W, Kammers K, Boelke E, et al. Adjuvant radiotherapy of regional lymph nodes in breast cancer - a meta-analysis of randomized trials. Radiat Oncol. 2013;8:267.
- Karpf D, Sakka M, Metzger M, et al. Left breast irradiation with tangential intensity modulated radiotherapy (t-IMRT) versus tangential volumetric modulated arc therapy (t-VMAT): trade-offs between secondary cancer induction risk and optimal target coverage. Radiat Oncol. 2019;14(1):156.
- Stranzl H, Zurl B, Langsenlehner T, et al. Wide tangential fields including the internal mammary lymph nodes in patients with left-sided breast cancer. Influence of respiratory-controlled radiotherapy (4D-CT) on cardiac exposure. Strahlenther Onkol. 2009;185(3):155–160.
- Thorsen LB, Thomsen MS, Berg M, et al. CT-planned internal mammary node radiotherapy in the DBCG-IMN study: benefit versus potentially harmful effects. Acta Oncol. 2014;53(8):1027–1034.
- Remouchamps VM, Vicini FA, Sharpe MB, et al. Significant reductions in heart and lung doses using deep inspiration breath hold with active breathing control and intensity-modulated radiation therapy for patients treated with locoregional breast irradiation. Int J Radiat Oncol Biol Phys. 2003;55(2):392–406.
- Corradini S, Ballhausen H, Weingandt H, et al. Left-sided breast cancer and risks of secondary lung cancer and ischemic heart disease: effects of modern radiotherapy techniques. Strahlenther Onkol. 2018;194(3):196–205.
- Nguyen MH, Lavilla M, Kim JN, et al. Cardiac sparing characteristics of internal mammary chain radiotherapy using deep inspiration breath hold for left-sided breast cancer. Radiat Oncol. 2018;13(1):103.
- Simonetto C, Eidemuller M, Gaasch A, et al. Does deep inspiration breath-hold prolong life? Individual risk estimates of ischaemic heart disease after breast cancer radiotherapy. Radiother Oncol. 2019;131:202–207.